Fig. 9.1
Number of reported adult lung transplants by year and procedure type reported to the International Society for Heart and Lung Transplantation Registry (Data from Wong et al. [3])
Preoperative Evaluation
LTx surgery tends to be performed on an emergency basis providing the anesthesiologist limited time in which to conduct an expedited but thorough preoperative evaluation. Potential lung transplant recipients undergo a comprehensive multidisciplinary pretransplant evaluation, the results of which must be immediately available to the anesthesia team. Key investigations that impact intraoperative management are reviewed in Table 9.1.
Table 9.1
Relevance of key preoperative investigations to anesthesia management
Investigation |
Relevant information |
---|---|
Spirometry and lung volumes |
Type and severity of lung disease influences ventilator management |
Ventilation perfusion scan |
Indicates which lung will better tolerate one-lung ventilation. Worst lung will be the one to be transplanted first |
Computed chest tomogram and/or plain radiograph |
Anatomic factors possibly affecting double-lumen tube placement and dissection of mediastinum for pneumonectomy |
Echocardiogram |
Left and right ventricular size, wall thickness, and systolic function; valvular disease; estimated pulmonary artery pressure; presence of intracardiac shunts |
Cardiac catheterization |
Hemodynamics/right-sided pressures extent of coronary artery disease |
Since a significant time interval may have elapsed since completion of the pretransplant evaluation, the anesthesiologist must ascertain if any changes in health status have occurred in the interim (e.g. worsening functional status, increased oxygen requirements, signs/symptoms of decompensated right heart failure). Recipients should be questioned regarding potential contraindications to transesophageal echocardiography. The patient’s fasting status should be determined, with consideration given to premedication for gastric aspiration prophylaxis . A discussion about postoperative analgesia, blood transfusion, and postoperative mechanical ventilation should also take place at this time. The availability of blood products should be confirmed with blood bank personnel. The anesthesiologist should ensure that critical preoperative medications such as pulmonary vasodilators, bronchodilators, and antibiotics are continued without interruption.
Appropriate perioperative antimicrobial prophylaxis should be initiated based on available microbiology data and local antimicrobial resistance patterns. The anesthesiologist is also typically responsible for initiation of the immunosuppressive regimen, which commonly includes methylprednisolone and an induction agent such as alemtuzumab (anti-CD52, Campath) or basiliximab (anti-CD25, Simulect). Alemtuzumab is commonly associated with infusion reactions (fever, chills, hypotension, urticaria, dyspnea) and therefore requires premedication with acetaminophen 500–1000 mg, famotidine 20 mg (anti-H2) and diphenhydramine 50 mg (anti-H1) 30 min prior.
Once the surgical team confirms the suitability of the donor lung(s) for transplant, the recipient is taken to operating theater. Limited premedication (midazolam 1–2 mg) may be considered according to the patient’s level of anxiety and respiratory compromise, but only under continuous monitoring for decompensation.
Vascular Access and Monitors
Reliable, large-bore intravenous access is required as is the availability of a rapid infusion device. Typically, invasive arterial pressure monitoring is established prior to induction of general anesthesia allowing continuous monitoring of systemic arterial pressure and blood gases. Femoral artery pressure correlates best with central aortic pressure and avoids issues of poor radial artery line reliability associated with arm positioning on over-arm boards during lengthy surgical procedures. Arterial catheterization at a second site allows for redundancy and permits uninterrupted arterial pressure monitoring during frequent sampling of blood gas samples.
Central venous and pulmonary artery (PA) catheters may be placed before or after induction of anesthesia depending on availability of suitable peripheral venous access and the patient’s ability to assume a recumbent position. PA catheters that allow continuous measurement of cardiac output, RV loading conditions and mixed venous oxygen saturation (SvO2) are preferable to allow rapid assessment of intraoperative changes in cardiac output and oxygen delivery. Although significant tricuspid regurgitation (TR) confounds cardiac output measurement by such catheters, SvO2 measurements remain valuable provided the results are calibrated periodically against laboratory measurements of mixed venous blood gas and hemoglobin values.
Near infrared reflectance spectroscopy monitoring has proven very useful in monitoring the adequacy of cerebral oxygenation during both ECMO and CPB, as well as detecting lower limb ischemic complications related to femoral cannulation [3].
Awareness monitoring is probably routinely indicated during LTx on the basis of widespread use of muscle relaxants and the frequent occurrence hemodynamic depression necessitating reductions in end-tidal anesthetic gas concentrations to below 0.7 MAC.
