Cardiopulmonary Arrest
Christopher Ross and Theresa M. Schwab
Sudden cardiopulmonary arrest (SCA) and sudden cardiac death (SCD) typically result due to ventricular dysrhythmias resulting in hemodynamic collapse due to the sudden cessation of cardiac activity. The triad of unconsciousness, apnea, and pulselessness defines cardiopulmonary arrest. Cardiovascular disease causes about a third of all worldwide mortality with half of this caused by SCD (1). SCD is defined as an unexpected natural death from a cardiac cause that occurs instantaneously or within 1 hour from the onset of an abrupt change in clinical status in a person without a prior condition that would appear to be fatal. The term SCD is used to describe cardiac arrest whether or not resuscitation occurs. The term can be misleading because not all affected individuals die. Patients who do not die after cardiac arrest have “aborted SCD.” By convention, the term describes both fatal and nonfatal cardiac arrest (2).
Resuscitation was described more than 100 years ago. It was only in the late 1950s that closed-chest cardiac massage, mouth-to-mouth ventilation, and electrical defibrillation were incorporated into practice guidelines in an attempt to improve survival. Although advances in emergency medical services (EMSs) and in the field of resuscitation have occurred, the rate of survival of cardiac arrest victims who are neurologically intact remains dismally poor (3). Although survival assessments have reached widely disparate conclusions based on study populations in the out-of-hospital setting, survival rates range from 4% to 15% (4). Survival to hospital discharge has been reported to increase in those treated with bystander cardiopulmonary resuscitation (CPR), EMS, and when the underlying rhythm was ventricular fibrillation (VF) (2). In-hospital survival of SCD has been reported to be 22% survival to hospital discharge (5). The concept of extending treatment into the community is a fairly new phenomenon. In the 1970s, it was demonstrated that advanced cardiac life support (ACLS) by trained paramedics could significantly improve resuscitation and survival in patients suffering cardiac arrest. Training laypersons in the community to deliver CPR has been shown to increase survival of SCD. The availability of automatic external defibrillators to trained persons such as police and flight attendants and their use by these people prior to EMS intervention also increases survival (2).
The epidemiology of SCD parallels that of ischemic heart disease with up to 70% of patients with known coronary artery disease (CAD) succumbing to SCD. The incidence increases with age and cardiac risk factors such as smoking, diabetes, left ventricular hypertrophy, and hypercholesterolemia. The biggest risk factor for SCD is previous CAD, especially if there is associated left ventricular dysfunction (6). The more cardiac risk factors and electrocardiogram (ECG) abnormalities present, the higher the risk of SCD.
The effect of race on SCD is controversial as it is difficult to separate socioeconomic influences from a true genetic predisposition. That being said, black patients appear more likely than whites to experience SCD at a younger age and with lower survivability. Black patients are also more likely to have an unwitnessed arrest and have their initial rhythm being a less favorable one for resuscitation (7). Men are three to four times more likely than women to experience SCD. This difference can be explained by the difference in the incidence of CAD and the hormonal protection women have before menopause. Recent major life changes, women who are not married or who have no children or few children, high-stress lifestyle, and type A personality are all factors that increase the risk for SCD, although the validity of some of these claims remains controversial. There may be both seasonal and circadian patterns for risk assessment in SCD because SCD is more likely to occur in the winter months. SCD, like acute myocardial infarction (AMI), is most likely to occur in the first few hours after awakening from sleep as a consequence of increased sympathetic stimulation (7). Modifiable risk factors play a role in risk of SCD. There was a 92% reduction in SCD in female patients who did not smoke, had an appropriate weight, exercised, and ate an appropriate diet (8).
The ECG can identify patients at an increased risk for SCD. ECG findings consistent with previous or current ischemic disease such as old myocardial infarction (MI), left ventricular hypertrophy, interventricular conduction delay, repolarization abnormality, or any current of injury pattern are significant predictors of SCD. Some studies report that a powerful predictor of SCD is three or more premature ventricular contractions (PVCs). Other studies state that high-risk forms of PVCs such as multifocal, bigeminy, short coupling with R-on-T phenomenon, and three or more consecutive ectopic beats are more predictive. There are also specific ECG findings such as interventricular conduction delays, QT prolongation, delta waves, and an increase in resting heart rate of more than 90 beats per minute that place patients at a higher risk.
