- •
The preoperative evaluation, which is performed prior to any anesthetic intervention, includes a history of present illness; past medical problems, including medication allergies; a past surgical and anesthetic history; a family history of anesthetic complications; a review of the patient’s current and possibly prior medical record, including the medication list; and a focused physical examination.
- •
General anesthesia includes a combination of medications to provide amnesia, analgesia, muscle relaxation, and attenuation of the sympathetic nervous system’s response to surgical trauma. The phases of general anesthesia include induction, maintenance, and emergence.
- •
The standards for intraoperative anesthetic monitoring have been outlined by the American Society of Anesthesiologists and include an oxygen analyzer, noninvasive blood pressure cuff, continuous electrocardiogram, pulse oximeter, end-tidal carbon dioxide analyzer, precordial or esophageal stethoscope, temperature probe, and ventilator disconnect alarm.
- •
Given the catastrophic effects of local anesthetic systemic toxicity (LAST), mechanisms to avoid it and prevent its occurrence are mandatory during the performance of regional anesthetic techniques in infants and children. Epinephrine (5 μg/mL) is added to the local anesthetic solution during performance of a regional anesthetic technique to serve as a marker of inadvertent systemic injection. Lipid emulsion should be readily available to treat LAST.
- •
Following the successful completion of the surgical procedure, a plan is determined for the postoperative delivery of analgesia. The plan should be a multimodality approach that may include intravenous opioids, agents to inhibit prostaglandin formation (acetaminophen or a nonsteroidal antiinflammatory agent), or a regional anesthetic technique. For major surgical procedures, the most effective way to deliver opioids for postoperative analgesia is patient-controlled analgesia.
Intraoperative surgical anesthesia can be provided by several different techniques, including local anesthesia, monitored anesthesia care, regional anesthesia, and general anesthesia. Local anesthesia (“local only”) involves the infiltration of a surgical site with a local anesthetic agent to render the site insensitive to pain. This may be performed solely by the surgeon without the involvement of an anesthesia provider.
Monitored anesthesia care can be thought of as being equivalent to procedural sedation. It involves monitoring a patient with standard monitors according to the American Society of Anesthesiologists (ASA; discussed later) while administering a sedative or analgesic agent intravenously to provide anxiolysis, sedation, and analgesia. Monitored anesthesia care is frequently combined with either infiltration of the surgical site with a local anesthetic agent or a regional anesthetic technique. The anesthetic agents used for monitored anesthesia care may include many of the same agents used for general anesthesia. It generally includes a medication with amnestic or anxiolytic properties (midazolam, dexmedetomidine, or propofol) combined with a medication to provide analgesia, such as an opioid (fentanyl) or, occasionally, ketamine. During the procedure, spontaneous ventilation with a native airway is maintained, eliminating the need for endotracheal intubation and controlled ventilation. The depth of sedation may range from a state in which the patient is awake and relaxed with the ability to respond to verbal stimuli to a state of deep sedation in which a painful stimulus is required to elicit a response.
Regional anesthetic techniques may include peripheral nerve blockade or neuraxial anesthesia. A peripheral nerve block involves the placement of a local anesthetic agent around a nerve or group of nerves (plexus) to render specific dermatomes insensitive to pain. Examples of plexus blockade include cervical plexus blockade for superficial and deep neck surgery, brachial plexus blockade for upper extremity or shoulder procedures, and lumbar plexus blockade for hip or leg surgery. Intravenous regional anesthesia, the Bier block, is another example of a peripheral block that can be used to provide surgical anesthesia. A Bier block involves the intravenous injection of a dilute local anesthetic into the vein of an extremity after that extremity has been exsanguinated by wrapping it with a bandage followed by occlusion with a tourniquet. The technique has been successfully and safely employed by nonanesthesia providers to allow for fracture reductions and other orthopedic procedures in the emergency department setting. , Although the latter technique is generally successful and easy to accomplish, it may not be feasible in younger children except when combined with deep sedation. Given the use of an occlusive tourniquet, its duration is limited to 60 to 70 minutes due to tourniquet pain. The major concern with the Bier block is that the amount of local anesthetic used approaches the toxic limit. In the event of tourniquet failure, cardiovascular (CV) or central nervous system (CNS) consequences from the local anesthetic agent may occur.
Neuraxial anesthesia involves the injection of a local anesthetic agent into either the subarachnoid or epidural space. This results in blockade of the spinal cord and its accompanying nerve roots to render an entire region of the body (lower abdomen, pelvis, perineum, or lower extremities) insensitive to pain. Examples of neuraxial anesthesia include spinal, epidural, and caudal anesthesia. , In infants and children, a regional anesthetic technique such as a peripheral nerve block or epidural anesthesia can be used instead of general anesthesia in patients with comorbid diseases or as a suitable alternative to general anesthesia. More frequently, the regional anesthetic technique is combined with a general anesthetic as part of a balanced anesthetic technique and continued into the postoperative period by use of a continuous infusion via a catheter to provide postoperative analgesia.
General anesthesia is the most frequently used intraoperative technique in the pediatric population. It usually includes the administration of medications to provide the requisites of amnesia, analgesia, muscle relaxation, and attenuation of the sympathetic nervous system’s response to surgical trauma. The phases of general anesthesia include induction, maintenance, and emergence. The induction of anesthesia can be carried out with the administration of an intravenous anesthetic agent (thiopental, propofol, ketamine, or etomidate) or with an inhalational (volatile) anesthetic agent such as sevoflurane. These agents may be combined with a neuromuscular blocking agent (NMBA) to facilitate endotracheal intubation or to provide surgical relaxation for specific procedures.