Induction
Due to the lack of evidence of the superiority of any particular induction regimen, the choice is mainly influenced by individual and institutional preferences. The overall aim is to avoid factors which increase PVR (hypoxia, hypercarbia, acidosis, light anesthesia) and thereby prevent the occurrence of pulmonary hypertensive crisis resulting in decompensated RV failure and circulatory collapse. Avoidance of systemic hypotension and subsequent right ventricular (RV) hypoperfusion is of paramount importance. A common choice of induction medications is low dose fentanyl combined with etomidate. Adequate depth of anesthesia is necessary to avoid an abrupt sympathetically mediated increase in pulmonary vascular resistance (PVR) in response to laryngoscopy and tracheal intubation. At the same time, excessive anesthetic depth and its attendant hemodynamic depression is also to be avoided. For these reasons, rapid sequence induction may be undesirable and a brief period of gentle mask ventilation with cricoid pressure may be necessary to avoid rapid oxyhemoglobin desaturation while titrating anesthetics and achieving muscle relaxation.
Vasopressor and inotropic infusions should be ready for immediate infusion on primed and programmed infusion pumps; epinephrine infusion may be started preemptively to support the circulation during induction of anesthesia in patients with RV dysfunction. Patients judged to be at excessive risk of cardiovascular collapse upon induction of general anesthesia and positive pressure ventilation (e.g. patients with severe pulmonary hypertension and evidence of right ventricular (RV) dysfunction) may have femoral vessels accessed under local anesthesia in order to facilitate rapid cannulation should rescue with extracorporeal support become necessary. Depending on the surgical plan, preemptive cannulation and/or institution of extracorporeal membrane oxygenation (ECMO) may even be considered prior to induction of anesthesia [4]. For all patients, we ensure that both the cardiothoracic surgeon and perfusionist are available with a primed cardiopulmonary bypass unit on standby should urgent institution of extracorporeal support become necessary.
Airway Management
A double lumen endobronchial tube (DLT) provides the most expedient means of lung isolation, particularly for bilateral sequential lung transplants during which a bronchial blocker would need to be repositioned multiple times. A left DLT is preferred due to the comparatively short length of the right main bronchus that necessitates withdrawal of a right DLT during bronchial anastomosis. DLTs also provide means of enabling postoperative independent lung ventilation, occasionally necessary following single lung transplant for patients with emphysema.
Intubation with a single lumen tracheal endotracheal tube may be initially preferable in two circumstances: (1) to more quickly and easily secure the airway when intubation is difficult, and (2) to facilitate toilet bronchoscopy and obtain bronchial washing for microbiological cultures in patients with cystic fibrosis and other forms of bronchiectasis.
Positioning
Single LTx can be performed either through a posterolateral thoracotomy in the lateral position (with access to the femoral vessels), or, via anterolateral thoracotomy in the supine position with a wedge placed under the operative side for better access. Double lung transplant is performed in the supine position, which increases shunt fraction during one-lung ventilation relative to the lateral position. One of three approaches can be used for double LTx: (1) bilateral transverse thoracosternotomy (“clamshell”) incision, (2) bilateral anterolateral thoracotomies sparing the sternum, or (3) median sternotomy, depending on patient factors and institutional preference.
Arms are often abducted or elevated and secured to an over-arm board which improves surgical exposure, but impedes access to and can limit the usefulness of upper extremity venous and arterial catheters. Care should be taken during arm positioning to avoid excessive stretching of the brachial plexus and peripheral nerve compression.
Prior to skin prep and sterile draping, placement of multifunction external defibrillator pads is advisable to provide rapid means of cardioversion should unstable arrhythmias arise.
Transesophageal Echocardiography
Transesophageal echocardiography (TEE) is used extensively during lung transplant surgery for diagnostic and monitoring purposes. Intraoperative TEE during LTx is supported by ASA practice guidelines as “the nature of the planned surgery or the patient’s known or suspected cardiovascular pathology might result in severe hemodynamic, pulmonary, or neurologic compromise” or in situations of unexplained persistent hypotension or hypoxia [5]. The authors routinely use TEE intraoperatively during LTx unless contraindicated and place the TEE probe immediately after tracheal intubation to begin treatments directed at optimization of right ventricular performance and loading conditions.
