Chapter Outline
VAPOR, EVAPORATION, AND VAPOR PRESSURE
Measurement of Vapor Pressure and Saturated Vapor Pressure
Dalton’s Law of Partial Pressures
Minimum Alveolar Concentration
Regulating Vaporizer Output: Variable Bypass Versus Measured Flow
Efficiency and Temperature Compensation
Incorrect Filling of Vaporizers
Vaporization of Mixed Anesthetic Liquids
Effect of Carrier Gas on Vaporizer Output
Effects of Changes in Barometric Pressure
Vaporizing Chamber Flow Controlled at Inlet
Vaporizer Chamber Flow Controlled at Outlet
Calibration and Checking of Vaporizer Outputs
EFFECT OF USE VARIABLES ON VAPORIZER FUNCTION
General Principles
The term vapor describes the gaseous phase of a substance at a temperature at which the substance can exist in either a liquid or solid state below a critical temperature for that substance. If the vapor is in contact with a liquid phase, the two phases will be in a state of equilibrium, and the gas pressure will equal the equilibrium vapor pressure of the liquid. The potent inhaled volatile anesthetic agents—halothane, enflurane, isoflurane, sevoflurane, and desflurane—are mostly in the liquid state at normal room temperature (20° C) and atmospheric pressure (760 mm Hg). Anesthesia vaporizers are devices that facilitate the change of a liquid anesthetic into its vapor phase and add a controlled amount of this vapor to the flow of gases entering the patient’s breathing circuit.
The anesthesia care provider should be familiar with the principles of vaporization of the potent inhaled anesthetic agents and their application in both the construction and use of anesthesia vaporizers designed to be placed in the low-pressure system of the anesthesia machine—that is, the fresh gas flow circuit downstream of the gas flow control valves. The 1989 voluntary consensus standard for anesthesia machines (American Society for Testing and Materials [ASTM] F1161-88) required that all vaporizers located within the fresh gas circuit be concentration calibrated and that control of the vapor concentration be provided by calibrated knobs or dials. The most recent standard, ASTM 1850-00, maintains these requirements.
Measured flow systems are not mentioned in the 1989 and subsequent ASTM standards and are therefore considered obsolete as defined in the American Society of Anesthesiologists (ASA) 2004 statement on determining anesthesia machine obsolescence. Despite their obsolescent status, the principles of measured flow vaporizing systems are briefly discussed in this chapter because they provide a basis for understanding the contemporary concentration-calibrated, variable bypass vaporizers used to deliver isoflurane, enflurane, halothane, and sevoflurane.
Desflurane has certain physical properties that preclude its delivery by a conventional variable bypass vaporizer and is therefore discussed in a separate section. The most recently introduced Aladin vaporizing system (GE Healthcare, Waukesha, WI) is a hybrid of the measured flow and variable bypass designs. The Aladin system can accurately deliver desflurane and the other less volatile potent anesthetic agents.
Vapor, Evaporation, and Vapor Pressure
When placed in a closed container at normal atmospheric pressure and room temperature (given above), a potent inhaled anesthetic is in liquid form. Some anesthetic molecules escape from the surface of the liquid to enter the space above as a gas or vapor. At constant temperature, an equilibrium is established between the molecules in the vapor phase and those in the liquid phase. The molecules in the vapor phase are in constant motion, bombarding the walls of the container to exert vapor pressure. An increase in temperature causes more anesthetic molecules to enter the vapor phase—that is, to evaporate; this results in an increase in vapor pressure. The gas phase above the liquid is said to be saturated when it contains all the anesthetic vapor it can hold at a given temperature, at which time the pressure exerted by the vapor is referred to as its saturated vapor pressure (SVP) at that temperature.
Measurement of Vapor Pressure and Saturated Vapor Pressure
The following description is intended to provide an understanding of how, in principle, the SVP of a potent inhaled volatile anesthetic agent could be measured in a simple laboratory experiment and demonstrate the pressure that a vapor can exert. Figure 3-1 , A, shows a simple (Fortin) barometer, which is essentially a long, glass mercury-filled test tube inverted to stand with its mouth immersed in a trough of mercury. When the barometer tube is first made vertical, the mercury column in the tube falls to a certain level, leaving a so-called Torricellian vacuum above the mercury meniscus. In this system, the pressure at the surface of the mercury in the trough is due to the atmosphere. In a communicating system of liquids, the pressures at any given depth are equal; therefore the pressure at the surface of the mercury in the trough is equal to the pressure exerted by the column of mercury in the vertical tube. In this example, atmospheric pressure is said to be equivalent to 760 mm Hg, because this is the height of the column of mercury in the barometer tube.

