Abstract
This chapter presents a concise description of the autonomic nervous system (ANS) with a focus on clinical elements related to anesthesiology. Beginning with a review of the anatomic divisions of the sympathetic (SNS) and parasympathetic (PNS) systems, the narrative links these structures to the interplay between the seemingly antagonistic physiologic actions of the two divisions. A close look at ANS cellular physiology reveals the receptor and effector mechanisms responsible for these differential effects, leading the reader to understand where anesthetic pharmacology might affect cardiovascular, pulmonary, gastrointestinal and humoral systems. Well-known ANS reflexes that are seen during the conduct of an anesthetic highlight the interaction between the SNS and the PNS. A review of the effect of anesthetics and ANS disease outlines the importance of the ANS in anesthetic practice. Emerging developments, including a radical change in the anatomic structure of the ANS, concludes the chapter.
Keywords
autonomic nervous system, sympathetic nervous system, parasympathetic nervous system, autonomic reflexes, disease states
-
Chapter Outline
Historical Considerations
A specialized taxonomy of the autonomic nervous system (ANS) has been developing since the time of Galen ( ad 130–200). In the early 1900s, Langley first referred to the ANS. He used the term sympathetic nervous system (SNS) as described by Willis in 1665, and introduced the second division as the parasympathetic nervous system (PNS) in 1921. Although Langley initially described only the visceral motor system (efferent fibers), the existence of visceral reflex arcs necessitated the inclusion of the sensory (afferent) portions of the ANS. Early anesthesia textbooks did not contain explicit information dealing with the ANS, but dealt with the practice of anesthesia, elucidating basic considerations of pharmacology and anesthetic technique. The evolution of the comprehensive anesthesia textbook has led to extensive chapters on the ANS.
The ANS maintains cardiovascular, thermal, and gastrointestinal homeostasis. A firm understanding of the basic anatomy and physiology of the ANS forms an important foundation for the practice of anesthesiology. ANS structure, function, and reflexes are critical to the support of the circulation under anesthesia. This chapter reviews anatomy and physiology of the ANS relevant to anesthesia.
Anatomy and Physiology
ANS anatomy is composed of central control and feedback areas, sensory receptors, peripheral effectors, and reflex conduction pathways. In addition, complex interactions occur between the ANS and the endocrine system (see Chapter 36 ). The renin-angiotensin system, antidiuretic hormone, glucocorticoid and mineralocorticoid responses, and insulin interact via an increasing number of receptor subtypes to maintain physiologic homeostasis ( Fig. 13.1 ).

There are no distinct centers of autonomic function in the cerebral cortex. However, input from various sensory systems can affect higher cortical centers, be processed, and result in efferent autonomic activity. Tachycardia and peripheral vasoconstriction heralding a “fight-or-flight” response or a vasovagal response (fainting) are well-known examples of this higher cortical sensory processing. External stimuli representing a threat or danger are detected by the senses of hearing, touch, smell, or sight. These signals are sent to the brainstem, where reflex responses are processed in the hypothalamus and the limbic forebrain. Higher cortical centers provide descending input to the paraventricular nucleus of the hypothalamus, which has projections to sympathetic and parasympathetic nuclei. Chronic stress alters these structures and their function, leading to both sensitization and habituation of the stress response.
The hypothalamus is the midbrain center that processes sympathetic and parasympathetic functions, temperature regulation, fluid regulation, neurohumoral control, and stress responses. Hunger, sleep, and sexual function are also regulated by the hypothalamus, dependent on both cortical input and complex feedback control. The anterior hypothalamus controls temperature, while the posterior hypothalamus is involved in water regulation. The hypothalamic-pituitary axis is a part of the ANS that ultimately regulates long-term blood pressure control and stress responses.
The output centers of the ANS reside in the medulla oblongata and the pons of the brainstem. Immediate control of blood pressure, heart rate, cardiac output, and ventilation is organized and integrated in specific nuclei. Tonic impulses from nuclei such as the nucleus tractus solitarius maintain blood pressure and respond to afferent signals from the sensory side of the ANS. These afferent impulses from the vagus (X) and glossopharyngeal (XI) nerves result in vasodilation and bradycardia (see the section on ANS reflexes).