In patients with severe pulmonary hypertension being evaluated for lung transplantation who had already had transthoracic echocardiograms, Gorcsan et al found that TEE provided new findings that significantly altered surgical decision making in 25 % of patients [6].
The initial intraoperative TEE examination focuses on the assessment of right and left ventricular function, identification of intracardiac shunts (Fig. 9.2a–c), and baseline assessment of the pulmonary arteries and veins. Echocardiographic assessment of the right ventricle and tricuspid regurgitation (TR) is summarized in Tables 9.2 and 9.3. A dilated RV with an under-filled left ventricle (LV) suggests RV dysfunction (Fig. 9.3a, b).
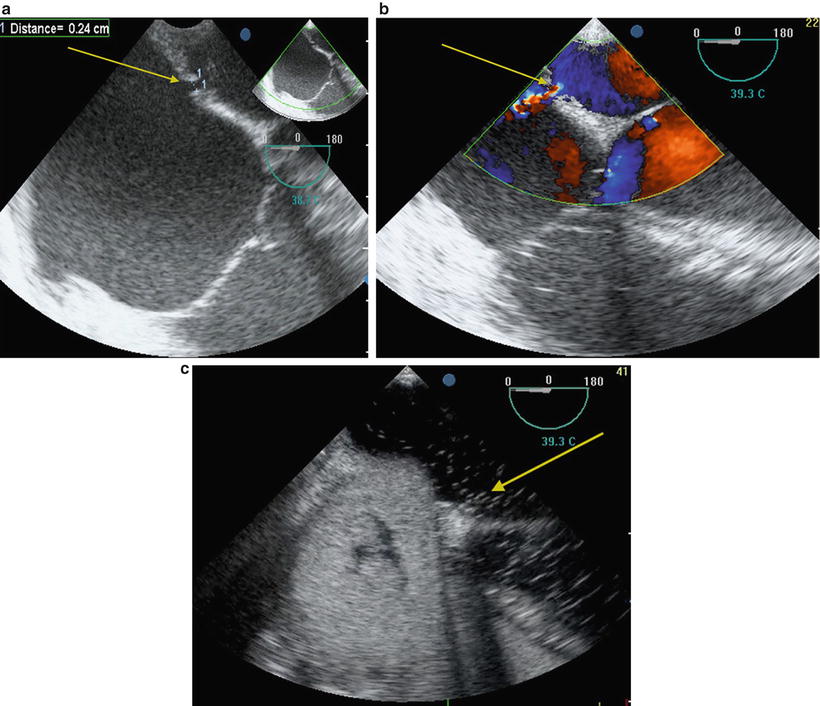
Fig. 9.2
Echocardiographic demonstration of intracardiac shunt in a patient undergoing double lung transplantation . (a) Two-dimensional echocardiography showing atrial septal defect (arrow). (b) Color Doppler showing flow through atrial septal defect (arrow). (c) Positive bubble study with air in the left side cardiac chambers (arrow)
Table 9.2
Echocardiographic assessment of the right heart
Parameter |
Criteria |
---|---|
Right ventricular enlargement |
RV size >2/3 LV size
RV minor axis:
>42 mm at the base
>35 mm at the mid-level
RV longitudinal axis >86 mm |
Right ventricular systolic dysfunction: |
RIMP:
>0.40 by PW
>0.55 by TDI
TAPSE < 16 mm
2-D FAC < 35 %
S′ < 10 cm/s |
Tricuspid regurgitation jet max velocity |
RV systolic pressure can be used to estimate PA systolic pressure if no PAC available |
RV hypertrophy |
Free wall thickness >5 mm |
Leftward displacement of VS |
RV pressure overload
Entire cardiac cycle, but mostly in end-systole
RV volume overload
Mid- to late diastole |
Table 9.3
Echocardiographic criteria for SEVERE tricuspid regurgitation
Tricuspid valve: Abnormal/flail leaflet/poor coaptation |
RV/RA/IVC usually dilated if chronic TR
RV, at end-diastole:
2/3 LV size
RV minor axis: >42 mm at the base, >35 mm at the mid-level
RV longitudinal axis >86 mm
RA, at end-diastole:
Diameter or minor axis >44 mm
Length or major axis >55 mm
IVC > 21 mm |
Jet area in central/non-eccentric jets on CFD: >10 cm2 |
Vena contracta width >0.7 cm |
PISA radius >0.9 cm
