Abstract
Non-invasive diagnostic tests (Table 3.1) are undertaken to support the clinical impression of and to quantify the extent of cardiac disease. Repeating the investigations over time allows for monitoring of management protocols and follow-up on disease progression. Information may be supplemented with advanced and invasive investigations (e.g. angiography in suspected coronary artery disease (CAD)).
Non-Invasive Diagnostic Tests
Non-invasive diagnostic tests (Table 3.1) are undertaken to support the clinical impression of and to quantify the extent of cardiac disease. Repeating the investigations over time allows for monitoring of management protocols and follow-up on disease progression. Information may be supplemented with advanced and invasive investigations (e.g. angiography in suspected coronary artery disease (CAD)).
Table 3.1 Classification of non-invasive diagnostic techniques
Electrocardiography | Ionizing radiation | Non-ionizing imaging |
---|---|---|
Resting 12-lead ECG Exercise stress test Ambulatory ECG monitoring Intraoperative ECG monitoring Intraoperative ST-segment analysis | CXR CT Nuclear scintigraphy | Echocardiography MRI |
Electrocardiography
The ECG represents the sum of myocardial voltage changes throughout the cardiac cycle along the vector of each of the leads recorded. It may be recorded from the skin surface, from the endocardium in the catheter laboratory or from the epicardium during certain open procedures requiring cardiac mapping. The simplicity of acquisition of the surface ECG makes it one of the most frequent tests performed.
The following processes affect the sum of myocardial voltage changes (Table 3.2):
The frequency of atrial and ventricular systole
The mass of the chambers undergoing depolarization
The route by which depolarization occurs
Myocardial perfusion
Metabolic influences
The ECG, in conjunction with markers of myocardial necrosis (e.g. troponin I), helps to establish the diagnosis of ST-elevation myocardial infarction (STEMI) or non-ST-elevation myocardial infarction (NSTEMI).
Table 3.2 Overview of ECG abnormalities
Abnormality | Description | Comments | |
---|---|---|---|
Atrioventricular conduction | First-degree block | PR interval >200 ms One P-wave per QRS | Seen in CAD, acute rheumatic fever, digoxin toxicity and electrolyte disturbance |
Second-degree block | Mobitz type I Progressive lengthening of PR interval | Also known as Wenkebach Usually benign | |
Mobitz type II PR interval constant, occasional non- conducted beats | May herald complete heart block | ||
2:1 block: two P-waves per QRS complex, normal P-wave rate | May herald complete heart block | ||
Third-degree block | Normal atrial depolarization, no conducted beats, usually wide QRS with ventricular rate <50 per minute | Ventricles excited by slow ‘escape’ mechanism. MI, chronic fibrosis around bundle of His and in RBBB. Consider pacing | |
Intraventricular conduction | LBBB | QRS >120 ms, late R-waves in I, aVL and V5–6, no septal Q-waves, deep S in V1, tall R in V6, associated with T-wave inversion in lateral leads | Best seen in V6 Always pathological, prevents further interpretation of ECG |
Left anterior hemiblock | QRS 100 ms, marked left-axis deviation, deep S in II, III, q in I ± aVL | ||
Left posterior hemiblock | QRS 100 ms, right-axis deviation | ||
RBBB | QRS >120 ms, RSR in V1–2, dominant R and inverted T-wave in V1, usually normal axis, | Best seen in V1 May indicate RV problems, may be normal variant Bifasicular block: RBBB with left anterior hemiblock | |
Ischaemia | ST depression >2 mm | ||
Infarction | Raised ST segments, ± Q-waves (>3 mm, >30 ms), normalization of ST segments, T-wave inversion | ||
Hypertrophy | RA | Peaked P-wave | |
LA | Bifid P-wave | ||
RV | Right axis deviation, tall R in V1, T-wave inversion V1–2, deep S in V6, ± RBBB | ||
LV | R in V5–6 >25 mm or R in V5–6 + S in V1–2 >35 mm |
Exercise ECG
Myocardial oxygen extraction is maximal at rest, so an exercise-induced increase in metabolic demand can only be met by increase in coronary blood flow. It follows therefore that coronary flow limitation in the setting of coronary artery stenosis has a far greater effect during exercise than at rest; thereby increasing the sensitivity of the ECG to detect ischaemia.