Advantages of an intravenous induction include the rapid onset of anesthesia and avoidance of issues associated with inhalation induction, including claustrophobia from anesthesia mask placement and the odor of the inhalational anesthetic agent. In pediatric patients, the inhalation induction of anesthesia is frequently chosen to avoid the need for obtaining intravenous access in an awake child. When inhalation induction is carried out without intravenous access, airway and CV issues may arise and mandate immediate treatment without intravenous access. In such cases, if intravenous access cannot be rapidly obtained, it may be feasible to use the intramuscular (IM) route for a select number of medications (atropine, succinylcholine). However, more aggressive resuscitation, such as the administration of epinephrine for hemodynamic compromise, may require the use of intraosseous (IO) access. The majority of problems during inhalation induction (bradycardia or laryngospasm) can be easily reversed with appropriate airway techniques or the administration of IM medications. Hemodynamic compromise, including cardiac arrest during inhalation induction, was far more common with the use of halothane given its negative inotropic and chronotropic properties. Given these and other concerns, halothane has been replaced by sevoflurane in clinical practice in most countries.
Even if intravenous access is present, the inhalational induction of anesthesia may be chosen, as it allows the maintenance of spontaneous ventilation even during deep planes of anesthesia (deep enough to allow for direct laryngoscopy and endotracheal intubation). Such a technique may be used if there is a question regarding the efficacy of bag-valve-mask ventilation, such as may occur in patients with a potentially compromised airway from infection, tumor, congenital or anatomic abnormalities.
Following anesthetic induction, one progresses into the maintenance phase of general anesthesia. This can be provided by the administration of intravenous agents, inhalational agents, or, often, a combination of the two. An example of a balanced technique includes the combination of inhalational agents (a volatile anesthetic agent) or the continuous infusion of an intravenous anesthetic agent (propofol) and an opioid. In most circumstances, the choice of maintenance anesthesia is based on the presence of comorbid features, the type of surgical procedure, and the preferences of the anesthesiologists.
Preoperative evaluation
Regardless of the type of procedure, patient’s status, and planned anesthetic technique, a preoperative evaluation is performed at some point prior to anesthetic care. This evaluation may be performed well in advance of the anticipated surgical procedure in a specialized clinic to allow for specific preoperative interventions, consultations, or preparation that may be required to allow for the safe completion of the anesthetic care and surgical procedure. Alternatively, in low-risk patients without accompanying comorbid diseases, a preoperative survey can be performed and instructions given on the phone or via the Internet using a standardized survey. This is followed by a review and physical examination by the anesthesia personnel on the day of surgery. For patients who are already admitted to the hospital or those presenting for emergent or urgent surgical procedures, the preoperative evaluation may be performed the night before or just prior to the surgical procedure.
The preoperative evaluation includes a history of present illness; review of past medical problems and hospitalizations; medication allergies; past surgical and anesthetic history; family history of anesthetic complications; and a review of the patient’s current and possibly prior medical record, including the medication list. For elective surgical procedures, the status of comorbid conditions should be optimized prior to the surgical procedure. The latter may not be feasible for urgent or emergent cases. The physical examination is directed primarily at the central nervous system, CV system, and respiratory system, including an examination of the airway. The preoperative evaluation attempts to identify patients with a difficult airway, which may preclude successful endotracheal intubation using standard techniques of direct larygnoscopy. As a full description of the airway examination is beyond the scope of this chapter, the reader is referred to Bryant et al. for additional details. An airway history should be obtained, seeking physical features as well as medical, surgical, and anesthetic factors that may indicate a difficult airway. Examination of previous anesthesia records is helpful, although a patient’s airway may change with changes in weight, with aging, or with the development of comorbid conditions. A physical examination of the airway is performed to detect physical characteristics associated with a difficult airway, such as a large tongue, small mouth, short neck (shortened thyromental distance), recessed mandible (micrognathia), limited extension or flexion of the neck, and limited mouth opening ( Table 129.1 ). The patient is then asked to open the mouth so that the uvula and tonsillar pillars can be assessed. The view of the oropharynx is assessed using the Mallampati grading system. Visualization of the entire uvula and tonsillar pillars (Mallampati grade 1) suggests that endotracheal intubation will be uncomplicated, whereas failure to visualize the tonsillar pillars and the soft palate (Mallampati class IV) suggests that endotracheal intubation will be difficult. Based on the preoperative evaluation and identification of comorbid conditions, an ASA Physical Status classification is assigned ( Table 129.2 ). The physical classification is based on the physical condition of the patient and does not include the planned surgical procedure. Laboratory tests and additional investigations are ordered based on the positive findings obtained during the history and physical examination and on the complexity of the surgical procedure. , The routine preoperative testing of all patients for elective surgery has been shown to be unjustified and expensive. In the absence of comorbid conditions, for surgical procedures with limited chance of significant blood loss, no laboratory or radiologic evaluation is necessary. Although it still may be commonly performed, routine testing of coagulation function has been shown to be of limited value without an antecedent history of bleeding problems. The most common coagulation disorder that may cause problems intraoperatively is von Willebrand disease, which cannot be identified on routine coagulation screening that includes a prothrombin time (PT), partial thromboplastin time (PTT), and an international normalized ratio (INR). Such problems are screened for by a thorough family and clinical history investigating possible clinical clues suggestive of coagulation problems, including prolonged bleeding after minor surgery such as dental extractions or trauma, excessive or heavy menstrual bleeding, easy bruising, or recurrent epistaxis. In patients presenting for surgical procedures that may require the administration of allogeneic blood products, hemoglobin is assessed and a type and screen obtained. In specific clinical scenarios—especially in the pediatric population, in whom awake phlebotomy may be problematic—these may be obtained and sent immediately following the induction of anesthesia and placement of an intravenous cannula. The latter is generally acceptable when there is no previous history of transfusion and an extremely low incidence of unexpected antibodies, which may result in type and cross issues.