Multiple factors trigger SCD in a susceptible myocardium, but the final mechanisms for triggering the fatal ventricular dysrhythmias is unclear. Significant coronary stenosis is the most common abnormality found in SCD patients, although more than half of SCD patients have no previous history of CAD. Fewer than half of all SCDs occur during an AMI. In fact, cardiac arrest during AMI is associated with an improved outcome when compared to cases not occurring in this setting. Other factors involved include other structural abnormalities and transient electrophysiologic disturbances (Table 3.1). In the majority of cases, the terminal rhythm of VF has occurred from the initiating event of a ventricular tachydyrhythmia (7).
TABLE 3.1
Risks Associated with Sudden Cardiac Death
Prolongation of the QT interval on the ECG increases the risk of SCD as a consequence of the increased risk of polymorphic ventricular tachycardia (VT). This prolongation predisposes myocardium to re-entry phenomenon. Re-entry is the major mechanism by which a dysrhythmia is created and maintained, although triggered activity and increased automaticity are also important. A minority of cases of SCD begins with a bradydysrhythmia or an organized rhythm without a pulse (pulseless electrical activity [PEA]). VF usually begins with a run of sustained or nonsustained VT that degenerates to VF.
The net result of the dysrhythmia is global cessation of blood flow and corresponding ischemic injury to various organs. Resultant hypoxia and hypotension cause cessation of aerobic metabolism within seconds. Anaerobic metabolism occurs that cannot meet the metabolic demands of the tissues, and subsequent cell death ensues if spontaneous circulation is not resumed. The time before irreversible cell death is dependent on the metabolic rate of the organ system. Both the heart and brain have high metabolic rate and are very sensitive to ischemic insults. Thus the probability of achieving successful resuscitation from cardiac arrest is related to the interval from onset to institution of resuscitative efforts, the setting in which the event occurs, the mechanism (VF, VT, PEA, or asystole), and the clinical status of the patient prior to the cardiac arrest.
Although estimates vary widely, VF and pulseless VT are responsible for 25% to 35% of all out-of-hospital episodes of SCD. PEA accounts for as much as 25%. The acute management of patients with SCD is based upon the underlying electrocardiographic rhythm. During the course of resuscitation, patients may manifest many different rhythms, and therapy is modified based on these changes.
CLINICAL PRESENTATION
Patients suffering from SCD do not have any more specific complaints than those suffering any major cardiac or pulmonary event. Often, symptoms such as increasing angina, dyspnea, palpitations, easy fatigability, and other nonspecific complaints are experienced by these patients in the preceding days to months of the event. However, the onset of the terminal event of SCD, if instantaneous or abrupt, is cardiac in origin in more than 95% of patients. Dysrhythmic events, characterized by a high likelihood of patients being awake and active immediately prior to the event, are dominated by VF as the electrical mechanism, and have a short duration of terminal illness. Patients who have circulatory failure deaths tend to have more systemic medical problems and present with a higher incidence of asystole and PEA. A cardiac arrest may be characterized by symptoms such as prolonged angina or the pain of an MI, acute shortness of breath, palpitations, and lightheadedness. The complete loss of consciousness in this setting is the sine qua non in cardiac arrest. The natural history of cardiac arrest is to progress to death within minutes unless expedient interventions are undertaken.
Phases of Resuscitation
Many researchers now consider there to be three distinct time-sensitive phases of cardiac arrest: electrical, circulatory or hemodynamic, and metabolic. Treatment emphasis varies according to the phase (9).
The electrical phase occurs during the first 4 minutes of arrest caused by VF. This is the period when immediate defibrillation is likely to be successful. As ischemia progresses, the success of attempted defibrillation diminishes without CPR. The hemodynamic or circulatory phase occurs during the period of 4 to 10 minutes after sudden cardiac arrest. This phase is characterized by transition to slow VF wavelets as ischemic metabolites accumulate in the myocardium. The patient may remain in VF. Although early defibrillation has traditionally been advocated for all patients found in VF, there is evidence that patients with time-sensitive phases caused by prolonged pulselessness may benefit from well-performed CPR to generate adequate cerebral and coronary perfusion prior to the initial attempts at defibrillation. There is insufficient evidence to determine if the 1½ to 3 minutes of CPR should be provided prior to defibrillation (10). CPR should be performed while a defibrillator is being readied for use. Treatment of the metabolic phase, which is defined as occurring after >10 minutes of pulselessness, stresses postresuscitation measures including hypothermia therapy. In the absence of conversion into a perfusing rhythm, patients who have reached this phase generally do not survive.