Temporal changes in the ST segment may be missed with a single recording
Small non-Q-wave infarcts may fail to meet diagnostic criteria
Large transmural infarcts may obscure additional electrocardiographic events
The sensitivity to detect ischaemia is limited by the position of the exploring electrode
Posterior cardiac events are often missed
Resting ECG may be normal even in the setting of three-vessel CAD
May fail to reflect the effect of exercise on myocardial perfusion
ECG changes lag behind changes in diastolic and systolic dysfunction with ischaemia
Prone to skeletal muscle myopotentials, obscuring changes
Exercise ECG (EECG) testing, using a treadmill or static cycle ergometer, is to investigate ‘cardiac-type’ chest pain or exertional breathlessness. Both treadmill and cycle-based testing are limited to patients with the ability to exercise. In the Bruce protocol both the speed and gradient of the treadmill are increased at each stage, whereas in the modified Bruce protocol the treadmill speed remains constant during the first three stages. In the Naughton protocol, however, only the treadmill gradient is altered. HR and BP are recorded at each increase in workload and the ECG examined for evidence of ST-segment depression. Myocardial ischaemia is suggested by the development of chest pain, ST-segment changes, failure to increase the BP or arrhythmias. Results are defined as positive, negative, equivocal and uninterpretable.
EECG testing is relatively cheap and can be performed in the outpatient setting. It has low specificity and sensitivity (65–70%) for CAD, although simultaneous TTE detection of regional wall motion abnormalities (RWMAs) improves specificity. Low-grade stenoses (<50%) are difficult to detect as are fixed stenoses with collateral blood flow. Contraindications to EECG included: ACS, severe congestive cardiac failure and severe AS. It may also be unsuitable for patients with physical disability, respiratory disease, peripheral vascular disease, LBBB, or atrioventricular (A-V) conduction abnormalities.
Ambulatory ECG
Ambulatory ECG monitoring, also known as Holter or continuous 24-hour recording, is a method used to aid the diagnosis of chest pain, palpitations or syncope that occur intermittently during normal daily activities. The ECG electrodes are applied to the chest and attached to a recording device which is carried by the patient for a period of 24–48 hours. Some devices comprise a patient-activated event monitor. Patients are required to complete a log of events to aid analysis and correlate physical activities with contemporaneous ECG changes. The recorder is interrogated to produce a printout or computer analysis of events.
Longer-term implantable ECG recorders are nowadays used to increase event detection rate. They may record data continuously or intermittently. Integral electrodes are placed subcutaneously in the left subclavian position. Over the past decade, a generation of the Reveal® devices has become the most commonly used implantable cardiac monitoring (ICM) systems. Those are capable of up to 3 years of cardiac monitoring, indicated for patients at increased risk of cardiac arrhythmias and/or experiencing transient dysrhythmic symptoms (e.g. dizziness, palpitation, syncope and chest pain). Reveal LINQ® ICM is the latest and smallest (about 1 ml in volume) programmable model that continuously monitors ECG and other physiological parameters (Figure 3.1). It records cardiac information (up to 30 minutes) triggered by either automatic detection of arrhythmias or upon patient activation. The device then communicates wirelessly to a linked secure network that daily transmits data to the clinic (scheduled or by clinician’s request). The device is also MRI conditional (1.5 and 3 Tesla) and both insertion and removal procedures are conducted under local anaesthesia.
Figure 3.1 The Medtronic Reveal® ICM latest models. Recording electrodes at the top in each model
Transthoracic Echocardiography
TTE is relatively quick and straightforward to perform and provides qualitative and quantitative assessment of cardiac structure and function. Although TTE is an extremely useful tool, it does have several limitations. Certain patient factors reduce echogenicity and therefore make image quality poor or unobtainable. These include obesity, pulmonary emphysema, interference from the ribs, an inability to lie in the lateral position and the presence of surgical drains and anterior chest wall dressings. Findings are relatively operator-dependent and technical difficulties may limit both the quality and completeness of an examination. Spatial resolution is restricted to one wavelength (0.3 mm at 5 MHz) and depth resolution to 200 wavelengths (60 mm at 5 MHz). TTE is poor at imaging posterior structures and Doppler can only be used to estimate flow accurately if the angle of incident ultrasound is <20° (see Chapters 32–35).
Stress Echocardiography
Echocardiography can be combined with exercise testing or dobutamine-induced (40–60 mg kg–1 min-1) stress in patients unable to exercise to allow qualitative assessment of ventricular performance with increase in HR. Occasionally, pacing or atropine is required to obtain an adequate HR response. Imaging dynamic changes in LV outflow obstruction in hypertrophic obstructive cardiomyopathy (HOCM) is also possible. Stress echocardiography is more sensitive than stress ECG at detecting ischaemia. The value of the test rests in the visualization of functional changes with increased myocardial demand.
Contrast Echocardiography
Contrast agents can be employed to improve image quality by delineating the border between endocardium and ventricular cavity. Sonographic contrast agents are suspensions of microspheres filled with perfluorocarbon gas, which enhance image resolution by acting as intravascular tracers. The insonated gas bubbles pulsate, with compression occurring at the peak of the ultrasound wave and expansion at the nadir. SonoVue® is a stabilized aqueous suspension of sulphur hexafluoride (2.5 µm microbubbles) within a shell of polyethylene glycol (macrogel 4,000). After peripheral IV injection of 0.2–0.4 ml, the microbubbles traverse the pulmonary vascular bed to opacify the ventricular cavity. Further refinements allow quantification of coronary microcirculation by assessment of subendocardial opacification. This is particularly powerful during stress echocardiography as a reduction in opacification is often easier to appreciate than a new RWMA. Allergic reactions to contrast agents have occurred and therefore contrast is contraindicated in patients with recent unstable cardiac symptoms, a recent (<7 days) coronary intervention, class III and IV heart failure or serious arrhythmias.