Physical Feature | Clinical Finding |
---|---|
Length of upper incisors | Relatively long |
Relation of maxillary and mandibular incisors during normal closure | Overbite with maxillary incisors anterior to mandibular incisors |
Relation of maxillary and mandibular incisors during voluntary protrusion of mandible | Cannot bring mandibular incisors in front of maxillary incisors |
Inter-incisor distance | Less than 3 cm (adult) or less than two finger breadths a |
Visibility of uvula | Mallampati grade 3 or 4 |
Shape of the palate | Highly arched or narrow |
Size and/or integrity of the submandibular space | Small and/or indurated, firm or mass present |
Thyromental distance | Less than 3 cm (adult) or less than 3 finger breadths a |
Length of neck | Shorter length |
Neck circumference | Larger neck circumference |
Range of motion of head and neck | Limited flexion and extension |
a For this evaluation in a child, the clinician should use the patient’s fingers.
Classification | Description | Example |
---|---|---|
1 | Normal healthy patient | — |
2 | Mild systemic disease with no functional limitation | Mild asthma, acyanotic congenital heart disease (atrial septal defect) |
3 | Severe systemic disease with functional limitation | Sickle cell disease, cystic fibrosis, palliated cyanotic congenital heart disease |
4 | Severe systemic disease that is a constant threat to life | Advanced stages of muscular dystrophy, cyanotic congenital heart disease with pulmonary hypertension |
5 | Moribund patient not expected to survive without operation | Perforated bowel with sepsis and shock |
6 | Brain-dead patient; organs are being removed for donor purposes | — |
E | Emergency operation | — |
Another area of ongoing controversy is the need for routine preoperative pregnancy testing in postmenarchal women. Given the theoretic potential for some anesthetic agents to be teratogenic and to increase the risk of spontaneous abortion, the history should include specific questioning about the potential for pregnancy, including the patient’s last menstrual cycle. There is increasing use of point-of-care urine pregnancy testing in many centers. Further tests—such as pulmonary function tests, electrocardiography, and echocardiography—are based solely on the presence of comorbid conditions. Following the preoperative visit, including the history and physical examination, the planned management of anesthesia is discussed with each patient. Risks and possible complications are reviewed. Options and plans for postoperative pain management are discussed. Obtaining answers to questions and an informed consent complete the preoperative evaluation.
Nothing-by-mouth guidelines
Although the aspiration of gastric contents is an uncommon event, the consequences may be severe and include pneumonitis, respiratory failure, or even death. Classical teaching states that the severity of the aspiration injury relates to the volume aspirated as well as its pH, with severe complications when aspiration includes a volume of 0.4 mL/kg or greater or a pH of 2.5 or less. Although aspiration may occur in any setting, patients at risk include parturients, obese patients, diabetics, patients who have received opioids, patients with gastrointestinal disease (reflux, obstruction), patients with altered mental status, patients with intraabdominal pathology (acute abdominal emergencies, including appendicitis), trauma patients, and those in whom difficult airway management is anticipated. Specific comorbid conditions may predispose to aspiration by limiting the patient’s ability to protect the airway, decreasing the normal barrier to aspiration (lower esophageal sphincter tone), increasing gastric volume, or delaying gastric emptying. , Patients who have the highest incidence of perioperative aspiration are those with a high ASA physical status classification (III–V) and those having emergency surgery. Although the incidence is highest during the induction of anesthesia, aspiration may occur intraoperatively and during the postoperative period following tracheal extubation. Keeping patients nil per os (NPO; nothing by mouth) remains the mainstay of therapy to limit the incidence and impact of acid aspiration. In the past, patients were fasted for 8 to 12 hours before surgery to reduce the volume of gastric contents at the time of induction of anesthesia and to decrease the risk of aspiration pneumonitis. However, these antiquated preoperative fasting guidelines did not take into account differences in gastric emptying of clear liquids and solids. Based on several investigations and clinical experience, there have been revisions in the perioperative fasting rules, especially for infants and children. Clear liquids have a gastric emptying time of 1 to 2 hours, whereas solids have an unpredictable gastric emptying time that may be greater than 6 hours. The ingestion of clear liquids up to 2 hours before surgery does not increase gastric fluid volume or acidity and, in fact, may reduce gastric volume and increase pH. As a result, the liberalization of guidelines for ingestion of clear liquids for elective surgery of otherwise healthy patients has been recommended. The guidelines from the ASA for patients with no known risk factors include no solid food for at least 6 hours before surgery and unrestricted clear liquids until 2 hours before surgery. Others have recommended the liberal allowance of clear liquids up to 1 hour prior to surgery. Importantly, instead of merely allowing clear liquids prior to surgery, their administration is encouraged, and parents are reminded to allow their children to have clear liquids (apple juice) prior to coming to the hospital. This improves hydration and limits irritability related to NPO time. Oral medications should be given 1 to 2 hours before surgery with a small sip of water. The latter is particularly important for specific medications such as anticonvulsants, as postoperative seizures may occur related to missing a single preoperative dose.