ED EVALUATION AND MANAGEMENT
Many of the recommendations stated in this chapter are taken from the guidelines for CPR introduced in 2010 by the American Heart Association. The new CPR guidelines evolved from emerging evidence-based resuscitation studies (Table 3.2). Based on their extensive review, the AHA and European Resuscitation Council (ERC), in collaboration with the International Liaison Committee on Resuscitation (ILCOR), published updated guidelines for BLS and ACLS.
TABLE 3.2
Levels of Evidence for Interventions
Return of spontaneous circulations (ROSCs) is dependent on restoration of adequate cardiac function. Restoration of normal brain function is the defining factor of a successful resuscitation. The likelihood of attainment of both of these goals decreases with each passing minute of the cardiac arrest. Healthcare providers need to orchestrate simultaneous assessment and management of a cardiac arrest victim.
Patients require airway and ventilatory support, control of cardiac dysrhythmias, and cardiac output stabilized to restore organ perfusion. Emphasis is on immediate, uninterrupted, high-quality CPR and early defibrillation. These procedures along with advanced life support measures such as endotracheal intubation, pacing, pressor agents, and insertion of an intravenous (IV) line carry out these goals in resuscitation. The final goal is to restore ROSC before irreversible neurologic damage occurs. If the patient is resuscitated, rapid diagnosis and proper management of the pathologic condition that precipitated and resulted from the arrest are essential for optimal outcome. Treatment with mild hypothermia also improves cerebral resuscitation in these patients.
Basic Cardiopulmonary Resuscitation
Americans spend about a billion dollars a year on some form of CPR training or retraining. Yet the utility of this training is limited in the field because of the poor outcome of community cardiac arrests. CPR alone is of little value for restoring effective circulation and preventing brain death. The survival from SCD is dependent on a series of actions represented by the AHA Chain of Survival: early access to 911, early CPR, early defibrillation, and early advanced care.
An algorithmic approach to the unresponsive victim is used. Once a victim is found unresponsive, activation of the EMS system should occur. The activation of the EMS system to allow rapid initiation of ACLS protocols is the first step in the graduated response to care. If the lay rescuer finds an unresponsive victim who is breathing abnormally or not at all, CPR should be initiated. Trained healthcare providers should take no longer than 10 seconds to check a pulse before CPR is initiated. For lay person/untrained personnel, chest compression only CPR (no intervening breaths) is recommended (11). For trained personnel, compressions should be performed prior to rescue breaths in a ratio of 30:2 with a rate of 100 compressions per minute. The patient’s airway can then be opened, followed by two breaths that should make the chest rise. Healthcare providers can then check for a pulse and, if it is absent, can initiate CPR in cycles of 30 compressions to two breaths. The function of basic CPR is to provide some blood flow to vital organs until more definitive treatment, such as defibrillation, can be initiated. Once a defibrillator arrives, the electrical rhythm of the heart can be ascertained, and, if a shockable rhythm is present, defibrillation can occur.
Most studies show a benefit of layperson CPR in the resuscitation of victims of cardiac arrest, especially when initiated early. “Hands only” CPR is a potentially lifesaving technique involving no mouth-to-mouth contact and has been the focus of the American Heart Association media blitz to educate the public that patients who get immediate CPR have twice the survival of those who do not. The earlier the CPR is applied the better the outcome becomes. Cardiac output generated by standard chest compressions is <10% to 30% of baseline and decreases precipitously with both time to initiation and duration of chest compressions. Endogenous mechanisms direct blood flow preferentially to the heart and brain during the early minutes of resuscitation. This distribution of blood flow is ideal during CPR because the goal is to generate myocardial perfusion adequate to restore organized electrical activity and effective mechanical function of the heart while minimizing ischemic brain injury. This response becomes ineffective within minutes of circulatory arrest; so early intervention is the key to survival.
The mechanism of blood flow during closed-chest compressions has been continuously debated. The major theories include (a) the direct cardiac compression model in which CPR squeezes the heart between the sternum and the thoracic spine, creating a pressure gradient between the ventricles and the great arteries and (b) the thoracic pump mechanism in which the thorax acts as a pump when the chest is compressed, generating pressure gradients between intrathoracic and extrathoracic structures. In either method, the valve system in the heart allows forward, rather than retrograde, blood flow (12) (See Figure 3.1. The boxes bordered with dotted lines indicate actions or steps performed by the healthcare provider but not the lay rescuer.)