Cardiovascular Imaging
Over the years, new cardiovascular radiological imaging modalities have been developed to assess the beating heart. A comprehensive non-invasive cardiac examination is now possible. The anaesthetist must be aware of the indications and limits of each technique.
Chest Radiography
A posteroanterior and lateral CXR provide information about the heart, the lungs, the great vessels and the thoracic skeleton (Box 3.2). A plain preoperative CXR provides a baseline against which to judge postoperative images.
Box 3.2 Assessment of the preoperative chest radiograph before cardiac surgery
Cardiac silhouette
Cardiothoracic ratio (‘normal’ ≤50%)
LA enlargement
Calcification – LV wall, valvular, pericardial
Prostheses/pacing wires
Mediastinal silhouette
Calcification – aortic arch
Mediastinal widening
Tracheal deviation
Hila
Pulmonary arteries and veins
Lymphadenopathy and other masses
Lung fields
Upper lobes blood diversion
Interlobular septal (Kerley B) lines
Perihilar (‘Bats Wing’) opacities
Diaphragm
Pleural effusion
Skeleton
Sternal wires – previous surgery
Rib notching
Retrosternal space – in redo-surgery
Nuclear Cardiology
Scintigraphy, positron emission tomography (PET) and single photon emission computed tomography (SPECT) detect gamma rays emitted by a radionuclide tracer administered to the patient. In contrast to scintigraphy imaging (planar 2D), PET and SPECT can provide information in three dimensions. The radionuclide tracers thallium (201TI), technetium-sestamibi (99mTc-sestamibi) and 99mTc-tetrofosmin are routinely used for SPECT and scintigraphy. Due to its shorter half-life (99mTc, 6 hours versus 201TI, 72 hours), a higher dose of 99mTc can be administered. Superior image quality at a lower radiation dose makes 99mTc the preferred tracer. The radionuclide tracers rubidium (82Rb) and N-ammonia (13N-ammonia) are used for PET.
In contrast to SPECT, PET is now increasingly penetrating clinical cardiac applications with advanced insights into pathophysiology and biology owing to several recent developments. PET current applications allow assessment of myocardial blood flow, myocardial viability and, when ECG-gated imaging is used, global and regional ventricular function. The main limitations of these techniques are relatively poor spatial resolution, soft tissue attenuation artefacts and delivery of a significant dose of ionizing radiation. Recent trends, however, have introduced novel developments into cardiac nuclear imaging, such as dedicated cardiac SPECT cameras (faster, dynamic with lower radiation dose), hybrid nuclear imaging (PET/MRI) and advanced molecular imaging (metabolism and sympathetic innervation).
Stress Imaging
Myocardial imaging at rest and during exercise (stress) permits the assessment of myocardial perfusion (Table 3.3). Pharmacological stress, induced using a vasodilator (adenosine or dipyridamole) or an inotrope (dobutamine), can be used in patients unable to exercise. Multiple or severe inducible ischaemic defects represent significant scintigraphic findings. ECG-gated acquisition gives improved diagnostic accuracy by allowing better distinction between true perfusion abnormalities and artefacts. It also allows evaluation of ventricular function (Figure 3.2).
Table 3.3 Clinical significance of radionuclide tracer uptake during myocardial perfusion
Tracer uptake pattern | Significance |
---|---|
Homogeneous | Normal myocardial perfusion (except when severe three-vessel disease: homogeneous uptake associated with poor LV function) Low risk of cardiac event (0.6% per year) |
Reversible defect | Defect on stress study only Indicates reversible ischaemia/viable myocardium High risk of cardiac event (~7–13% per year) Indication of revascularization therapy |
Fixed defect | Defect on rest and stress study Indicates MI |
Figure 3.2 99mTc-sestamibi myocardial perfusion scintigraphy. Example of a patient with reversible apical and inferobasal perfusion defects (white arrows). Analysis of tracer uptake allows quantification and localization (20 myocardial segments) of the lateral (LAT) and septal (SEPT) lesions.
Furthermore, viable myocardium can be identified either by perfusion (201TI or 99mTc-sestamibi; assessment of cellular membrane integrity) or metabolic (fluorodeoxyglucose (18FDG)) tracers. Myocardial viability is an important determinant of the likely benefits of revascularization therapy. The indications and effectiveness of myocardial perfusion imaging are summarized in Box 3.3 and Table 3.4.