The ANS is anatomically and functionally divided into two complementary systems, the SNS and the PNS (see Fig. 13.1 ). The peripheral SNS is controlled by the thoracolumbar segment of the spinal cord, while PNS control arises from the brainstem nuclei and sacral segments (see Fig. 13.1 ). Activation of the SNS produces diffuse physiologic responses, while the PNS exerts local control of innervated organs. Both systems have efferent pathways through peripheral ganglia; the SNS ganglia are located close to the thoracolumbar spine, and the PNS ganglia are situated near or inside the innervated organs (see Fig. 13.1 ; Fig. 13.2 ). Ganglia serve as synaptic relay stations, and in the SNS coordinate an efferent mass action response through signal amplification. Thus one preganglionic SNS fiber can activate 20 to 30 postganglionic sympathetic neurons and their fibers. In contrast, PNS preganglionic fibers terminate in ganglia located in proximity to the innervated organs and affect only one to three postganglionic neurons. The close proximity of PNS ganglia to their effector organs is the anatomic basis of the more focused and specific responses elicited by PNS activation.

Most organs are affected by both the SNS and the PNS. Different organs have their resting tone dominated by the SNS or the PNS, and this ratio can change depending on pathophysiologic states and also can change over the lifetime of an individual. For instance, newborns are dominated by parasympathetic responses; hence bradycardia can be seen in 20% of unpremedicated infants during stressful situations such as anesthetic induction and airway manipulation, while it is uncommon in adults. As another example, vasoreactivity of the major blood vessels, arteries, and arterioles is primarily responsive to the SNS, while PNS cardiovascular effects reside mainly at the level of the heart. The differential effects of the ANS in various organs and organ systems are summarized in Table 13.1 .
Receptor | Effector | Response to Stimulation |
---|---|---|
Sympathetic Nervous System | ||
α 1 | Smooth muscle (vascular, iris radial, ureter, trigone, bladder sphincters) | Constriction |
α 2 | Presynaptic SNS nerve endings | Inhibition of NE release |
Brain | Neurotransmission | |
β 1 | Heart | Increase rate, contractility, conduction |
Adipose tissue | Lipolysis | |
β 2 | Blood vessels | Dilation |
Bronchioles | Dilation | |
Kidney | Renin secretion | |
Liver | Gluconeogenesis, glycogenolysis | |
Endocrine pancreas | Insulin secretion | |
Uterus | Relaxation | |
D 1 | Blood vessels | Dilation |
D 2 | Presynaptic SNS nerve endings | Inhibition of NE release |
Parasympathetic Nervous System | ||
M 1 | Skeletal prejunctional nerve endings | Facilitate ACh release |
M 2 | Lung–presynaptic PNS nerve endings | Inhibits ACh release |
Visceral organs | Increase | |
M 3 | Lung smooth muscle, postsynaptic | Bronchoconstriction |
N 1 | PNS and SNS ganglion | Ganglionic blockade |
N 2 | Skeletal muscle | Muscle contraction |
In general, the SNS modulates the activity of vascular smooth muscle, cardiac muscle, and various glands (especially the adrenal gland); this modulation is critical for the fight-or-flight response. In contrast, the PNS modulates “rest-and-digest” functions such as salivation, lacrimation, urination, digestion, defecation, and sexual arousal.
Sympathetic Nervous System
The SNS is formed from preganglionic fibers in the thoracolumbar segments (T1-L3) of the spinal cord arising from the intermediolateral gray column (see Fig. 13.1 ). These myelinated fibers enter the paravertebral ganglia and travel a variable distance up or down the sympathetic chain to synapse with the neuronal cell bodies of postganglionic sympathetic neurons. The unmyelinated postganglionic fibers then innervate their respective organs. An exception to this rule is the adrenal gland, where the preganglionic fibers do not synapse in the thoracic ganglia, but course through the sympathetic chain into the adrenal medulla. The chromaffin cells in the adrenal medulla are derived from neuronal tissue and essentially function as the postganglionic cells.
The stellate ganglion consists of postganglionic neurons that provide sympathetic innervation to the head and neck. Preganglionic fibers from the first four or five thoracic segments form this ganglion as well as the superior and middle cervical ganglia. Local anesthetic injected in the vicinity of the stellate ganglion blocks the sympathetic fibers coursing to the ipsilateral head and neck and may result in Horner syndrome, characterized by ptosis, miosis, enophthalmos, and anhydrosis on the affected side. This syndrome might be seen as a direct effect of a stellate ganglion block or be experienced as a side effect of a brachial plexus block owing to its close proximity to the stellate ganglion. Similarly, blockade of the lumbar plexus produces a sympathectomy in the lower extremities, and peripheral nerve block often produces a sympathectomy in the affected limb because the postganglionic sympathetic nerve fibers travel along the somatic nerves.