FIGURE 3.1 Adult basic life support healthcare provider algorithm. (From Berg RA, Hemphill R, Abella BS, et al. Part 5: Adult basic life support: 2010 American Heart Association Guidelines for Cardiopulmonary Resuscitation and Emergency Cardiovascular Care. Circulation. 2010;122:
S685–S705.)
The mechanism used to stimulate blood flow back to the heart is controversial. It was recently recognized that a small vacuum occurs in the thorax after each chest compression. New devices have been developed to increase this vacuum effect, such as the vest approach, active compression–decompression, and an impedance threshold valve. Using these adjuncts for resuscitation in this manner has been tried, but clinical results have proven disappointing for many reasons, including an inability to implement the mechanism and an inability to overcome the standard CPR mindset. Thus, CPR has changed very little in the past 20 years.
CPR has now taken a more central role in the provision of BLS. The current focus is on high-quality CPR. Poor performance of CPR is one factor contributing to poor outcomes. Multiple studies assessing both in-hospital and prehospital CPR performance have shown that trained healthcare providers consistently fail to meet BLS guidelines. Outcomes from sudden death are dependent on the effectiveness of chest compression, and chest compressions are thought to be the most important element of CPR. When performed at a rate of 100 compressions per minute, with a downstroke of at least 38 mm and allowing the chest to recoil fully between each downstroke, coronary perfusion pressures are optimized, and ROSC is maximized. Most importantly, interruptions in compressions, regardless of how brief, result in a decline in coronary perfusion pressure and result in worse outcomes (13). Clinicians must strive to minimize interruptions of compressions, because interruptions that exceed 15 seconds appear to significantly reduce the success of resuscitation, increase myocardial dysfunction, and significantly reduce survival. The new guidelines mandate that for routine resuscitation, only a single shock, rather than a sequence of three shocks, be delivered so that there is minimal interruption of compressions. Another mandate is to immediately resume chest compressions after defibrillation, decreasing the delay that occurs for a visual confirmation of rhythm. If there is a delay in recognizing that a perfusing rhythm has occurred, continuing chest compressions is not likely to be damaging. The 2010 ACLS protocols stress initiating advanced procedures (advance airway, medication, and reassessments) in a way that does not interrupt CPR (14).
The precordial thump was originally advocated for use in a witnessed cardiac arrest scenario. A forceful precordial thump can convert patients from VF or pulseless VT into a perfusing rhythm. However, patients can also be converted from coordinated cardiac activity to VF, pulseless VT, or asystole. There is little recent literature in the form of peer-reviewed studies, but there are numerous case reports of both benefit and risk (15). The precordial thump should not be used for unwitnessed out-of-hospital cardiac arrest. The precordial thumb may be considered for patients with witnessed, monitored, unstable VT including pulseless VT if a defibrillator is not immediately available, but this should never delay CPR and shock delivery (13).
Airway
After assessing a cardiac arrest victim’s unresponsiveness and activating the response system (in the community, the EMS system and the code team in a hospital are activated), the next step is airway management if there is no immediate access to a defibrillator. In a cardiac arrest victim, the most common reason for airway obstruction is from the tongue falling back in the oropharynx. The airway can be opened by performing either a jaw-thrust or head-tilt–chin-lift maneuver (see Chapter 2). The jaw thrust is the better maneuver if any trauma is suspected or if cervical spine immobilization is required (16).
If the airway is still obstructed after these maneuvers, one must be concerned about an obstructed airway from another cause. The Heimlich maneuver, or subdiaphragmatic abdominal thrust, is the recommended procedure for clearing the obstructed airway of a foreign body. The rescuer’s hand is positioned between the patient’s xiphoid and navel, and several quick thrusts are administered in an attempt to relieve the foreign body obstruction. If the victim is unconscious, like a victim of SCD, the abdominal thrust maneuver may be accompanied by attempts to visualize the foreign body in the pharynx and by finger sweeps to remove the foreign body.