Box 3.3 Indications for myocardial perfusion imaging
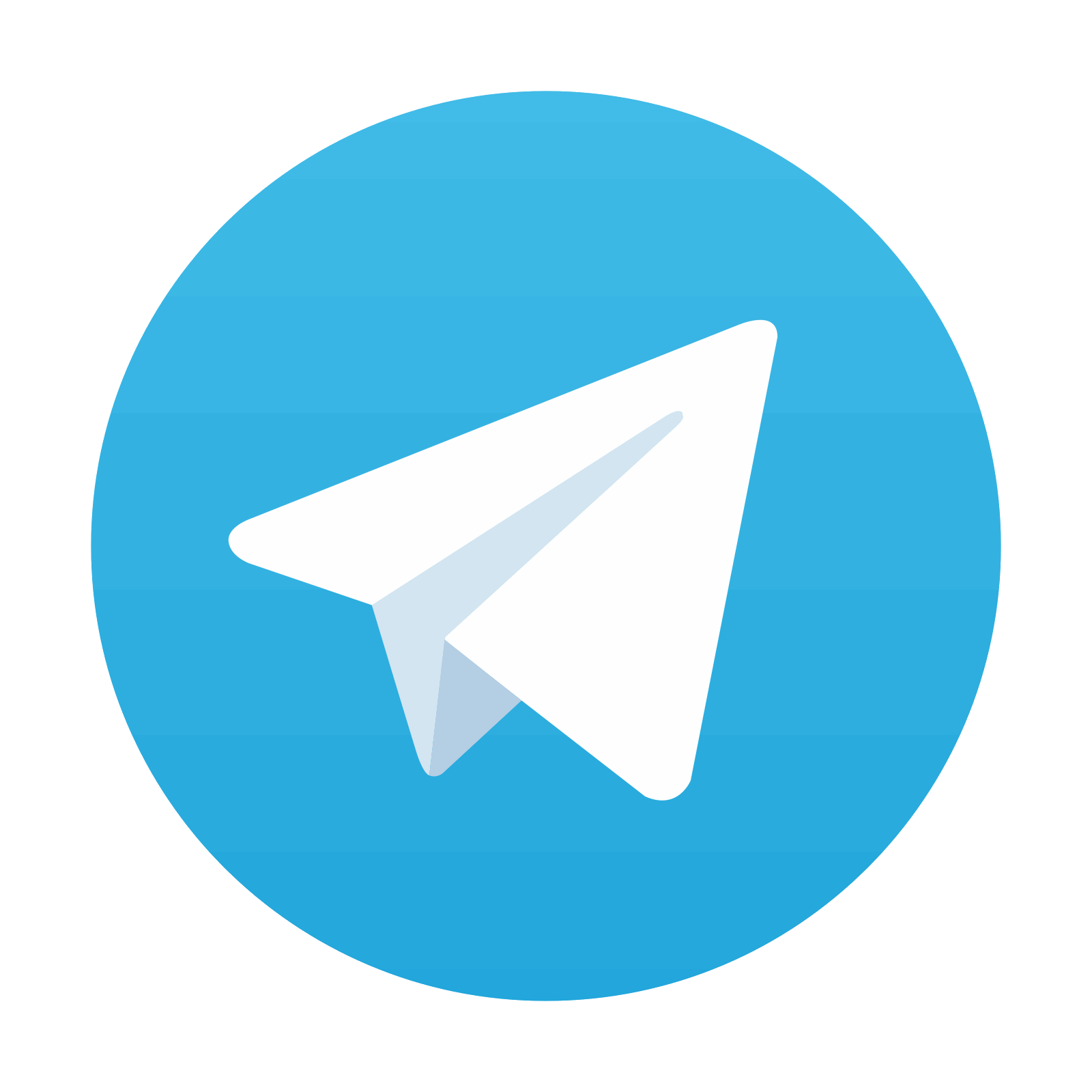
Stay updated, free articles. Join our Telegram channel

Full access? Get Clinical Tree
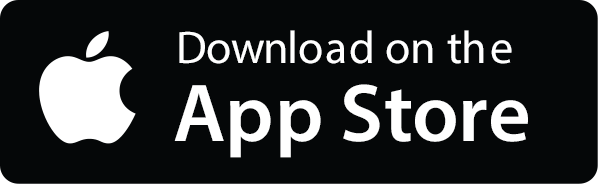
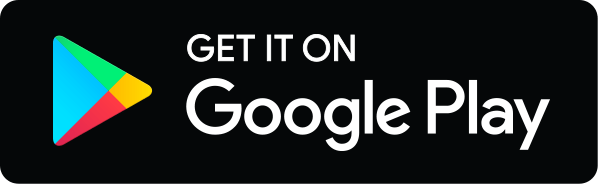