Parasympathetic Nervous System
Preganglionic fibers in the PNS arise from the midbrain, medulla oblongata, and sacral segments of the spinal cord. Cranial nerves II, VII, IX, and X carry preganglionic parasympathetic fibers directly to ganglia located near or directly in innervated organs. The sacral segments S2-S4 provide innervation to the rectum and genitourinary tissues (see Fig. 13.1 ).
The vagus nerve (X) is the major carrier of parasympathetic neuronal traffic. These preganglionic fibers affect the heart, lungs, and abdominal organs with the exception of the distal portion of the colon. A combination of the distal location of the ganglion and the smaller two- to threefold amplification factor between preganglionic and postganglionic fibers causes parasympathetic effects to be specific to each organ.
Cellular Physiology
Preganglionic Neurons
Synaptic transmission through ANS ganglia is similar in both the SNS and the PNS. Preganglionic neurons in both the SNS and PNS are cholinergic. Acetylcholine (ACh) is stored in synaptic vesicles and released by a calcium ion (Ca 2+ )-dependent process upon nerve terminal depolarization ( Fig. 13.3 ). ACh then interacts with postsynaptic receptors to depolarize the postsynaptic membrane. The principal ganglionic receptors are excitatory nicotinic ACh receptors, related to the ACh receptors at the neuromuscular junction (see Chapter 21 ). Thus many neuromuscular blocking agents have cardiovascular side effects mediated by their actions at the level of ANS ganglia. Recent advances in the pharmacology of these drugs have been directed at decreasing these ganglionic actions (see Chapter 22 ).

There are two distinct nicotinic receptor types, designated neuronal and muscle nicotinic ACh receptors ( Fig. 13.4 ). Neuronal ACh receptors expressed in the autonomic ganglia are composed of α 3 β 4 subunits and are blocked by older neuromuscular blockers (e.g., gallamine), leading to ganglionic blockade. The neuromuscular junction has muscle nicotinic ACh receptors (composed of αβδε subunits in adults) that are blocked selectively by the newer neuromuscular blocking agents, resulting in few side effects. Volatile anesthetics and ketamine are potent inhibitors both at α 4 β 2 in the central nervous system (CNS) and ganglionic α 3 β 4 receptors. The development of neuromuscular blocking agents has been focused on reduction of muscarinic side effects and elimination of ganglionic blockade. Structure-activity relationships indicate that the presence of quaternary ammonium moieties facilitates binding at the ACh site, whereas interionic distances may play a role in diminishing ganglionic as well as muscarinic cross-reactivity.

Both nicotinic and muscarinic agonists and blockers interact at the level of the ganglia, the effects of which summate to either excite or inhibit the postganglionic neuron, and subsequently inhibit the effector organ. Thus the ganglionic synapse serves complex integrative and processing functions during normal physiology and while under the influence of anesthetic agents.
Postganglionic Neurons
Sympathetic Nervous System
Epinephrine, norepinephrine, and dopamine are the classic neurotransmitters of sympathetic synaptic transmission released from postganglionic neurons ( Fig. 13.5 ); they interact with adrenergic receptors to effect sympathetic physiologic responses. As shown in Fig. 13.6 , these neurotransmitters and their receptors can be characterized at different levels. Building on the original observation by Ahlquist, adrenergic “receptors” are of two different types (α and β) classified in terms of the overall physiologic response they elicit (the “classic pharmacology” approach). Modern pharmacology further categorizes these receptors in terms of their molecular biology (e.g., DNA sequence and protein structure). From a mechanistic perspective, these receptors can also be classified in terms of how their signals are transduced (i.e., which G-protein subtype is involved) and how the response is effected (i.e., what ion channels or enzymes are involved).