Although several maneuvers have been suggested in patients with risk factors for acid aspiration, there is limited if any evidence-based medicine to demonstrate their efficacy in preventing perioperative aspiration. Although the administration of preoperative medications to decrease the acidity of the gastric fluid (antacids, H 2 -antagonists, or proton pump inhibitors) and speed gastric emptying (metoclopramide), their efficacy in preventing aspiration and limiting its clinical effect has not been proven. To be effective, these medications need to be administered 60 to 90 minutes prior to anesthetic induction. Alternatively, a nonparticulate antacid (sodium bicitrate) can be given immediately prior to anesthetic induction, a common practice in obstetric anesthesia. However, not one of these practices has been shown to alter outcome when examined rigorously using evidence-based medicine.
In patients at risk for acid aspiration, rapid sequence induction (RSI) is commonly practiced. This involves the rapid and sequential administration of a rapidly acting neuromuscular blocking agent (discussed later) with an intravenous anesthetic agent and the application of cricoid pressure. As the cricoid is the only complete ring of the trachea, it can be gently pushed posteriorly to effectively occlude the esophagus and prevent passive regurgitation of gastric contents when consciousness is lost during anesthetic induction. In its classical form, RSI involves preoxygenation, the administration of an anesthetic agent (sedative) and NMBA in rapid sequence, and the performance of endotracheal intubation without bag-valve-mask ventilation. Bag-valve-mask ventilation is not provided, as it may distend the stomach and predispose to regurgitation. Without assisted ventilation, oxygen desaturation may occur in infants and small children, patients with reduced functional residual capacity, or those with significant alveolar space disease. Additionally, as the sedative and NMBA are administered in rapid sequence without demonstrating the ability to provide bag-valve-mask ventilation, this may result in a “cannot intubate, cannot ventilate” scenario. A modification of this technique, known as a modified RSI, uses gentle bag-valve-mask ventilation with a peak inflating pressure less than 20 cm H 2 O to provide oxygenation and ventilation while waiting for the anesthetic agent and NMBA to take effect. The modified RSI may be used more commonly in pediatric anesthesia, as even brief periods of apnea without bag-valve-mask ventilation may result in precipitous decreases in oxygenation due to the low functional residual capacity and high metabolic rate for oxygen in young children and infants. Although RSI is commonly practiced, it is not universally accepted and has not been tested using evidence-based medicine. It has been demonstrated that even in experienced hands, especially in young infants and children, the correct application of cricoid pressure is problematic.
Preoperative medication
There are several categories and uses of preoperative medications ( Table 129.3 ). The most common use of a preoperative medication is to provide sedation and anxiolysis prior to transport to the operating room. Preparing the patient for surgery includes psychologic preparation and frequently pharmacologic premedication to decrease separation anxiety in young children and facilitate the inhalational induction of anesthesia. Psychologic preparation includes the preoperative visit and an interview by the anesthesiologist. Pharmacologic premedication may be given orally, intranasally, or rarely intramuscularly, 30 to 60 minutes prior to the induction of anesthesia. In the adult population or in pediatric patients with a preexisting intravenous cannula, premedication may be administered immediately prior to transport to the operating room. Commonly used pharmacologic agents for premedication include benzodiazepines, such as midazolam, or occasionally α 2 -adrenergic agonists, such as clonidine or dexmedetomidine. A frequently used agent and route of administration for the pediatric patient is the oral administration of the benzodiazepine midazolam to ease separation from parents and improve mask acceptance for the inhalation induction of anesthesia. This is generally necessary when children are 9 to 18 months of age and begin to manifest stranger anxiety. Given alterations in bioavailability when administered by the oral route, doses of 0.3 to 0.5 mg/kg are required. Alternatively, in patients who refuse oral premedication, the intranasal route may be chosen. For this purpose, commonly used agents include both midazolam and dexmedetomidine.
Type of Medication | Purpose |
---|---|
Benzodiazepine | Sedation, anxiolysis, amnesia—eases parental separation |
α 2 -adrenergic agonists (clonidine, dexmedetomidine) | Sedation, anxiolysis—decrease intraoperative anesthetic needs |
Opioids | Analgesia during invasive procedures |
Anticholinergic agents (atropine, glycopyrrolate) | Prevent bradycardia, blunt airway reflexes, dry secretions |
Inhaled β-adrenergic agonists (albuterol) and anticholinergic agents (ipratropium) | Prevent or relieve bronchospasm |
Inhaled lidocaine | Prevents airway reflexes during awake fiberoptic intubation, direct laryngoscopy, or bronchoscopy |
H 2 -antagonists, proton pump inhibitors | Decrease pH of stomach contents |
Promotility agents, such as metoclopramide | Decrease volume of gastric secretions |
Antiemetic agents (scopolamine patch, neurokinin-1 inhibitors) | Prevent perioperative nausea and vomiting |
H 2 -antagonists, proton pump inhibitors, or motility agents may be administered preoperatively to increase gastric pH and decrease gastric volume in patients at risk for acid aspiration. However, there is no firm evidence-based medicine to demonstrate their efficacy in preventing or mitigating acid aspiration during emergent or elective procedures. Aerosolized β-adrenergic agonists (albuterol) and anticholinergic agents (ipratropium) may be administered preoperatively to prevent bronchospasm in patients with reactive airway diseases (asthma, recent upper respiratory infection, or chronic obstructive pulmonary diseases). Anticholinergic agents may also be used to dry airway secretions in patients requiring fiberoptic intubation.
Monitoring
The ASA has outlined standards for basic intraoperative monitoring. The monitoring standards are the same regardless of whether the case entails a general anesthetic, regional anesthetic (peripheral nerve block, spinal, or epidural), or monitored anesthesia care. These monitoring guidelines are similar to those suggested for procedural sedation performed by nonanesthesiologists. The standards according to the ASA include an oxygen analyzer, noninvasive blood pressure cuff, continuous electrocardiogram (ECG), pulse oximeter, end-tidal carbon dioxide monitoring, a temperature probe, and a ventilator disconnect alarm.
