70 Burns and Inhalation Injury
Inhalation injury often occurs in combination with thermal injury and leads to serious complications that manifest at different points in the disease process. Inhalation injury alone carries a 5% to 8% risk of mortality; when combined with burn injury, mortality from inhalational injury can increase to 20% or more.1 These factors, combined with a complicated pathologic course, make inhalation injury a potentially difficult and dangerous disease process.
Classification of Injury
Classifications of inhalation injury have been developed according to several different schemes. One of the first schemes was developed as a result of observations made at the Cocoanut Grove fire of 1942 and grouped patients according to outcomes and their initial symptoms. Early signs of hypoxia that were directly attributable to respiratory tract injury had the highest and most immediate mortality. Signs of cyanosis and dyspnea that occurred within a few hours of the insult could be due to development of pulmonary edema. At 24 hours, upper airway edema was found to be increased and necessitated establishment of an airway (via intubation through a tracheostomy). After 48 hours, patients developed worsening respiratory symptoms due to atelectasis and subsequent pneumonia.2
Additional classification symptoms for inhalation injury were based on the anatomic location of injury. Upper airway injury involves the nasopharyngeal and oropharyngeal regions to the larynx. This damage results in massive edema and compromise of airway patency, often necessitating intubation or tracheostomy. Injury to distal parts of the tracheobronchial tree manifests at a later time after the inhalational injury. Tracheal and major bronchi injuries result in direct mucosal damage and desquamation of the epithelial lining. Injury to the distal alveoli results in atelectasis and predisposes to pneumonia.3
Initial Inhalation Insult
The initial manifestations of inhalation injury are due to direct damage to airway surfaces that result in inflammation and edema.4 Clinically, patients initially present with symptoms of stridor, hypoxia, and respiratory distress.5 Management of the initial insult incorporates a thorough physical examination as well as a careful and specific history that provides details about the extent of exposure to the inhaled substance and the nature of the substance itself. Physical examination should include inspection of the oropharynx for direct damage and documentation of stridor, cyanosis, and confusion; it is not unusual for there to be no obvious physical symptoms of inhalation injury at the time of original assessment. Initial management includes providing adequate oxygenation as well reevaluation and maintenance of airway patency.6
Environmental Variables That Determine Severity
The extent of inhalation injury is related to the duration of exposure and severity of trauma to the tracheobronchial tree. A major component of the degree of the initial inhalation energy is the amount of heat-carrying capacity of the inhaled substance. For example, dry heat has a low heat-carrying capacity and thus usually injures upper airway and supraglottic structures; steam has 4000 times the heat-carrying capacity of dry heat and tends to cause more extensive tracheobronchial damage.7 Thermal injury produces direct injury to the mucosa of upper airway structures, manifesting clinically as upper airway edema within the first 24 hours.3
The level of injury produced by inhalation of particulate matter also depends on the diameter of the inhaled matter. Large-diameter particles (>100 µm) enter the airway but usually do not travel beyond the upper respiratory tract. Smaller particles (<10 µm) can reach the lower tracheobronchial tree, while even smaller particles (<5 µm) can reach the terminal bronchus and alveolus. Particulate matter can cause direct mechanical damage and can also transport toxins beyond the level of the initial inhalation.3
Pathology
Upper Airway Injury
Upper airway structures that are in direct danger from inhalation injury include the mucous membranes of the nasopharynx, hypopharynx, epiglottis, glottis, and larynx, which can demonstrate a significant amount of inflammation due to direct injury. In addition, the cartilage of the glottis is intolerant of edema, and damage to this structure can produce life-threatening compromise of airway patency.7
Injury to the upper airway from direct thermal injury occurs very early and quickly manifests symptoms. Mucous membranes are damaged when the temperature of inhaled gases reaches 150°C. The resulting damage initiates an inflammatory cascade that leads to increased capillary permeability, histamine release, and inflow of transudative fluid, all of which result in edema. This process starts over the course of the first 24 hours post exposure, and the resultant edema typically resolves in 4 to 5 days. Airway compromise occurs when edema causes the airway diameter to fall below 8 mm and thus mandates the need for a mechanical airway.7
Lower Airway Injury
Thermal Injury
Direct thermal injury to lower airway structures is an uncommon occurrence (5%). The low incidence of lower airway injury is due to the dissipation of heat during travel through the airway and to reflexive closing of the glottis at high temperatures (150°C). Small particulate matter (<5 µm) can travel to terminal bronchi and alveoli and cause damage to cell populations such as epithelial cells and alveolar macrophages.8
Tracheobronchial Injury
Cytoplasmic vacuolization and cytoplasmic blebbing are seen in epithelial cells of the bronchial tree 48 hours after severe smoke inhalation.9 This is followed by epithelial necrosis, hemorrhage, and perivascular congestion. Such damage initiates an inflammatory cascade that recruits activated neutrophils and macrophages to the injured area, causing further damage.10 Airway congestion and increased lymphatic flow lead to obstruction of bronchial segments and impaired gas exchange.