Breathing
The 2010 AHA Guidelines for CPR and Emergency Cardiovascular Care (ECC) recommend the initiation of compressions before ventilations. Blood flow depends on chest compression and delays in, and interruptions of, chest compressions reduce chance of survival. Beginning CPR with 30 compressions rather than two ventilations leads to a shorter delay to first compression. Once chest compressions have been initiated, a trained rescuer should deliver rescue breaths by mouth-to-mouth or bag mask to provide oxygenation and ventilation. The compression to ventilation ratio of 30 chest compressions to 2 ventilations should be followed. During the initial phase of cardiac arrest, the pulmonary vessels and the heart are likely to contain sufficient oxygenated blood to meet markedly reduced demands, and here the importance of compressions supersedes that of ventilations (16). As pulselessness persists, properly performed ventilations become more important, but clinicians must continue to ensure that ventilations do not interfere with the continuity of chest compressions. In the patient with an advanced airway, one asynchronous ventilation (not necessarily coordinated with chest compression) should be given 8 to 10 times per minute and should be delivered in as short a period as possible, while avoiding excessive ventilatory force. Only enough tidal volume should be given to obtain a chest rise. Multiple studies have shown that hyperventilation during resuscitation is routine either through higher rates or increased volumes. Hyperventilation raises intrathoracic pressure, which decreases venous return, pulmonary perfusion, cardiac output, and cerebral and coronary perfusion pressures. This hyperventilation reduces the success of defibrillation and decreases overall survival (16).
Bag mask ventilation is the first choice for BLS ventilation but should give way to alternative airway ventilation methods once ACLS providers are available. Tracheal intubation remains the procedure of choice for the unconscious, apneic patient, although there is no study directly addressing the timing of advanced airway placement during ACLS. This skill is not without significant challenges, and ACLS guidelines recommend intubation be performed by those experienced in the procedure. Physical examination, an end-tidal carbon dioxide detector, or an esophageal detection device can confirm correct tube placement in the trachea. Continuous quantitative waveform capnography is recommended for confirmation and monitoring of endotracheal tube placement, CPR quality, and to aid in the detection of ROSC.
Other airway maneuvers recommended instead of bag valve masking are the laryngeal mask airway or the esophageal–tracheal Combitube (see Chapter 2). Once definitive airway management has occurred, the ventilations are administered independent of the chest compressions at a rate of 8 to 10 per minute.
Circulation
Lay rescuers and healthcare providers have difficulty detecting a pulse in unresponsive patients. Healthcare providers also often take too long trying to feel for a pulse. Lay rescuers should not check for a pulse and healthcare providers should spend no longer than 10 seconds feeling for a pulse. If a pulse check is undertaken, the carotid pulse should be checked because it is the most reliable pulse to check in low-flow states. If no pulse is detected, chest compressions should be instituted. The victim needs to be placed on a firm surface or backboard to assist mechanically with the compressions. The heel of the hand is placed on the lower half of the sternum and depressed 1.5 to 2 in in the adult. In children, the compression depth should be 1 to 1.5 in, and in neonates, it should be 0.5 to 1 in. The recommended compression rate is 100 times per minute in adults (ratio of 30:2 until an advanced airway is placed). Rescuers should push hard and push fast, allow complete chest recoil between compressions, and minimize interruptions in compressions for all victims (13).
Advanced Life Support
Defibrillation
The passage of current through the heart causes defibrillation with the goal of restoring a perfusing cardiac rhythm. If energy and current are too low, the delivered shock will not terminate the dysrhythmia; if energy and current are too high, myocardial damage may result. Because of ischemia, acidosis, and electrophysiologic deterioration, a longer duration of VF correlates with a higher defibrillation threshold (17).
Modern day defibrillators deliver electrical shocks in one of two ways. Monophasic waveforms deliver current in one direction through the heart. Biphasic waveforms are used to deliver current through the heart, reverse polarity, and return through the myocardium. As a result, the energy needed for successful conversion of tachydysrhythmias with biphasic technology is often significantly less than that required with monophasic waveforms. The latest defibrillators and automated external defibrillators (AEDs) use biphasic waveform technology. If a biphasic defibrillator is available, providers should use the manufacturer’s recommended energy dose for terminating VF (usually 120 to 200 J). If the provider is unaware of the effective dose range, the provider may use the maximal dose. Second and subsequent energy levels should be at least equivalent, and higher energy levels may be considered if available (14).
Current is directly related to the energy set on the defibrillator and inversely related to the transthoracic impedance. The transthoracic impedance can be minimized by attention to several factors, including position of the defibrillation paddles, the amount of energy selected on the defibrillator, firm pressure on the paddles, the use of conductive material between the skin and paddles, employment of sets of shocks, and the coordination of the shock with the end-expiratory phase of ventilation.