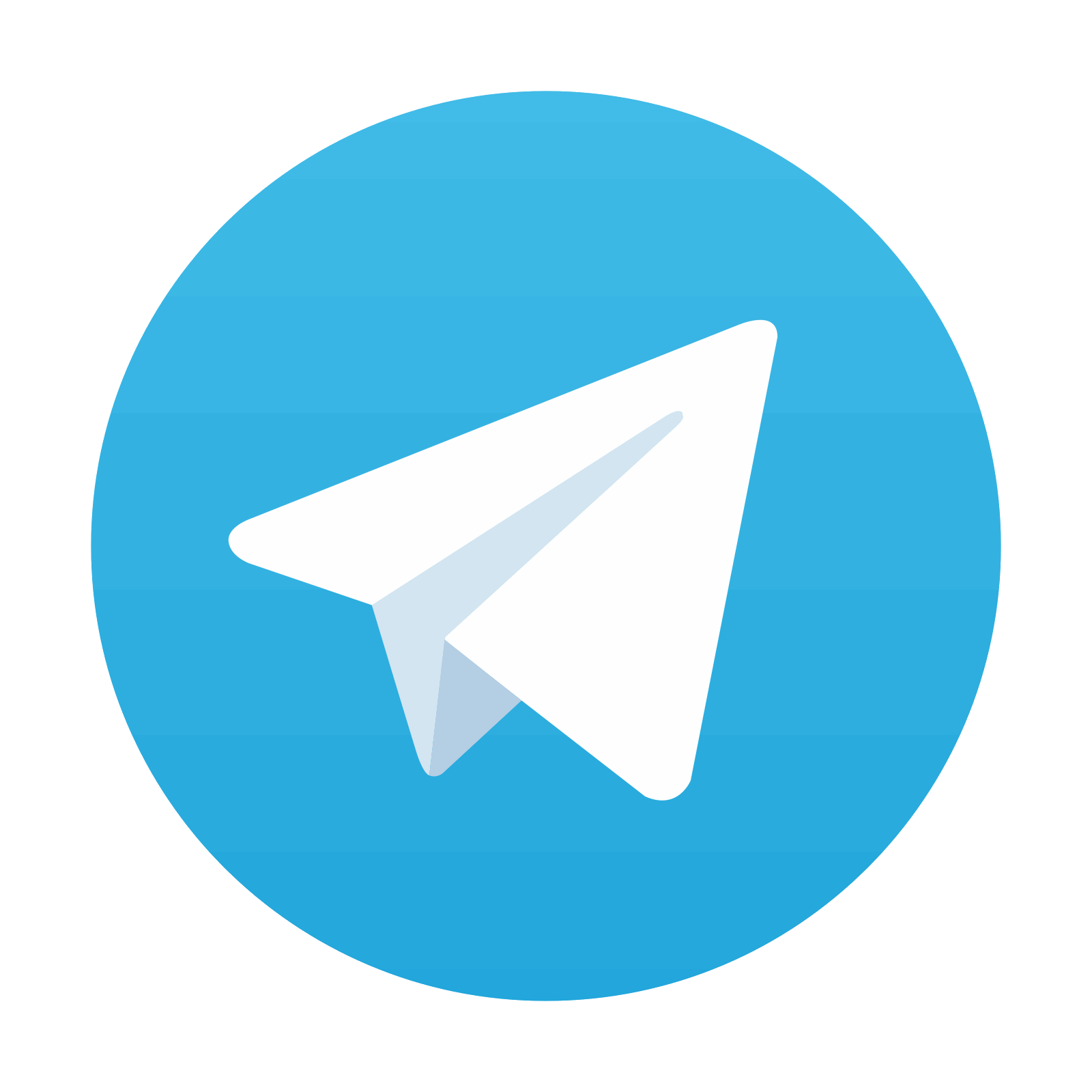
Stay updated, free articles. Join our Telegram channel

Full access? Get Clinical Tree
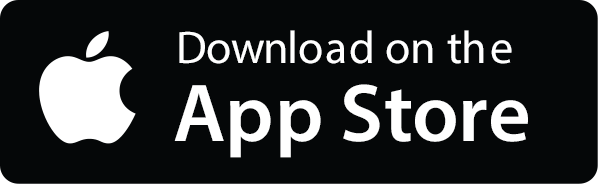
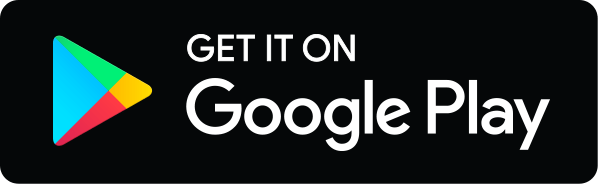