In Figure 3-1 , B, sevoflurane liquid is introduced at the bottom of the mercury column. Being less dense than mercury, it rises to the top and evaporates into the space created by the Torricellian vacuum. The sevoflurane vapor exerts pressure and causes an equivalent decrease in the height of the mercury column. If liquid sevoflurane is added until a small amount remains unevaporated on the top of the mercury meniscus ( Fig. 3-1 , C ), the space above the column must be fully saturated with vapor; the pressure now exerted by the vapor is the SVP of sevoflurane at that temperature, and adding more liquid sevoflurane will not affect the vapor pressure. If this experiment is repeated at different temperatures, a graph can be constructed that plots SVP against temperature. Such curves for some of the potent inhaled volatile anesthetic agents are shown in Figure 3-2 . Contemporary technologies for measuring the partial pressures or SVPs of gases and vapors are described in Chapter 8 .

Boiling Point
The SVP exerted by the vapor phase of a potent inhaled volatile agent is a physical property of that agent and depends only on the agent and the ambient temperature. The temperature at which SVP becomes equal to ambient (atmospheric) pressure and that at which all the liquid agent changes to the vapor phase (i.e., evaporates) is the boiling point of that liquid. Water boils at 100° C at 1 atm because at 100° C, the SVP of water is 760 mm Hg. The most volatile of the agents are those with the highest SVPs at room temperature. At any given temperature, these agents also have the lowest boiling points: desflurane and diethyl ether boil at 22.9° C and 35° C, respectively, at an ambient pressure of 760 mm Hg. Boiling point decreases with decreasing ambient barometric pressure, such as occurs at increasing altitude.
Units of Vapor Concentration
The presence of anesthetic vapor may be quantified either as an absolute pressure, expressed in millimeters of mercury (mm Hg) (or, less commonly, kilopascals [kPa]) or in volumes percent (vol%) of the total atmosphere (i.e., volumes of vapor per 100 volumes of total gas). From Dalton’s law of partial pressures, the volumes percent can be calculated as the fractional partial pressure of the agent:
v ol % = Partial pressure from vapor Total ambient pressure × 100 %
Dalton’s Law of Partial Pressures
Dalton’s law states that the pressure exerted by a mixture of gases, or gases and vapors, enclosed in a given space such as a container is equal to the sum of the pressures that each gas or vapor would exert if it alone occupied that given space or container. A gas or vapor exerts its pressure independently of the pressure of the other gases present. For example, in a container of dry air at 1 atm (760 mm Hg), with oxygen representing 21% of all gases present, the pressure exerted by the oxygen—its partial pressure—is 159.6 mm Hg (21% × 760). Consider the same air at a pressure of 760 mm Hg but fully saturated with water vapor at 37° C (normal body temperature). Because vapor pressure depends on temperature, the SVP for water at 37° C is 47 mm Hg. The pressure from oxygen is therefore now 21% of 713 (i.e., 760 − 47) mm Hg. The partial pressure of oxygen is therefore 149.7 mm Hg.
Note that volumes percent expresses the relative ratio or proportion (%) of gas molecules in a mixture, whereas partial pressure (mm Hg) represents an absolute value. Anesthetic uptake and potency are directly related to partial pressure and only indirectly to volumes percent. This distinction become more apparent when hyperbaric and hypobaric conditions are considered.
Minimum Alveolar Concentration
The minimum alveolar concentration (MAC) of a potent inhaled anesthetic agent is the concentration that produces immobility in 50% of patients who undergo a standard surgical stimulus. Used as a measure of anesthetic potency or depth, MAC is commonly expressed as volumes percent of alveolar (end-tidal) gas at 1 atm pressure at sea level (i.e., 760 mm Hg). Table 3-1 shows how MAC expressed in familiar volumes percent can be expressed as a partial pressure in millimeters of mercury. Anesthesiologists should learn to think of MAC in terms of partial pressure rather than in terms of volumes percent because the partial pressure (tension) of the anesthetic in the central nervous system is responsible for the depth of anesthesia. This concept has been advocated by Fink, who proposed the term minimum alveolar pressure (MAP), and by James and White, who suggested minimum alveolar partial pressure (MAPP). In this text, the term P MAC1 (see Table 3-1 ) is used to express the partial pressure of a potent inhaled agent at a concentration of 1 MAC; thus 1 MAC of isoflurane is equivalent to a P MAC1 of 8.7 mm Hg.