Using baseline shift with the Nyquist limit at 28 cm/s |
Jet signal density and contour on CW: Dense, triangular with early peaking |
Hepatic vein systolic flow reversal |
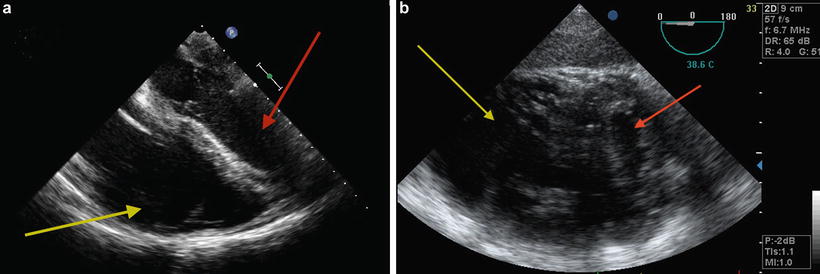
Fig. 9.3
TEE showing dilated RV with underfilled LV suggestive of RV dysfunction. (a) Midesophageal four chamber view. (b) Transgastric short-axis view; note flattening of the interventricular septum. Red arrow indicates LV and yellow arrow indicates RV
Patent foramen ovale (PFO) is a common finding that may result in intracardiac shunting and hypoxemia (Fig. 9.4). However, no definitive evidence is available to advise whether concomitant surgical closure is indicated at the time of lung transplant [7].
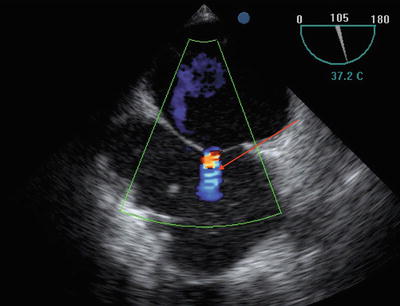
Fig. 9.4
Midesophageal bicaval view showing patent foramen ovale (arrow)
Similarly, no consensus exists on the surgical management of TR at the time of lung transplant. Frequently, no intervention is performed on the tricuspid valve, yet TR severity decreases in the post-transplant period due to a decrease in pulmonary vascular resistance (Fig. 9.5a, b) [7].
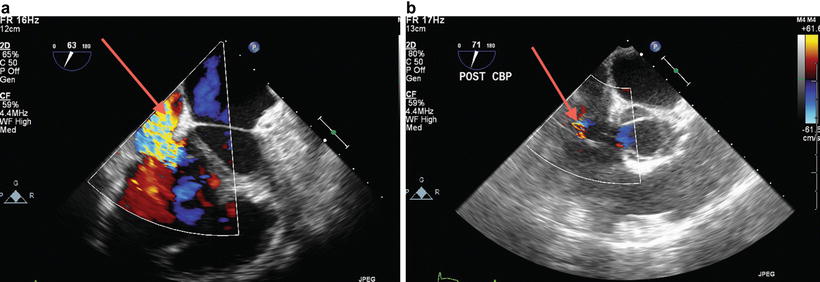
Fig. 9.5
Severe tricuspid regurgitation (TR) in a patient with end-stage lung disease and severe pulmonary hypertension. Severe prebypass TR (a) became mild (b) after new lung implantation with decreased pulmonary vascular resistance
All four pulmonary veins (PVs) should be identified by two-dimensional (2D) and color flow Doppler and the normal biphasic systolic-diastolic spectral Doppler flow pattern should be confirmed. Anatomic variation in PV drainage is common with the typical arrangement occurring in 60 % of the population, a right trifurcation pattern in 20 %, a right common pattern in 8 %, and a left trifurcation pattern in 8 % [8]. Scanning for aortic atheroma (Fig. 9.6) is also important, should the patient require cannulation for extracorporeal support.
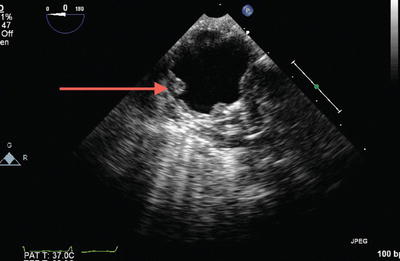
Fig. 9.6
Severe atheromatous disease of the aorta
TEE is also useful in cases of difficult PA catheter placement and to avoid too distal placement in the right or left PA that could increase risk of PA rupture or allow the catheter to become ensnared or damaged during pneumonectomy (Fig. 9.7).