Defibrillation should be used quickly because delay will reduce the effectiveness of the resuscitation. If VF is corrected within 1 minute, there is a survival rate of >90%; however, the survival rate falls by 10% per minute following the initiating event. In this fashion, public access defibrillation (PAD) has the potential to be one of the greatest advances in the treatment of SCD since the development of CPR. The mechanism for PAD is in the form of an AED. AEDs are computerized devices that are simple and reliable to operate; they allow lay rescuers with minimal training to administer this life-saving intervention. Self-adhesive electrode pads are provided with application diagrams; voice and text prompts guide the rescuer through a few simple steps. A dysrhythmia analysis algorithm automatically interprets the rhythm and either recommends countershock, to be given by the push of a button, or no countershock. After the shock, the device immediately re-evaluates the rhythm and determines whether to recommend an additional shock. Flight attendants, security personnel, sports trainers, police officers, firefighters, lifeguards, family members, and many other trained laypersons have used AEDs successfully in the field.
In the past several years there have been significant changes in our understanding of the physiology of cardiac arrest and the technology used for defibrillation. Biphasic defibrillators, which measure impedance between the electrodes placed on the patient and adjust the energy delivered accordingly, successfully defibrillate approximately 85% of patients with the first shock. The 2010 guidelines restrict the recommended energy delivery to 360 J when using a monophasic defibrillator. With a biphasic defibrillator the suggested energy level is 200 J. The single major change regarding shock delivery in the most recent guidelines is to no longer recommend three-stacked shocks. The single shock should be followed by 2 minutes of CPR with no pulse check. Although there are no randomized controlled trials comparing the one-shock strategy to the three-shock strategy, the evidence for a reduction of coronary perfusion pressure with the interruption of chest compressions was felt to be compelling enough to recommend the one-shock strategy. Early after cardiac arrest, immediate defibrillation is likely successful. The question of intentionally delaying defibrillation to perform CPR is controversial with differing results in out-of-hospital studies. If a defibrillator is immediately available and VF or pulseless VT is evident, a 120-J biphasic shock should be delivered preceding airway management and IV line placement. If VF or pulseless VT is sustained after the initial 120-J shock, higher energies of 200 J are tried later in the resuscitation.
There is a wide variation in the ability of rescuers to determine pulselessness. The AHA 2010 BLS guidelines recommend that CPR performed by the lay rescuer be initiated immediately without a pulse check upon determining unconsciousness in a patient without signs of respiratory effort. Healthcare providers must not spend >10 seconds checking for a pulse and should start CPR immediately if no pulse is felt. Agonal or gasping breaths should not be taken as evidence that organized respiratory effort is present. Further, the guidelines recommend that CPR be resumed for 2 minutes without a pulse check after defibrillation, regardless of the resulting rhythm. Data suggest that after defibrillation the myocardium does not provide effective cardiac output and that CPR may improve postdefibrillation perfusion (14).
Pharmacologic Therapy
Pressor Agents
Historically, catecholamine-induced adrenergic receptor stimulation is the therapy most commonly used in cardiac arrest, with epinephrine being the drug used most often. Epinephrine stimulates α1 and α2 receptors almost equally, and β1 and β2 receptors in a ratio of 1:4. We now realize that trials validating the use of epinephrine in cardiac arrest are not well defined because there are no prospective clinical trials. Although some study results have raised doubts about the benefit of epinephrine, including a large prospective study published in 2012, pending more conclusive data or a formal change in ACLS protocols, epinephrine is still recommended in accordance with ACLS guidelines (18). “High-dose” epinephrine was endorsed in cardiac arrest if regular-dose epinephrine was unsuccessful in resuscitation, only to have the endorsement rescinded by the AHA when larger numbers revealed no benefit and potential harm with such administration. Some studies found that patients may have a higher incidence of ROSC with high-dose epinephrine but no improvement in neurologic outcome. Patients with irreparable central nervous system (CNS) injury may result in significant burden on families and healthcare resources. At present, meta-analyses have not determined if high-dose epinephrine is better, worse, or the same as standard dose. The current recommended dose is epinephrine 1 mg initially intravenously/intraosseously, with repeated doses every 3 to 5 minutes, but the optimal dose has not been established (19).