Agent | MAC (vol%) | P MAC1 (mm Hg) |
---|---|---|
Halothane | 0.75 × 760 | 5.7 |
Enflurane | 1.68 × 760 | 12.8 |
Isoflurane | 1.15 × 760 | 8.7 |
Methoxyflurane | 0.16 × 760 | 1.2 |
Sevoflurane | 2.10 × 760 | 16 |
Desflurane | 7.25 × 760 | 55 |
Latent Heat of Vaporization
Vaporization requires energy to transform molecules from the liquid phase to the vapor phase. This energy is called the latent heat of vaporization and is defined as the amount of heat (calories) required to convert a unit mass (grams) of liquid into vapor. For example, at 20° C the latent heat of vaporization of isoflurane is 41 cal/g. The heat of vaporization is inversely related to ambient temperature in such a way that at lower temperatures, more heat is required for vaporization. The heat required to vaporize an anesthetic agent is drawn from the remaining liquid agent and from the surroundings. As vapor is generated and heat energy is lost, the temperatures of the vaporizer and the liquid agent fall. This causes the vapor pressure of the anesthetic to decrease. If no compensatory mechanism is provided, this will result in decreased output of vapor.
Specific Heat
Specific heat is the quantity of heat (calories) required to raise the temperature of a unit mass (grams) of a substance by 1° C. Heat must be supplied to the liquid anesthetic in the vaporizer to maintain the liquid’s temperature during the evaporation process, when heat is being lost.
Specific heat is also important when it comes to vaporizer construction material. For the same amount of heat lost through vaporization, temperature changes are more gradual for materials with a high specific heat than for those with a low specific heat. Thermal capacity, defined as the product of specific heat and mass, represents the quantity of heat stored in the vaporizer body.
Also of importance is the construction material’s ability to conduct heat from the environment to the liquid anesthetic. This property is called thermal conductivity, defined as the rate at which heat is transmitted through a substance. For the liquid anesthetic to remain at a relatively constant temperature, the vaporizer is constructed from materials that have a high specific heat and high thermal conductivity. In this respect, copper comes close to the ideal; however, bronze and stainless steel have been used more recently in vaporizer construction.
Regulating Vaporizer Output: Variable Bypass Versus Measured Flow
The SVPs of halothane, sevoflurane, and isoflurane at room temperature are 243, 160, and 241 mm Hg, respectively. Dividing the SVP by ambient pressure (760 mm Hg) gives the saturated vapor concentration (SVC) as a fraction (or percentage) of 1 atm. This is an application of Dalton’s law, as discussed earlier. The SVCs of halothane, sevoflurane, and isoflurane are therefore 32%, 21%, and 31%, respectively. These concentrations are far in excess of those required clinically ( Table 3-2 ). Therefore the vaporizer first creates a saturated vapor in equilibrium with the liquid agent; second, the saturated vapor is diluted by a bypass gas flow. This results in clinically safe and useful concentrations flowing to the patient’s breathing circuit. Without this dilution of saturated vapor, the agent would be delivered in a lethal concentration to the anesthesia circuit.
Agents | Halothane | Enflurane | Isoflurane | Methoxyflurane | Sevoflurane | Desflurane |
---|---|---|---|---|---|---|
Structure | CHBrClCF 3 | CHFClCF 2 OCHF 2 | CF 2 HOCHClCF 3 | CHCl 2 CF 2 OCH 3 | CH 2 FOCH(CF 3 ) 2 | CF 2 HOCFHCF 3 |
Molecular weight (AMU) | 197.4 | 184.5 | 184.5 | 165.0 | 200 | 168 |
Boiling point at 760 mm Hg (° C) | 50.2 | 56.5 | 48.5 | 104.7 | 58.5 | 22.8 |
SVP at 20° C (mm Hg) | 243 | 175 | 238 | 20.3 | 160 | 664 |
SV conc. at 20° C and 1 ATA ∗ (vol%) | 32 | 23 | 31 | 2.7 | 21 | 87 |
MAC at 1 ATA ∗ (vol%) | 0.75 | 1.68 | 1.15 | 0.16 | 2.10 | 6-7.25 † |
P MAC1 (mm Hg) | 5.7 | 12.8 | 8.7 | 1.22 | 16 | 46-55 † |
Specific gravity of liquid at 20° C | 1.86 | 1.52 | 1.50 | 1.41 | 1.51 | 1.45 |
mL vapor per gram liquid at 20° C | 123 | 130 | 130 | 145 | 120 | 143 |
mL vapor per mL liquid at 20° C | 226 | 196 | 195 | 204 | 182 | 207 |
∗ 1 ATA = one atmosphere absolute pressure (760 mm Hg).