Although arrhythmias are generally uncommon, bradycardia may occur due to the administration of the volatile anesthetic agents (halothane or sevoflurane), hypoxemia, hypothermia, alterations in intracranial pressure, or from the oculocardiac or trigeminocardiac reflex. The incidence of significant bradycardia has decreased with the transition to the newer volatile anesthetic agent sevoflurane, which is generally devoid of significant negative chronotropic properties when compared with halothane. However, clinically significant bradycardia may occur with sevoflurane in patients with trisomy 21. , In most clinical scenarios, a three-lead ECG is used intraoperatively with monitoring of lead II to facilitate the identification of P wave morphology and arrhythmia analysis. In adult patients and in specific pediatric patients, a five-lead ECG may be used to facilitate ischemia detection, including monitoring V 5 for the anterior myocardium. Other potential applications for intraoperative ECG monitoring include identification of potentially lethal electrolyte disturbances, such as hyperkalemia, prolonged QT syndrome (congenital, acquired, or drug induced), and monitoring for inadvertent systemic injection of local anesthetic agents. By using the T-wave, systolic blood pressure (BP), and heart rate (HR) criteria, the positive response rate to a test dose containing epinephrine (0.1 mL/kg of a 1:200,000 solution or 0.5 µg/kg of epinephrine) was 100%, 95%, and 71%, respectively, during sevoflurane anesthesia and 90%, 71%, and 71%, respectively, during halothane anesthesia. ,
Pulse oximetry estimates the saturation percentage of the hemoglobin. It is not meant as a surrogate measure of the partial pressure of oxygen in the blood (Pa o 2 ), especially at its extreme values. The relationship between Pa o 2 and oxygen saturation is affected by many factors, including the type of hemoglobin and the state of the oxyhemoglobin dissociation curve. The latter is affected by acid-base status, temperature, and 2,3-diphosphoglycerate (DPG) levels. Pulse oximetry is known to be inaccurate during periods of hypoxemia (saturation <85%–90%) and generally reads 98% to 100% when the Pa o 2 exceeds 100 mm Hg. Both patient-related and external factors—including motion, ambient light, and low tissue perfusion—may interfere with its accuracy. New technology has led to the introduction of low-perfusion pulse oximetry that provides accurate readings during low-perfusion states; devices using 6 to 8 wavelengths of light instead of 2 wavelengths that can identify abnormal hemoglobin species (carboxyhemoglobin, methemoglobin); improved accuracy at saturation levels less than 90%; and alternative sites for monitoring, such as the forehead, which may not be affected by low perfusion states.
End-tidal carbon dioxide (ETCO 2 ) monitoring, or capnography, remains a standard of care for intraoperative monitoring. It not only documents correct placement of the endotracheal tube within the trachea but also ensures ongoing ventilation during the case. The capnogram displays the patient’s exhaled CO 2 concentration continuously during exhalation, with the ETCO 2 being the peak value before the next breath is initiated. When used continuously in the operating room setting, the technology provides a continuous estimation of the partial pressure of CO 2 (Pa co 2 ) in the blood; serves as a disconnect alarm during mechanical ventilation, monitoring respiratory rate; and provides information regarding pulmonary function via the shape of the capnogram. Abrupt changes in the ETCO 2 , such as decreases related to increased dead space, may alert the clinician to decreased cardiac output or alterations in pulmonary perfusion related to gas or pulmonary embolism. Acute increases in ETCO 2 may be the initial sign of malignant hyperthermia or other hypermetabolic states.
The normal capnogram has three phases of exhalation and one of inspiration, generally labeled as phases 1 through 4. Phase 1 is the beginning of exhalation, representing dead space ventilation and therefore having no ETCO 2 present. If ETCO 2 is present during phase 1, this indicates the rebreathing of exhaled gas, which may be due to inadequate fresh gas flows. Phase 2 is the rapid and steep upslope of the capnogram, representing the emptying of alveolar gas with dead space gas. Phase 3 is the plateau phase that, in the normal state, should be relatively horizontal. Upsloping of phase 3 of the capnogram is indicative of obstructive lung disease (asthma, bronchospasm) with differential emptying of alveoli with varying time constants. With the initiation of inspiration, there is an abrupt decrease of the ETCO 2 (phase 4), which should return to 0 mm Hg. The ETCO 2 generally correlates in a clinically useful fashion with the Pa co 2 , with the ETCO 2 being 2 to 4 mm Hg lower than the Pa co 2 , and can be used to adjust intraoperative ventilation. However, this correlation is dependent on effective matching of ventilation with perfusion. The relationship between the ETCO 2 and Pa co 2 may be affected by technology and patient-related factors. Such issues may be particularly relevant in the practice of pediatric anesthesia, in which smaller tidal volumes, type of ventilation (continuous vs. intermittent flow), and sampling issues may have an effect. With these caveats in mind, its use has expanded outside of the operating room with its addition to the suggested monitoring guidelines for procedural sedation from the ASA, which are used to judge the adequacy of resuscitation and to prevent inadvertent hyperventilation during patient transport. ,
Based on the medical condition of the patient and the surgical procedure, more invasive monitoring may be added to these standard monitors, such as a urinary catheter and catheters for measuring continuous intraarterial pressure, central venous pressure, and pulmonary artery (PA) pressure. In the practice of pediatric anesthesia, there are no strict guidelines dictating which patients should have invasive monitors placed. However, their use is generally considered in patients undergoing procedures with the potential for large or rapid blood loss, patients with underlying hemodynamic instability, those receiving vasoactive agents, and those undergoing surgery for congenital heart disease. Invasive monitoring of cardiac output and PA pressure is rarely if ever used in the practice of pediatric anesthesia. In addition, its use in the adult population has dramatically decreased over the years given the lack of evidence-based medicine to demonstrate a difference in clinical outcomes.