Parenchymal Damage
Direct damage to the lung epithelium causes the recruitment of inflammatory mediators that produce increased parenchymal damage; neutrophils are among the first mediators recruited. In addition to growth factors and cytokines, neutrophils release reactive oxygen species and proteases that cause direct cellular damage. Such damage triggers further inflammation and leads to pulmonary dysfunction.11 This dysfunction includes evidence of increased apoptosis of lung epithelial cells, leading to decrease in surfactant release and defective surfactant mechanisms, resulting in obstruction and collapse of lung segments.12 In addition, alveolar macrophages release free radicals that cause further damage to the pulmonary parenchyma.13 With extensive destruction and inflammation, pulmonary compliance is reduced and gas exchange is impaired, leading to altered pulmonary blood flow patterns and ventilation/perfusion mismatches.14
Damage From Asphyxiants
Carbon Monoxide Toxicity
CO is an odorless, nonirritating gas that is responsible for up to 600 accidental deaths per year. The pathology of CO poisoning is attributable to its ability to rapidly diffuse into the bloodstream and bind to the iron moiety of heme that is normally bound by oxygen. Because of higher affinity (240 times) for the heme-binding site, CO easily displaces oxygen and impairs the ability of hemoglobin to deliver oxygen. The stoichiometry of hemoglobin is also altered, further impairing oxygen delivery by the other sites of hemoglobin. CO also binds to enzymes within mitochondria involved in the utilization of oxygen by cells and tissues. By binding to these enzymes, myoglobin, cytochromes, and NAPDH reductase, cellular and local tissue acidosis increases, further impairing oxygen delivery. This results in progressive cellular dysfunction and ultimately organ failure.15
Neurologic symptoms are often the first manifestation of CO poisoning. Mild carboxyhemoglobin levels (5%–10%) are usually well tolerated. When concentrations reach 10% to 30%, symptoms usually begin to manifest. Headaches, nausea, and dizziness are the most common initial symptoms in mild to moderate CO poisoning. With severe poisoning (50% carboxyhemoglobin levels), more significant neurologic symptoms occur, such as syncope, seizures, and coma. The diagnosis of CO poisoning is made based on the combination of physical symptoms and elevated levels of systemic carboxyhemoglobin. Pulse oximetry values do not differentiate between carboxyhemoglobin and oxyhemoglobin and thus remain paradoxically elevated. Blood PO2 level remains normal because it reflects oxygen dissolved in plasma that is not affected by CO.16 Neurologic symptoms may persist in the form of delayed neuropsychiatric sequelae with symptoms that include a persistent vegetative state, parkinsonism, short-term memory loss, behavioral changes, hearing loss, and psychosis. These symptoms may manifest anytime from 3 to 240 days after recovery. Approximately 50% to 75% of patients with delayed neuropsychiatric sequelae recover fully in 1 year.17
The hallmark of treatment of CO poisoning involves maintaining adequate oxygenation. The CO half-life decreases from 6 to 8 hours to 40 to 80 minutes within 1 hour of treatment with 100% oxygen. Administration of 100% oxygen can be done via facemask or by mechanical ventilation. Hyperbaric oxygen treatment has been shown to have an advantage over normobaric oxygen treatment for CO poisoning; when administered in a hyperbaric chamber, the half-life of CO decreases to 15 to 30 minutes.18 However, given the limited number of hyperbaric chambers available, the widespread use of hyperbaric therapy is limited.17,19
Cyanide Toxicity
Cyanide inhalation is a potentially life-threatening occurrence that requires immediate intervention. Once inhaled, cyanide rapidly crosses into the blood and disrupts normal cellular utilization of oxygen by binding to cytochrome oxidase, thus interfering with cellular respiration. As in CO toxicity, cellular lactic acid production is increased, and cellular dysfunction soon follows.20
Diagnosis of cyanide toxicity is made by careful review of the history of inhalation, duration of exposure, and clinical symptoms. Physical manifestations of cyanide poisoning include headache and confusion followed by fixed pupils, bradycardia, hypotension, seizures, arrhythmias, heart block, cardiac failure, and coma. Diagnosis is aided by measurement of blood concentrations of cyanide, which are considered toxic at levels greater than 0.5 mg/L.20