Contemporary anesthesia vaporizers are concentration calibrated, and most are of the variable bypass design. In a variable bypass vaporizer, such as those made by GE Healthcare (Tec series) and the Dräger Vapor 2000 (Dräger Medical, Telford, PA), the total fresh gas flow from the anesthesia machine flowmeters passes to the vaporizer ( Fig. 3-3 ). The vaporizer splits the incoming gas flow between two pathways: the smaller flow enters the vaporizing chamber, or sump, of the vaporizer and leaves it with the anesthetic agent at its SVC. The larger bypass flow is eventually mixed with the outflow from the vaporizing chamber to create the desired, or “dialed in,” concentration (see Fig. 3-3 ).

In the now-obsolete measured flow, non-concentration-calibrated vaporizers such as the Copper Kettle (Puritan-Bennett; Covidien, Mansfield, MA) or Verni-Trol (Ohio Medical Products, Gurnee, IL), a measured flow of oxygen is set on a separate flowmeter to pass to the vaporizer, from which vapor emerges at its SVP ( Fig. 3-4 ). This flow is then diluted by an additional measured flow of gases (oxygen, nitrous oxide, air, etc.) from the main flowmeters on the anesthesia machine. With this type of arrangement, calculations are necessary to determine the anesthetic vapor concentration in the emerging gas mixture.

With both types of vaporizing systems, there must be an efficient method to create a saturated vapor in the vaporizing chamber. This is achieved by having a large surface area for evaporation. Flow-over vaporizers (Dräger Vapor 2000 series, GE Tec series) increase the surface area using wicks and baffles. In measured flow, bubble-through vaporizers, oxygen is bubbled through the liquid agent. To increase the surface area, tiny bubbles are created by passing the oxygen through a sintered bronze disk in the Copper Kettle, for example, which created large areas of liquid/gas interface, over which evaporation of the liquid agent could quickly occur.
Calculation of Vaporizer Output
At a constant room temperature of 20° C, the SVPs of two commonly used potent inhaled agents are 160 mm Hg for sevoflurane and 238 mm Hg for isoflurane. If ambient pressure is 760 mm Hg, these SVPs represent 21% sevoflurane (160/760) and 31% isoflurane (239/760), each in terms of volumes percent of 1 atm (760 mm Hg).
A concept fundamental to understanding vaporizer function is that under steady-state conditions, if a certain volume of carrier gas flows into a vaporizing chamber over a certain period, that same volume of carrier gas exits the chamber over the same period. However, because of the addition of vaporized anesthetic agent, the total volume exiting the chamber is greater than that entering it. In the vaporizing chamber, anesthetic vapor at its SVP constitutes a mandatory fractional volume of the atmosphere (i.e., 21% in a sevoflurane vaporizer at 20° C and 760 mm Hg). Therefore the volume of carrier gas will constitute the difference between 100% of the atmosphere in the vaporizing chamber and that resulting from the anesthetic vapor. In the case of sevoflurane, the carrier gas represents 79% of the atmosphere in the vaporizing chamber at any time. Thus if 100 mL/min of carrier gas flows through a vaporizing chamber containing sevoflurane, the carrier gas represents 79% (100% − 21%) of the atmosphere and the remaining 21% is sevoflurane vapor. By simple proportions, the volume of sevoflurane vapor exiting the chamber can be calculated to be 27 mL ([100/79] × 21) when rounded to the nearest whole number.
In other words, if 100 mL/min of carrier gas flows into the vaporizing chamber, the same 100 mL of carrier gas will emerge together with 27 mL/min of sevoflurane vapor. Another way of expressing this is shown below:
SVP agent ( mm Hg ) Total pressure ( mm Hg ) = Agent vapor ( x mL ) Carrier gas ( y mL ) + Agent vapor ( x mL ) = Volume of agent vapor Total volume leaving vaporizer
Continuing the above example for sevoflurane, y is 100 mL/min, and 160/760 = x /(100 + x ), from which x can be calculated as 27 mL (rounded to the nearest whole number). Conversely, if x is known, the carrier gas flow y can be calculated. At steady state, the total volume of gas leaving the vaporizing chamber is greater than the total volume that entered, the additional volume being anesthetic vapor at its SVC.