Vasopressin, also termed antidiuretic hormone, is also part of the treatment armamentarium for the cardiac arrest victim who has VF or pulseless VT. Vasopressin produces the same positive effects as epinephrine in terms of vasoconstriction and increasing the blood flow to the brain and heart during CPR. Vasopressin may not have the adverse effects on the heart that epinephrine does, such as increased ischemia and irritability and, paradoxically, the propensity for VF. Circulating endogenous vasopressin concentrations were high in patients undergoing CPR, and levels in successfully resuscitated patients are significantly higher than in patients who died. Meta-analysis of trials comparing vasopressin with epinephrine for cardiac arrest found no evidence of improved overall outcome or in any subgroup associated with vasopressin therapy (19). Vasopressin is given as a single one-time dose (40 U IV/IO) because of the much longer half-life of 10 to 20 minutes. Vasopressin can be administered as an alternative to the first or second dose of epinephrine.
There is limited experience with vasopressin in PEA or asystole, so epinephrine remains the pressor of choice empirically.
Antidysrhythmic Agents
The role of antidysrhythmic drugs is to suppress premature ectopic complexes and improve symptoms related to palpitations. Most of the antidysrhythmic agents raise the fibrillation threshold and are thus useful for preventing recurrent VF in patients who have been successfully defibrillated.
Amiodarone is categorized as a class III antidysrhythmic but contains characteristics of all four Vaughan Williams classes. Amiodarone can be considered for VF/pulseless VT that is refractory to CPR and vasopressor therapy. In blinded randomized controlled trials in adults with refractory VT/VF in the out-of-hospital setting, paramedic administration of amiodarone (300 mg or 5 mg/kg) improved survival to hospital admission rates when compared with administration of placebo or 1.5 mg/kg or lidocaine (20). This study demonstrated no difference between the amiodarone and placebo groups in hospital discharge of patients with good neurologic functioning, although the study was underpowered for these observations. Additional studies documented consistent improvement in defibrillation response when amiodarone was given to humans or animals with VF or hemodynamically unstable VT. In the ALIVE trial of patients with persistent or recurrent VF despite defibrillation and epinephrine, the number of patients surviving to hospital admission was higher with amiodarone. The ARREST trial in patients with resistant VF/VT again showed increased survival to hospital admission in the amiodarone group. Time to therapy with amiodarone was an independent predictor of survival (21). No study has shown or been powered to show differences in survival to hospital discharge when comparing amiodarone to lidocaine. Amiodarone has been associated with more treatment requiring adverse effects including hypotension and bradycardia when compared to lidocaine but this has been attributed to the vasoactive solvents, as when these products were removed, there was no more hypotension that associated with lidocaine administration (22). The dosage of amiodarone in VF/pulseless VT arrests is 300 mg IV push.
Lidocaine has been used to treat VF/pulseless VT but with little evidence to support its use. The only evidence for lidocaine’s use is from a large historical trial in which receipt of the drug was associated with improved resuscitation and admission alive to hospital. This study had nursing staff present only for the lidocaine cohort, which could explain the difference in survival alone (23). Lidocaine has thus taken an indeterminate role in ACLS and is primarily used if amiodarone is unavailable. The initial recommended dosage of lidocaine is 1 to 1.5 mg/kg IV/IO push. This dose can be followed with 0.5 to 0.75 mg/kg every 5 to 10 minutes for refractory VF to a maximum of 3 mg/kg.
Procainamide is no longer considered an antiarrhythmic option if amiodarone or lidocaine is unsuccessful in breaking VT/VF, given the time required for infusion of an effective dose of procainamide. Procainamide is an effective agent for suppressing ectopic ventricular complexes and VT, as well as other perfusing ventricular dysrhythmias. Procainamide has a limited use in cardiac arrest because of the slow loading to therapeutic doses. Procainamide is administered in an infusion of up to 50 mg/min to a total maximum of 17 mg/kg. The previous use of procainamide in cardiac arrest was supported by only one retrospective comparison study of 20 patients (24).
Magnesium has an unclear mechanism of action in treatment of dysrhythmias. Magnesium’s primary use is in the management of torsades de pointes, although this indication has not had formalized trials. There have been studies that show a correlation between normal magnesium levels and successful resuscitation, but the administration of supplemental magnesium to patients in arrest has not been studied. Three randomized controlled trials have not shown a benefit of magnesium administration in VF arrest in out-of-hospital and in-hospital settings. Its routine use is not recommended in cardiac arrest. The dosage of magnesium is 1 to 2 g IV push in this specific subset of torsades patients.