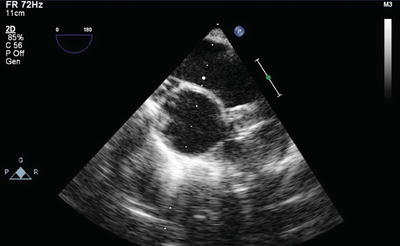
Fig. 9.7
Pulmonary artery catheter tip position (arrow) confirmed in the main pulmonary artery by TEE
Pneumonectomy: Anesthesia Considerations
The pneumonectomy phase requires attentive anesthetic management, particularly in cases of LTx performed off-pump. As discussed previously, in bilateral sequential LTx the lung with the least perfusion on the V/Q scan is transplanted first. One lung ventilation (OLV) is initiated of the contralateral lung allowing deflation of the operative lung and the hilar dissection is performed. If extensive adhesions are encountered (e.g. due to previous thoracic surgery, pleural disease, or bronchiectasis) the dissection may be lengthy and associated with significant bleeding or lung injury. Hypertrophy of the bronchial circulation seen in many LTx recipients may also predispose to hemorrhage. Close communication with the surgeon is necessary as surgical manipulation of mediastinal structures results in hemodynamic fluctuations. Surgical exposure of the left hilum is often more difficult because of the heart requiring more retraction and compression [9]; when manipulations cause significant hypotension the surgeon should be alerted so that he/she may modify technique or intermittently cease manipulation allowing hemodynamics to stabilize. Fluids, blood products, inotropes, pulmonary vasodilators, and vasopressors should be administered as indicated to maintain cardiac output and blood pressure, allowing surgical progress to continue.
Intraoperative hypoxemia can significantly impede surgical progress. Refractory hypoxemia during OLV can be ameliorated by PA occlusion, which reduces shunt fraction through the nonventilated lung. Test occlusion of the PA is first performed under close monitoring for hemodynamic deterioration, changes in PA pressures, and signs of RV dilation or hypokinesis on TEE. If test occlusion is tolerated the procedure can often proceed safely without the need for extracorporeal support. If there is a significant rise in PA pressure but no deterioration in RV function, pharmacologic treatment with inhaled pulmonary vasodilators (nitric oxide (NO) or prostacyclin) may be administered to reduce PVR and off-pump transplantation may be attempted without extracorporeal support [10–12]. In contrast, if occlusion causes RV dilatation and dysfunction, the decision is made to support the circulation with ECMO or CPB. Next, the PA and veins are ligated and divided. Following this, the main bronchus is stapled and divided. Subsequently, the native lung is explanted and the hilar structures are prepared for allograft implantation.
During pneumonectomy and particularly incision of the bronchus, the anesthesiologist and should pay close attention to the heightened risk of airway fires with the use of electrocautery (Fig. 9.8a, b) [13]. Three components are necessary for a fire to occur: (1) fuel (e.g dry laparotomy sponge, polyvinylchloride endotracheal tube (ETT), or alcohol-based skin antiseptics), (2) an oxidizer enriched environment (oxygen or nitrous oxide) and (3) an ignition source (electrosurgical cautery or laser). Precautions should be taken to avoid chest cavity and airway fire, as this can result in significant mortality and morbidity (Table 9.4). In the event of a fire, oxygen should be immediately discontinued, saline instilled into the chest, and, if involved, the ETT should be removed and replaced. Bronchoscopic examination of the airway is required at this time to evaluate the extent of injury.