Vaporizer Function
Measured Flow Vaporizers
Although measured flow vaporizers are not mentioned in the ASTM anesthesia machine standards published after 1988, it is helpful to review the function of one example, the Copper Kettle. If 1% (vol/vol) isoflurane must be delivered to the patient circuit at a total fresh gas flow rate of 5 L/min ( Fig. 3-5 ), the vaporizer must evolve 50 mL/min of sevoflurane vapor (1% × 5000 mL) to be diluted in a total volume of 5000 mL.

In the Copper Kettle, isoflurane represents 31% of the atmosphere, assuming a constant temperature of 20° C and a constant SVP of 238 mm Hg. If 50 mL of isoflurane vapor represents 31%, the carrier gas flow ( x mL) of, oxygen flow x must represent the other 69% (100% − 31%). Thus
50 31 = x 69
31 x = 50 × 69
x = ( 50 × 69 ) 31 = 111 mL
Therefore, if 111 mL/min of oxygen is bubbled through liquid isoflurane in a Copper Kettle vaporizer, 161 mL/min of gas emerges, of which 50 mL is isoflurane vapor and 111 mL is the oxygen that flowed into the vaporizer. This vaporizer output of 161 mL/min must be diluted by an additional fresh gas flow of 4839 mL/min (5000 mL − 161 mL) to create an isoflurane mixture of exactly 1% because 50 mL of isoflurane vapor diluted in a total volume of 5000 mL gives 1% isoflurane by volume.
Although this situation is highly unlikely to occur in contemporary practice because of the obsolescence of measured flow vaporizers, if a measured flow system had to be used to deliver isoflurane, the anesthesia provider would likely set flows of 100 mL/min oxygen to the Copper Kettle and 5 L/min of fresh gas on the main flowmeters, which would result in only slightly less than 1% isoflurane (44.9/5044.9 = 0.89%). Multiples of either of the vaporizer oxygen flow and main gas flowmeter flows would be used to create other concentrations of isoflurane from the Copper Kettle. Thus a 200 mL/min oxygen flow to the vaporizer and 5000 mL/min on the main flowmeters would create approximately 1.8% isoflurane. It is important to realize that if there is oxygen flow only to the Copper Kettle vaporizer and no bypass gas flow is set on the main machine flowmeters, lethal concentrations approaching 31% isoflurane would be delivered to the anesthesia circuit, albeit at low flow rates.
Because halothane and isoflurane have similar SVPs at 20° C, the Copper Kettle flows to be set for halothane would be essentially the same as those for isoflurane when a 1% concentration of isoflurane is to be created with a Copper Kettle. A Copper Kettle arrangement on an older model anesthesia machine is shown in Figure 3-6 .

Because enflurane and sevoflurane have similar vapor pressures at 20° C (175 mm Hg and 160 mm Hg, respectively), similar flow settings could be used to create approximately the same agent concentrations with a measured flow system. In the case of sevoflurane, the measured flow vaporizer contains 21% sevoflurane vapor (160/760 = 21%). The oxygen flow therefore represents the remaining 79% of the atmosphere in the Copper Kettle. If precisely 1% sevoflurane is required at a 5 L/min total rate of flow, 50 mL/min of sevoflurane vapor must be generated. If 50 mL represents 21% of the atmosphere in the vaporizer, the carrier gas flow required is 188 mL/min ([50/21] × 79).
If 188 mL/min of oxygen are bubbled through liquid sevoflurane contained in a measured flow vaporizer, 238 mL/min of gas will emerge, 50 mL/min of which is sevoflurane vapor. This must be diluted by a fresh gas flow of 4762 mL/min (5000 − 238) to achieve exactly 1% sevoflurane.