Instead of the use of a PA catheter, information regarding structural and functional issues of the myocardium may be obtained with transesophageal echocardiography (TEE). TEE is being used more frequently in the adult population with the development of a specific curriculum to teach the skills necessary to perform TEE during cardiac anesthesia fellowships. The latter has been followed by the American Board of Anesthesiology, which recognizes such training and provides the opportunity for credentialing through the completion of a written examination. The strongest indications for perioperative TEE that are supported by evidence-based medicine include cardiac surgery procedures such as repair of valvular lesions (insufficiency or stenosis) or congenital lesions, assessments and repairs of thoracic aortic aneurysms and dissections, pericardial window procedures, and the repair of hypertrophic obstructive cardiomyopathy. For noncardiac surgery, intraoperative TEE may be indicated to evaluate acute, persistent, and life-threatening hemodynamic disturbances in which ventricular function and its determinants are uncertain and have not responded to treatment, especially when placement of a PA catheter is not feasible.
Given the current limitations of the clinically available measures of cardiac output (TEE or PA catheter), there is ongoing interest in the development of accurate and noninvasive means of assessing cardiac output. Doppler-based techniques—specifically, transesophageal Doppler—provide a noninvasive alternative with accuracy that is similar to, though not superior to, thermodilution. Calibrated pulse contour analysis is superior to uncalibrated pulse contour analysis, though both tend to suffer during periods of hemodynamic instability. Bioreactance is completely noninvasive and shows promising accuracy in comparison to thermodilution. However, it is a relatively new technology when compared with its thermodilution and Doppler counterparts. More research is needed before meaningful conclusions can be made regarding the clinical utility of bioreactance, whereas bioimpedance monitors have shown poor accuracy and cannot be recommended for clinical use. At this point, none of these technologies can be considered the standard of care. In most circumstances, their use is confined to clinical investigations, with limited applications in standard operating room patient management.
In addition to the standard ASA monitors and other monitors of hemodynamic function previously discussed, there is growing interest in the development and potential use of depth of anesthesia monitors to allow titration of anesthetic agents (inhaled and intravenous). Although these monitors may have several potential benefits, their primary role has been to prevent intraoperative awareness. Although controversial, the potential impact of such monitors is highlighted by trials demonstrating that intraoperative awareness occurs in approximately 0.1% to 0.2% of patients. Specific patient populations are at higher risk, including those undergoing trauma, cardiac, obstetric, and emergent surgery. Several manufacturers have marketed or are developing monitors that provide the anesthesia provider with a numeric value against which anesthetic agents are titrated. The most commonly used monitors include the Bispectral Index (BIS monitor, Covidien); the Narcotrend, which is currently available only in Europe; the Patient State Analyzer; SNAP; the SedLine Brain Function Monitor (Masimo); and Auditory Evoked Potential Monitor (AEP Monitor, Danmetter Medical). The first one introduced into clinical use was the BIS and remains the one used most commonly in clinical practice. The BIS is a modified electroencephalographic (EEG) monitor that uses a preset algorithm based on intraoperative EEG data obtained from adults who were anesthetized with agents that work through the γ-amino butyric acid (GABA) pathway. The BIS number is determined from three features of the EEG tracing: (1) the amplitude and frequency of the waves, (2) the synchronization of low- and high-frequency information, and (3) the percentage of time in burst suppression (flat EEG). The output of the processed EEG then provides a depth of sedation/anesthesia that is displayed numerically, ranging from 0 to 100, with 40 to 60 being the proposed suitable level of anesthesia to ensure amnesia. With the use of BIS monitoring, a decreased incidence of awareness has been demonstrated as well as a decrease in the total amount of anesthetic agent used. Additional studies have suggested faster recovery times and faster discharge times from the postanesthesia care unit, both of which may translate into reduced perioperative costs. , Although not considered the standard of care for intraoperative monitoring, the ASA does recommend the availability of such monitors whenever general anesthesia is provided and suggests their use in clinical scenarios in which the patient may be at high risk for awareness, including situations involving patients who have had a previous episode of awareness. Given the success of such monitors in the perioperative arena, there is ongoing interest in the application of this technology in the intensive care unit (ICU) and procedural sedation arena.
Pharmacology of anesthetic agents
Local anesthetic agents
The local anesthetic agents can be divided into two chemically distinct classes: esters and amides. Local anesthetic agents in the amino ester class include procaine, chloroprocaine, and tetracaine. Amino amides include lidocaine, mepivacaine, prilocaine, bupivacaine, levobupivacaine, and ropivacaine. Clinically important differences between these two classes of local anesthetic agent include their site of metabolism, plasma half-life, adverse effect profile (CNS vs. cardiac toxicity), and allergic potential (discussed later). Amino esters are metabolized by plasma cholinesterases, whereas amino amides undergo hepatic metabolism. As there is limited change in the activity of plasma cholinesterase based on chronologic and gestational age, there may be inherent safety advantages to using these agents in the neonatal population.