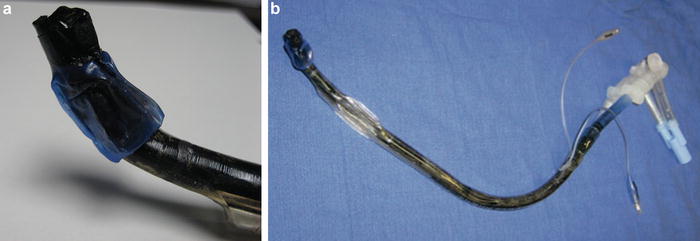
Fig. 9.8
(a, b) Airway fire during lung transplantation leading to complete destruction and charring of the double lumen endotracheal tube
Table 9.4
Prevention of surgical fires during lung transplantation
Preparation
• Lung transplant surgery represents a high-risk situation; all team members should be vigilant to avoid the use of an ignition source (electrocautery) in proximity to an oxidizer (oxygen) and fuel source (endotracheal tube, drapes, sponges)
• All personnel in theater should have predetermined and rehearsed actions to follow in the event of an airway fire according to institutional protocol, which should preferably be displayed in the operating theater
Prevention
• The surgeon should be advised against using electrocautery to enter the airways
• Delivered oxygen concentration should be reduced to the minimum required to avoid hypoxia. During off pump double-lung transplantation, the ipsilateral pulmonary artery may need to be snared down to improve arterial oxygen saturation by eliminating the intrapulmonary shunt through the nonventilated lung
• Wait a few minutes after reducing the oxygen concentration before approving the use of an ignition source
• Ensure that there is no air leak from around the ETT cuff into the operative field
• Continuous suction may be applied to the nonventilated lung prior to dividing the bronchus in order to scavenge excess oxygen
• The operating field may be flooded with carbon dioxide to minimize the oxidizer concentration if use of electrocautery is necessary to achieve hemostasis
• Avoid dry sponges when cauterizing near the airway |
Extracorporeal Support
Extracorporeal cardiopulmonary support (including CPB or extracorporeal membrane oxygenation (ECMO)) may be necessary in the perioperative period. Preoperative ECMO support with a veno-venous (VV) or veno-arterial (VA) configuration is used increasingly as a bridge-to-transplant [13].
Intraoperative extracorporeal support may become necessary at several points during LTx: (1) for a concomitant cardiac surgical procedure (such as closure of atrial septal defect or coronary artery bypass grafting), (2) continuation of preexisting ECMO or conversion to another modality, (3) electively in patients with severe pulmonary hypertension, (4) due to refractory hypoxemia or acidosis during OLV for pneumonectomy, (5) hemodynamic instability, usually following anesthetic induction or related to RV failure at the time of PA occlusion, and (6) to support oxygenation after lung implantation when graft function is poor. If CPB is used to facilitate LTx, the heart is kept warm and beating unless cardioplegia is required for a cardiac repair.
Intraoperative support during LTx has historically been provided with CPB but in recent years VA ECMO has emerged as the method of choice in some centers including the University of Pittsburgh. Observational studies report improved outcomes using intraoperatitve ECMO relative to matched patients who received CPB [14, 15]. Compared to CPB, ECMO uses a smaller circuit with reduced priming volume and lacks both a venous reservoir and cardiotomy suction resulting in no air-blood contact, reduced heparin requirements, less hemodilution, and less severe activation of the systemic inflammatory response. ECMO also has the added versatility of preoperative application as a bridge-to-transplant or postoperative bridge-to-recovery in patients with severe primary graft dysfunction.
Cannulation sites for VA ECMO may be peripheral (femoral, internal jugular, or axillary vein to femoral or axillary artery) or central (right atrium to aorta). TEE is useful for confirming guidewire placement during cannulation and for confirming appropriate cannula position for both ECMO and CPB modalities. A detailed description of perfusion management for LTx is described in elsewhere in this text.
During ECMO support, anesthesia management is directed at maintaining adequate intravascular volume as no venous reservoir is present, and monitoring for LV overdistension and continuing inotropic therapies to ensure LV ejection across the aortic valve and decrease risk of thrombus formation. TEE is helpful in troubleshooting low ECMO flows and can quickly differentiate between hypovolemia and poor venous drainage from cannula malposition, both of which are common during LTx. Note also that VA ECMO should prompt conversion to total IV anesthesia due to limited pulmonary blood flow and uptake of inhalation anesthetics.
Hemodynamic Support and Fluid Therapy
Hemodynamic instability during LTx is multifactorial and can be related to fluid deficits, blood loss, RV dysfunction, cardiac manipulation, and vasoplegia. Anesthesiologists must use clinical judgment to appropriately intervene during the surgical procedure, considering data obtained from observation of the surgical field, real-time monitors (e.g. mean arterial pressure, SaO2, central venous pressure, PA pressure, SvO2) and laboratory tests (e.g. pH, hemoglobin (Hb), PaO2, lactate, base excess) to inform decisions. Until further research in this patient population defines specific target parameters, goal-directed hemodynamic therapy with fluids, blood products, and inotropic and/or vasoactive medications should seek to optimize cardiac output, filling pressures, SvO2, and arterial blood gas values.