The treatment of cyanide toxicity includes administration of oxygen as well as decontamination agents. When cyanide toxicity is suggested, 100% oxygen should be administered immediately. This can be done under normobaric or hyperbaric conditions, but the use of hyperbaric chambers is yet to be proven to provide a benefit.21 Amyl and sodium nitrates are often used as decontamination agents; these compounds induce the formation of methemoglobin, to which cyanide has high affinity. Methemoglobin thus acts as a scavenger for cyanide. Another utilized compound for treatment of cyanide toxicity is sodium thiosulfate, which acts by transferring a sulfur group to cyanide and converting it to renally excreted thiocyanate. Hydroxycobalamin (not approved by the U.S. Food and Drug Administration [FDA]) detoxifies cyanide by binding to it and forming cyanocobalamin, an inert vitamer of the vitamin B12 family.22,23
Features of Specific Irritants
Smoke produces a variety of compounds that have been shown to cause or initiate damage to the lung. The mechanism of damage for many of these compounds is unknown, but the location of damage within the respiratory tract is related to the ability of the compound to reach that location (Table 70-1). The ability of gases and toxins to exert damage on the tracheobronchial tree depends on the capacity of the toxin to reach different areas of the airway.5 Water solubility affects the location of deposit of gases and toxins. Mucous membranes line much of the upper respiratory tract, which allows gases that are highly water soluble to be absorbed in the upper respiratory tract and cause irritation to these structures. Because less-soluble gases are not absorbed in the upper airway, they travel to the lower airway and cause irritation and damage to those structures.3
TABLE 70-1 Specific Lung Irritants
Chemical Irritants | Properties | Mechanism of Toxicity |
---|---|---|
Smoke | ||
Acrolein | Lipophilic | Direct epithelial damage |
Industrial | ||
Chlorine | Water soluble | Forms free radicals |
Phosgene | Low solubility | Causes the release of arachidonic acid metabolites |
Nitric oxide | Lipid soluble | Causes lipid peroxidation |
Sulfur dioxide | Water soluble | Causes lipid peroxidation |
Ammonia | Water soluble | Forms hydroxyl ions and causes liquefactive necrosis |
Acrolein
Acrolein, a lipophilic aldehyde carbonyl with an attached vinyl group, is a toxic compound found in the inhalation of several materials, including tobacco smoke, vehicle exhaust, and wood smoke. Its lipophilic nature allows it to pass through the upper airway and penetrate lower airway structures, where it is eventually absorbed. Systemic acrolein is metabolized by the liver through reaction with glutathione, resulting in the generation of mercapturic acids that are renally excreted.24 This transformation, however, does not occur as readily in the lung, and thus acrolein levels remain elevated in lung tissue and cause direct epithelial damage.25,26
Hydrogen Chloride
The toxicity of chlorine is related to its water solubility as well as duration of exposure. Chlorine is a moderately water-soluble gas that can penetrate deep into the lower lung structures. Within the upper airway, chlorine has a direct irritant effect that causes inflammation and edema. Within the lower airway, hydrogen chloride forms reactive ions that create free radicals that react with various compounds and ultimately lead to mucosal destruction, pulmonary edema, and parenchymal damage.27,28
Phosgene
Phosgene is an acylating agent found in plastics and aniline dyes. It is a low-soluble gas that when inhaled produces severe pathology within the bronchoalveolar spaces. Phosgene reacts with glutathionine and causes the release of arachidonic acid metabolites.29–31
Ammonia
The inhaled form of ammonia, anhydrous ammonia, is highly water soluble and is mostly absorbed in the upper airway. However, owing to its toxic nature, lower airway structures can also be affected. Ammonia exerts its effects by reacting with tissues, creating hydroxyl ions, which results in liquefactive necrosis.32
Nitrogen Oxide
Nitric oxides are highly lipid-soluble compounds that are absorbed in the lower lung regions. Nitric oxides exert their toxic effects by the production of free radicals through lipid peroxidation, leading to parenchymal damage and pulmonary edema.33,34
Role of a Cutaneous Thermal Injury