Local anesthetic agents block the sodium channels in the nerve membrane, preventing depolarization and impulse propagation. The nonionized portion of the local anesthetic agent penetrates the lipid membrane, whereas the ionized portion reversibly blocks the inner aspect of the sodium channel. Local anesthetic agents differ in intrinsic potency, onset of action, duration of action, and their ability to produce differential sensory and motor blockade. Potency is determined primarily by lipid solubility (high lipid solubility = potency). Bupivacaine and tetracaine are examples of local anesthetic agents with high lipid solubility and, hence, high potency. The onset of action is determined primarily by the pK a , with onset being most rapid in agents with a pK a closest to the physiologic pH. , With a pK a close to the physiologic pH, the percentage of the unionized form is greater, increasing passage through the lipid nerve membrane. Lidocaine has a pK a of 7.7 at a pH of 7.4; 35% exists in the unionized base form, yielding a relatively rapid onset of blockade. In contrast, tetracaine has a pK a of 8.6 with only 5% in the unionized form at a tissue pH of 7.4, resulting in a slower onset of blockade than lidocaine. Last, the duration of action is determined primarily by the degree of protein binding to receptors in the sodium channel. High protein binding and therefore a prolonged duration of action are characteristic of bupivacaine, levobupivacaine, tetracaine, and ropivacaine. Duration of action is also influenced by the degree of vasodilation produced by the local anesthetic. Vasodilation results in increased blood flow to the area and therefore an increased removal of the agent from the depot in the tissues. The local anesthetic agents also differ in their differential effects on sensory versus motor nerves. Bupivacaine and ropivacaine demonstrate this property, which is beneficial for postoperative analgesia whether provided as a single injection or administered through an epidural catheter. The differential blockade allows patients to ambulate, as they have limited motor weakness, and yet sensory blockade provides analgesia.
When performing regional anesthesia, the goal is to place the local anesthetic agent in close proximity to the nerve or plexus that needs to be anesthetized. An addition to the armamentarium of the anesthesiologist has been the use of ultrasound to visualize the individual nerve roots or the plexus that is to be anesthetized. This technology is also being used for neuraxial techniques, including spinal and epidural anesthesia. In both the adult and pediatric population, the use of ultrasound has been shown to increase the success rate of various regional anesthetic techniques and to result in successful blockade with a decreased dose of the local anesthetic agent.
Given the catastrophic effects of local anesthetic toxicity, mechanisms to avoid it and prevent its occurrence are mandatory during the performance of regional anesthetic techniques in infants and children. Epinephrine (5 μg/mL or a concentration of 1:200,000) is added to the local anesthetic solution during performance of a regional anesthetic technique to cause local vasoconstriction, decreasing the vascular absorption of the drug and serving as a marker of inadvertent systemic injection. Even with negative aspiration for blood, there is the potential for inadvertent intravascular administration. As such, the use of a test dose is common practice for both peripheral and neuraxial blockade as a means of identifying inadvertent systemic injection of the local anesthetic solution. Epinephrine is added in a 1:200,000 concentration (5 μg/mL). In adults, the test dose includes 3 mL or 15 μg of epinephrine. In the pediatric population, 0.1 mL/kg of the local anesthetic solution is administered, which results in the delivery of 0.5 μg/kg of epinephrine. If this amount of epinephrine is injected intravascularly, it can generally be detected by changes in HR, blood pressure, or the ST-T wave segments of the electrocardiogram and can alert the practitioner that inadvertent intravascular injection is occurring.
The site of injection of the local anesthetic agent also has a significant impact on the clinical effects, including the duration of action and vascular uptake (plasma concentrations). The shortest duration of action occurs with either intrathecal injection for spinal anesthesia or subcutaneous administration. The longest duration of action and onset of blockade are seen with major peripheral nerve blocks (brachial or lumbar plexus blockade). The highest plasma concentration occurs with an intercostal nerve block or interpleural analgesia followed, in order, by caudal epidural, lumbar or thoracic epidural, brachial plexus, peripheral nerve blockade, subarachnoid, and subcutaneous infiltration.
The greatest risk of morbidity and mortality related to the use of local anesthetic agents is the potential to achieve a toxic plasma concentration of the drug. Local anesthetic-induced systemic toxicity affects the CNS and the CV system. The differential effects on these two organ systems and the plasma concentration at which toxic effects are noted vary according to the agent. With most local anesthetic agents, CNS toxicity occurs at doses and blood levels below those that produce CV toxicity. The latter provides some degree of safety, as the CNS symptoms (seizures) are generally more amenable to treatment than the CV effects (arrhythmias and conduction blockade). Death from local anesthetic toxicity is most commonly the result of the CV effects of these agents with adverse effects on cardiac electrical and mechanical activity. Bupivacaine produces cardiac arrhythmias by inhibiting the fast sodium channels and the slow calcium channels in the cardiac membrane. Hypercarbia, acidosis, and hypoxia potentiate the negative chronotropic and inotropic effects of high plasma concentrations of local anesthetic agents. These effects are so profound that resuscitative measures for ventricular tachycardia/fibrillation, including standard Advanced Cardiac Life Support protocols, may be ineffective. Anecdotal case reports have suggested the potential role of various agents such as amiodarone for refractory ventricular arrhythmias or even the use of extracorporeal support. Anecdotal human data and animal studies have suggested that intralipid solutions may be used to bind the local anesthetic agent, resulting in the return of spontaneous circulation. Current recommendations from the ASA and American Society of Regional Anesthesia include ready access to 20% intralipid solutions whenever large doses of local anesthetic agents are used for regional anesthetic techniques ( Box 129.1 ). Given the risks of morbidity and mortality from local anesthetic toxicity, avoidance of toxicity is the goal through the careful calculation of the dose, use of the lowest necessary dose (concentration and volume), use of a test dose with epinephrine to identify inadvertent intravascular injection, intermittent aspiration to identify vascular penetration, and slow incremental injection of the dose.
- 1.
Airway management to prevent or reverse hypoxia, hypercarbia, and acidosis
- 2.