Commonly-used vasopressors include norepinephrine and vasopressin. Vasopressin may be preferable in patients with pulmonary hypertension because it does not constrict the pulmonary vasculature (in contrast to norepinephrine and other phenylephrine) and may in fact reduce PVR via endothelial release of NO [16]. Milrinone and epinephrine are the primary inotropes used in the authors’ practice, often in combination. Milrinone has the advantage of reducing PVR and RV afterload but at the cost of a high incidence of systemic hypotension, particularly when a loading dose is given. Vasopressin again may have advantages in the setting of milrinone-related hypotension because of a more selective action on systemic vessels and more favorable effect on the PVR/SVR ratio [17].
Hemodynamic swings are especially challenging in LTx performed off-pump without the support of ECMO or CPB. In these circumstances, SvO2 is a good monitor of the overall adequacy of systemic oxygen delivery, with persistent low SvO2 (below 65 %) indicating the possible need to convert to an on-pump approach. Left-sided implantation can be more hemodynamically challenging than right-sided surgery. Partial clamping of the left atrium (LA) during anastomosis of the atrial cuff frequently causes hypotension, which is best treated by adjustment of the clamp rather than overzealous fluid administration.
Fluid management is complicated by the lack of a validated intraoperative monitor of optimized fluid status and the susceptibility of the newly reimplanted allograft to the development of pulmonary edema due to increased vascular permeability [18] and lack of lymphatic drainage. Multiple observational studies have correlated liberal fluid administration with acute lung injury (ALI) after pneumonectomy [19] and pulmonary resection for cancer [20, 21]. Based on the above small series, some recommend restricting intraoperative fluid to 2000 mL or less [22]. Both colloids and crystalloids have been implicated in the genesis of ALI. In a retrospective analysis of LTx patients, increased intraoperative fluid infusion correlated with poorer oxygenation and reduced extubation rates [23].
Intraoperative red cell transfusion is aimed optimizing oxygen delivery to the tissues and therefore should be based on objective evidence of oxygen debt such as SvO2, arterial base deficit or lactate rather than hemoglobin triggers, although transfusion is generally indicated below a hemoglobin concentration of about 8 g/dL in the intraoperative setting. Massive transfusion may be required if there is extensive bleeding due to adhesive disease or surgical injury to the PA and the potential for severe coagulopathy exists. Patients with preoperative or intraoperative anticoagulation requirements because of extracorporeal support may also be at risk for increased bleeding. More evidence is needed to define the predictors of transfusion and transfusion-related outcomes in the LTx patient population. Cell salvage should be utilized as part of a blood conservation strategy unless a contraindication exists.
Mechanical Ventilation
Induction of anesthesia, muscle paralysis, positive pressure ventilation, and supine position can all induce changes in functional residual capacity and negatively impact gas exchange. Ventilation strategies for the diseased lungs preimplantation may vary compared to post-implantation ventilation of the graft lungs. For example, principles of high PEEP, low tidal volume lung-protective ventilation may not be feasible during one lung ventilation in patients with end-stage lung disease undergoing double lung transplant since the primary goal in this setting is to maintain adequate oxygenation and avoid severe respiratory acidosis until graft implantation, at which time ventilator settings can be adjusted. The following is a description of general ventilation strategies useful in the two major groups of LTx recipients, those with chronic obstructive lung disease and those with restrictive lung diseases such as pulmonary fibrosis.
Chronic obstructive pulmonary disease (COPD) including alpha-1 antitrypsin deficiency is the most common indication for adult LTx. Due to expiratory airflow obstruction there is gas trapping which can be progressive during positive pressure ventilation leading to dynamic hyperinflation and intrinsic positive end-expiratory pressure (PEEP) , also known as auto-PEEP . Some degree of auto-PEEP is common during one lung ventilation, even in the absence of significant COPD [24, 25], and, if excessive, can lead to decreased cardiac preload, hypotension, and even cardiac arrest. The pressure- and flow-versus time displays on modern anesthesia ventilators can be used to readily identify auto-PEEP, showing nonzero end-expiratory flow and pressure. This entity should be considered in any LTx patient with hemodynamic deterioration as the treatment is unique (disconnect the circuit for a period of seconds until airway pressure decreases to zero) and failure to treat this problem will impede any other attempts at resuscitation.