Guidelines for Cardiac Arrest
The AHA developed guidelines for treatment of patients in cardiac arrest. Every few years the protocols are updated to include the latest in cardiovascular literature, so clinicians should be aware of the most recent version. Although the ACLS treatment for patients in cardiac arrest is based on the ECG monitor rhythm, the clinician must always consider the etiology of the arrest in developing a treatment plan. Cardiac arrest is an endpoint of a physiologic process that results in the loss of circulation and ventilation. Treatable causes of cardiac arrest should always be thought of during resuscitation (Table 3.3).
TABLE 3.3
Most Frequent Causes of Asystole and Pulseless Electrical Activity
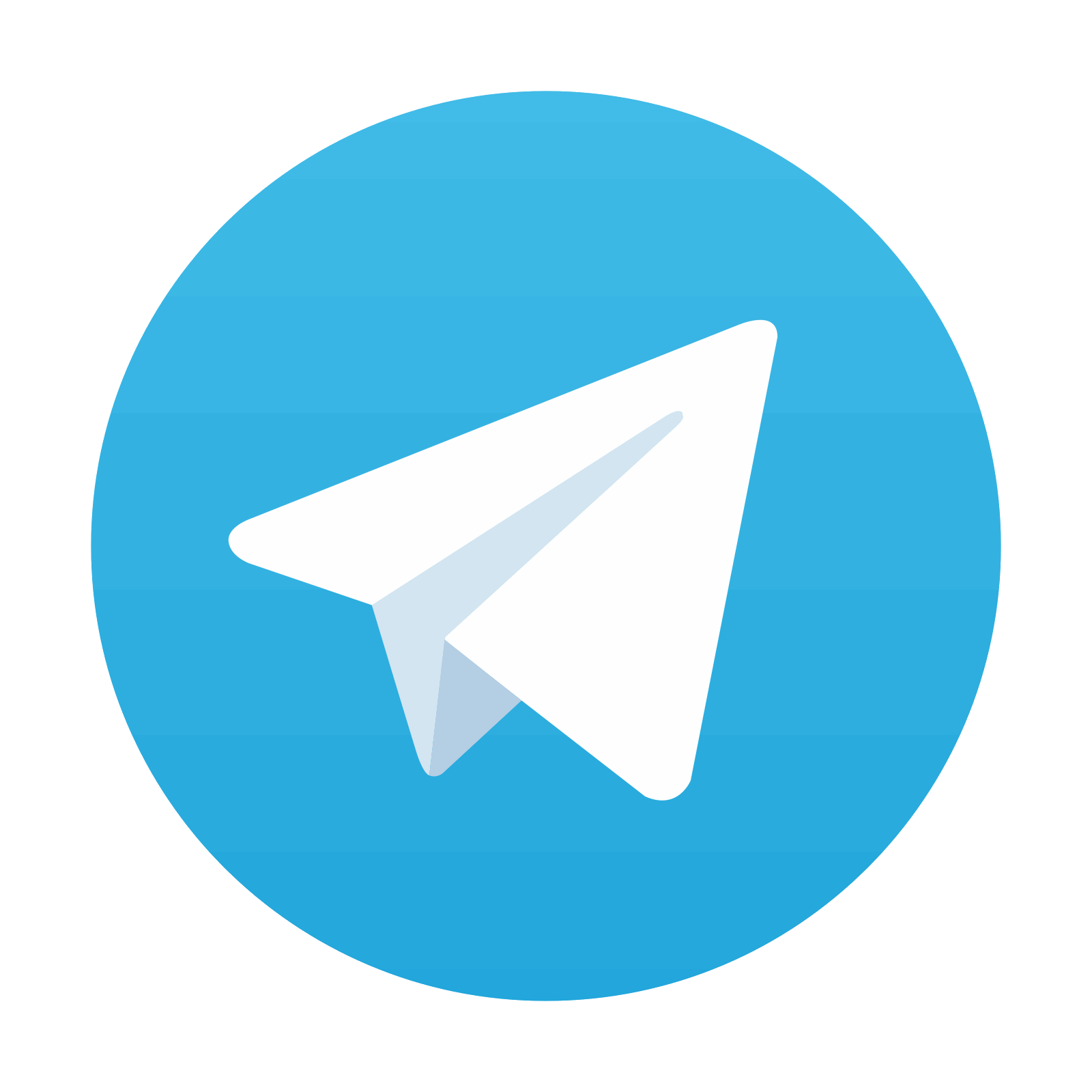