The combined effect of thermal injury and inhalation injury is synergistic on morbidity and mortality, creating increased pulmonary vascular changes and inflammation that lead to decreased pulmonary compliance and pulmonary function. Burn injury alone increases vascular permeability and can result in pulmonary edema. When associated with inhalation injury, this increase in pulmonary edema is exacerbated and results in a massive influx of inflammatory mediators, which increases damage to the lung parenchyma.36 With increasing damage to lung parenchyma, pulmonary compliance decreases and ventilation/perfusion mismatch occurs. With the resulting edema, atelectasis and consolidation of the lung (from the increased vascular permeability and increased lymphatic flow) set the stage for secondary bacterial infections.37–39 In addition, pulmonary edema and decreased pulmonary compliance result in increased intrathoracic pressure, which causes a left side–dominant myocardial depression and contributes to the altered hemodynamic profile observed in combined thermal and inhalation injury.40
Postinhalation Pulmonary Complications
Local Factors
Ciliary Dysfunction
Inhalation injury causes direct damage to mucosal and ciliary elements, leading to ciliary dysmotility. Such damage is caused by several agents, including acrolein and other aldehydes.26 In addition, the release of inflammatory mediators (such as thromboxane) has been shown to decrease mucociliary activity.41 This allows particles and toxins to exert their effects on local defense mechanisms as well as initiate a cascade that leads to parenchymal damage and bacterial infection.42
Pulmonary Alveolar Macrophage
Alveolar macrophage numbers increase in smoke inhalation injury, with resultant increases in circulating tumor necrosis factor alpha (TNF-α) and interleukin (IL)-8 within 24 hours of injury, leading to extensive subsequent tissue damage.43 In addition, the phagocytic function of macrophages is diminished, which precipitates increased lung parenchymal exposure to various toxins and bacteria.13,44
Proteasomes
Analysis of bronchoalveolar lavage fluid obtained on admission following inhalation injury demonstrates decreased circulating levels of proteasome 26S, with reduced specific proteasome activity. Furthermore, decreased proteasome 26S concentrations are associated with increased rates of developing ventilator-associated pneumonia, suggesting that insufficient proteasome function may contribute to increased susceptibility for pulmonary complications following inhalation injury.45
Surfactant
Severe inhalation injury and subsequent increased capillary permeability alter surfactant production and function. In lung injury models, surface tension generated by surfactant is reduced, leading to a loss of the normal force that maintains alveolar patency; this ultimately results in alveolar collapse.12 In addition, reduction in surfactant protein levels (SP-A, SP-B) may lead to reduced lung defense mechanisms, further enhancing evolving lung pathology following inhalation injury.46
Infections
Infectious complications are a common occurrence with burn injury. Pneumonia in particular can occur in up to 50% of severely burned patients, with the majority (65%) of these patients requiring mechanical ventilation.47,48 The mortality rate doubles in patients with concomitant inhalation injury and nosocomial pneumonia, reaching rates as high as 86%.49 The root cause of this synergistic effect has to do with both direct lung injury from inhalation and immune dysfunction and systemic inflammation, creating an environment susceptible to opportunistic infections such as Pseudomonas aeruginosa and Acinetobacter species and leading to fulminant nosocomial pneumonias.50,51
Pathogens
The organisms that cause infections in inhalation injury can be organized into groups according to the pathogens’ exogenous/endogenous state or to the time from injury to infection. Organisms that are endogenous and cause infections are those present in the oral and respiratory tract or those in the gut at the time of admission. These include Staphylococcus aureus, Streptococcus pneumoniae, Haemophilus influenzae, Proteus mirabilis, and Escherichia coli. Exogenous organisms are those that are acquired during the hospital course and were not present in either the gastrointestinal or respiratory tract. These include methicillin-resistant S. aureus, Acinetobacter, Pseudomonas aeruginosa, and other opportunistic organisms (e.g., Candida). Within these groupings, early infections tend to be from endogenous organisms, whereas infections at a later time tend be from exogenous organisms.47 P. aeruginosa infection has been shown to significantly increase mortality rates in burn-injured patients. The emergence of Acinetobacter species has increased in burn injury and is difficult to treat owing to its easy transmissibility and multidrug resistance.52 Like P. aeruginosa, infections by Acinetobacter tend to occur later in the time course of treatment and carry a high mortality rate.53 A thorough understanding of the pathogens involved in inhalation injury and the time course for the onset of these infections is important to tailor effective empirical antimicrobial therapy in order to avert serious complications.