20% lipid emulsion bolus:
- •
Bolus
- •
100 mL over 2–3 min if the patient is >70 kg
- •
1.5 mL/kg over 2–3 min if the patient is <70 kg
- •
- •
Infusion (continue for at least 10 min after return of spontaneous circulation)
- •
200–250 mL over 15–20 min if the patient weighs >70 kg
- •
0.25 mL/kg/min if patient weighs <70 kg
- •
- •
- 3.
Avoid using propofol
- 4.
Seizure control with benzodiazepines
- •
If seizures persist, use NMBA to reduce hypoxemia and acidosis
- •
- 5.
If cardiac arrest occurs:
- •
Use epinephrine (<1 μg/kg for hypotension; higher doses only for cardiac arrest)
- •
Avoid calcium channel blockers and β-adrenergic receptor antagonists
- •
Amiodarone is preferable if ventricular arrhythmias develop
- •
- 6.
Cardiopulmonary bypass or extracorporeal membrane oxygenation if no response to above therapies
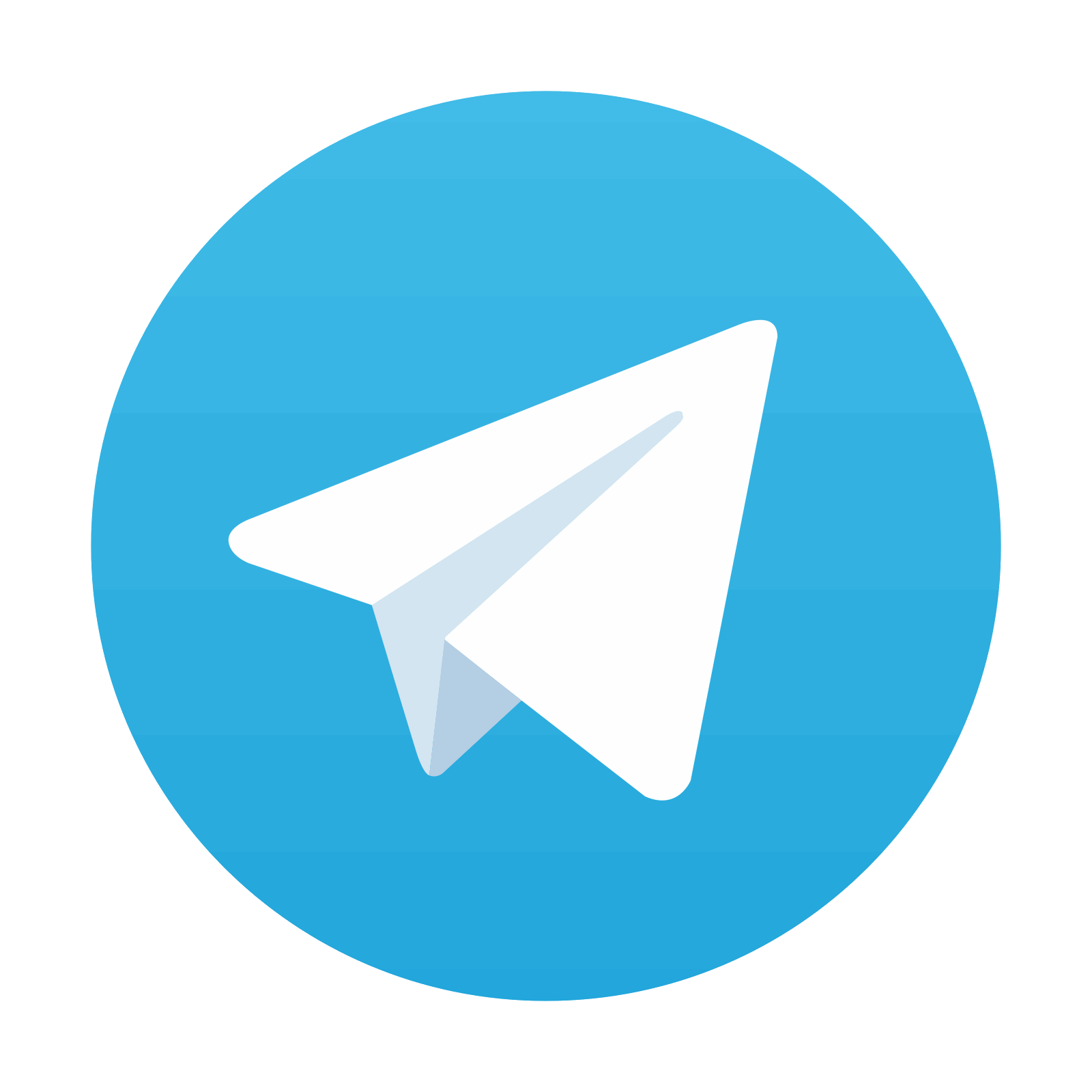
Stay updated, free articles. Join our Telegram channel

Full access? Get Clinical Tree
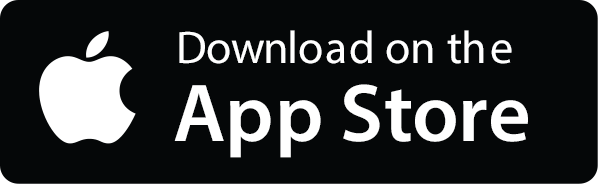
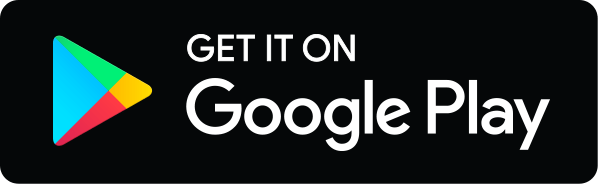