Alternatively, using the formula given previously:
160 760 = 50 50 + y
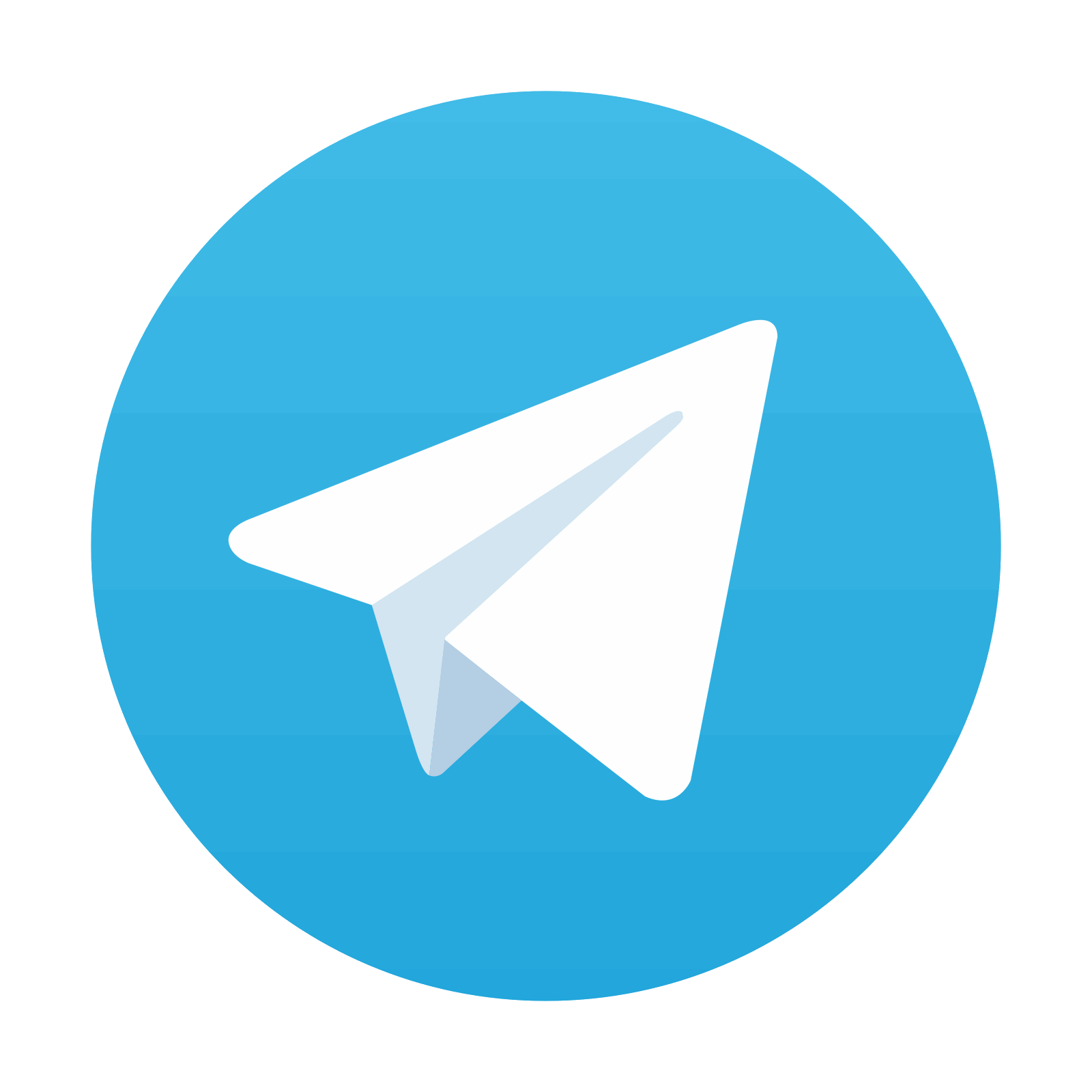
Stay updated, free articles. Join our Telegram channel

Full access? Get Clinical Tree
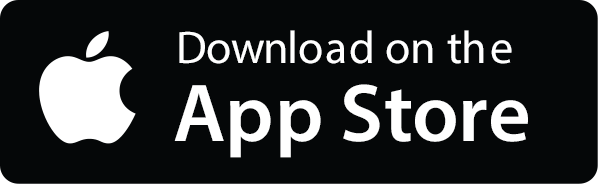
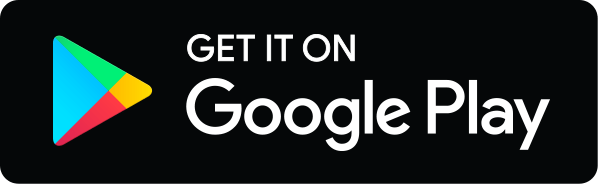