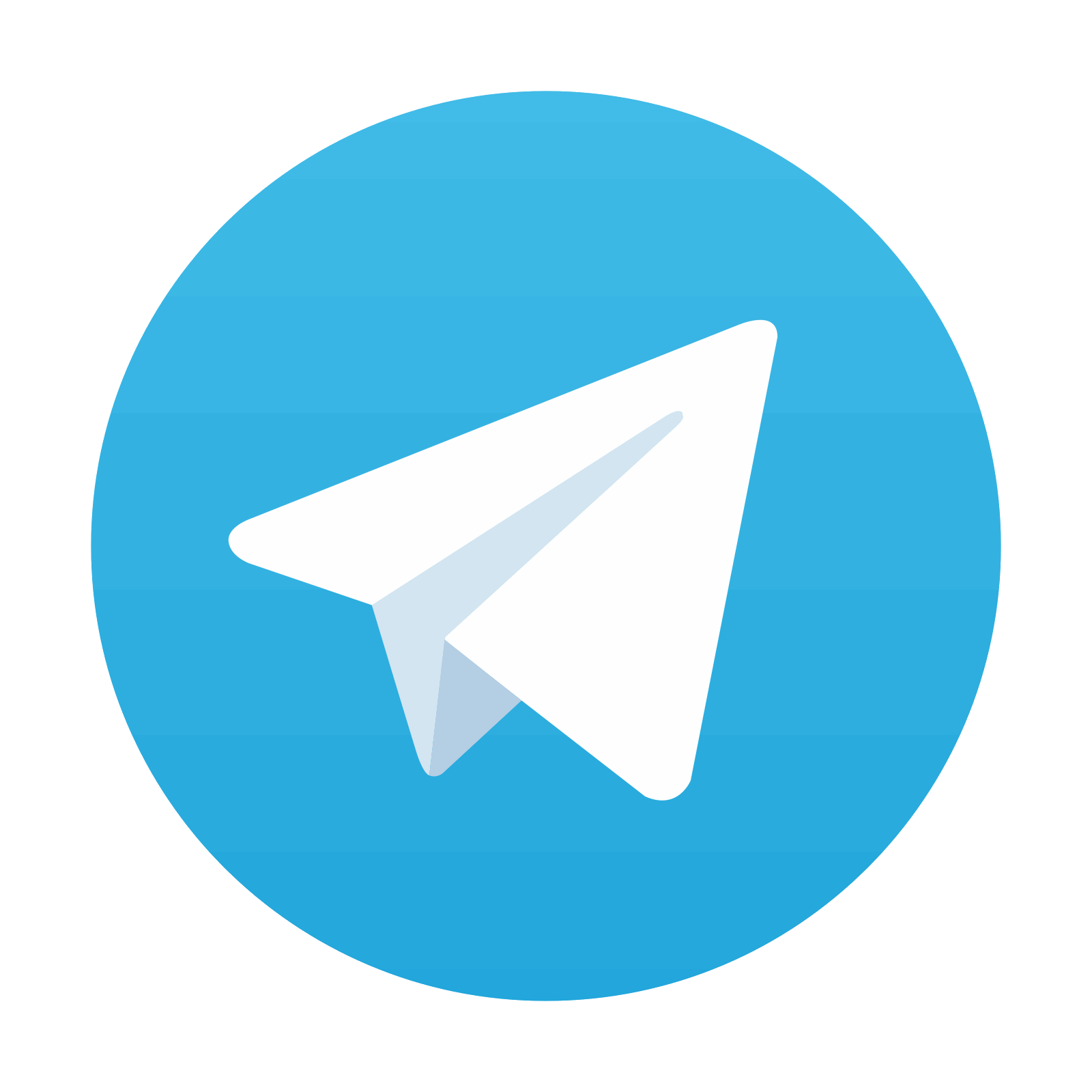
Stay updated, free articles. Join our Telegram channel

Full access? Get Clinical Tree
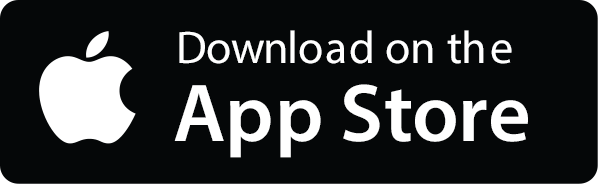
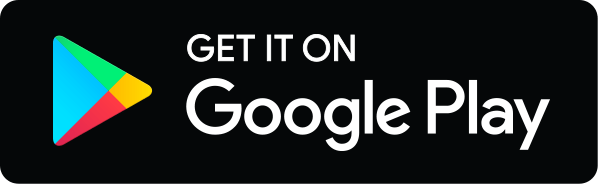