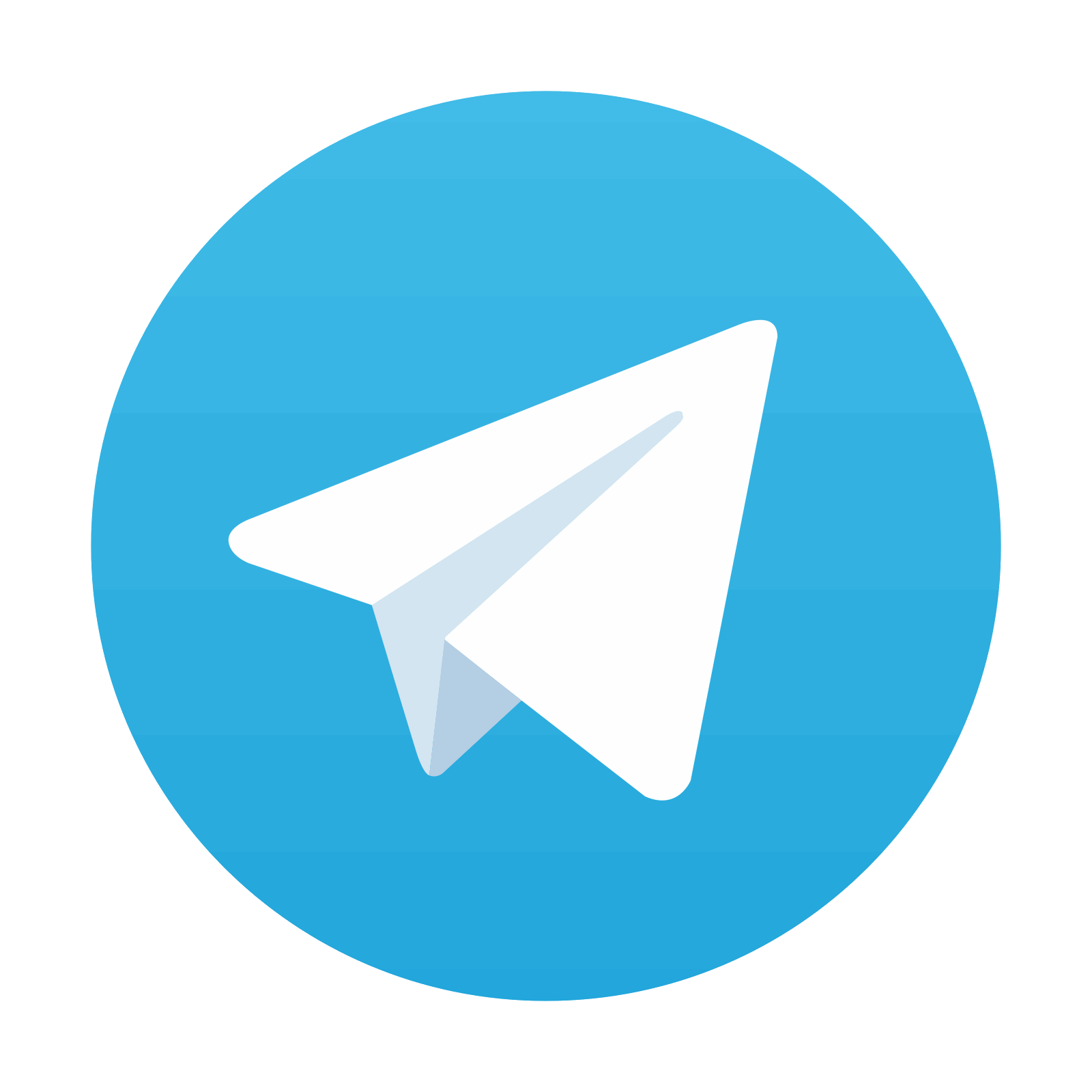
Stay updated, free articles. Join our Telegram channel

Full access? Get Clinical Tree
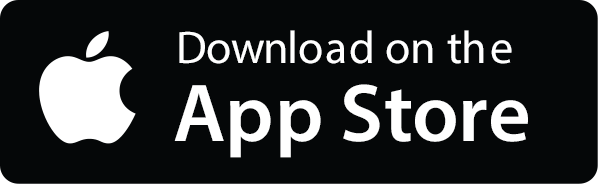
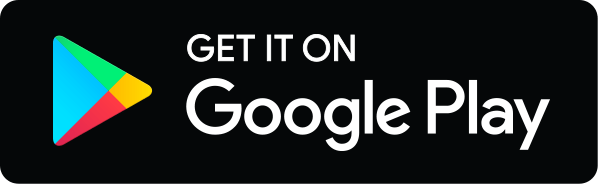