Anesthesia for Cardiac Surgery
Nikolaos J. Skubas
Adam D. Lichtman
Aarti Sharma
Stephen J. Thomas
Key Points












Related Matter
Aortic Stenosis
Aortic Insufficiency
Mitral Stenosis
Mitral Regurgitation
Cardiopulmonary Bypass
Cardioplegia
Centrifugal and Roller Pumps
IABP
Anesthetizing patients who undergo cardiac surgery is exciting, intellectually challenging, and emotionally rewarding. The cardiac anesthesiologist should have a thorough understanding of normal and altered cardiac physiology; have knowledge of the pharmacology of anesthetic, vasoactive, and cardioactive drugs; and be familiar with the physiologic alterations associated with cardiopulmonary bypass (CPB) and the surgical procedures. This chapter presents a brief overview of the critical physiologic and technical considerations during cardiac surgical procedures. Some of the issues discussed are controversial because the field is continuously evolving. The authors have tried, whenever possible, not to allow their own preferences overshadow what is the consensus about the various topics presented in the chapter.
Coronary Artery Disease
The prevention or treatment of myocardial ischemia during coronary artery bypass graft (CABG) surgery is associated with a decreased incidence of perioperative myocardial infarction. The hemodynamic management is tailored to avoid factors known to increase myocardial oxygen demand (M[V with dot above]O2), particularly during the vulnerable pre-CPB period. Optimizing oxygen delivery to the myocardium is equally important for the successful management of these patients because it is well recognized that most ischemic events occur with minimal or no change in M[V with dot above]O2.1,2 The determinants of myocardial oxygen supply and demand are shown in Figure 38-1 and are also discussed in Chapter 10.
Myocardial Oxygen Demand
The principal determinants of M[V with dot above]O2 are wall tension and contractility.3 According to Laplace’s law, wall tension is directly proportional to intracavitary pressure and radius and inversely proportional to wall thickness. Therefore, myocardial oxygen demand can be reduced by interventions that (1) decrease intraventricular pressure and (2) prevent or promptly treat ventricular distention.
Myocardial Oxygen Supply

Coronary Blood Flow
The critical factors that modify coronary blood flow are the perfusion pressure and vascular tone of the coronary circulation, the time available for perfusion (determined mainly by heart rate), the severity of intraluminal obstructions, and the presence of (any) collateral circulation. The area most vulnerable to ischemia is the subendocardium of the left ventricle (LV), which is exposed to the LV intracavitary pressure and where metabolic requirements are increased because of greater systolic shortening.4
Perfusion of the LV subendocardium takes place almost entirely during diastole, whereas the right ventricular subendocardium is perfused in diastole and systole, assuming pulmonary hypertension is not present. This temporal disparity is explained by the different intraventricular pressures developing during systole.
The LV coronary perfusion pressure is often defined as the gradient between aortic diastolic (or mean) pressure and left ventricular diastolic pressure (LVDP; usually estimated by the pulmonary artery occlusion pressure). In the presence of intraluminal obstruction or increased vascular tone, this pressure gradient is reduced (Fig. 38-2). A low LVDP is ideal for improving perfusion (higher pressure gradient) and reducing M[V with dot above]O2 (decreased LV volume and wall tension). On the other hand, increasing perfusion pressure by raising the aortic pressure will increase M[V with dot above]O2. However, this is not as important, when one considers that tachycardia is the most important cause of intraoperative and perioperative ischemia.
Alterations in the tone of the small intramyocardial arterioles regulate diastolic vascular resistance, allowing the matching of oxygen supply with metabolic demand over a wide range of perfusion pressures.5 The difference between autoregulated, baseline flow, and blood flow available under conditions of maximal vasodilation is termed coronary vascular reserve and is normally three to five times higher than basal flow. As epicardial coronary stenosis becomes more pronounced, progressive vasodilation of these resistance vessels allows preservation of basal flow, but at
the cost of reduced reserve. Once perfusion pressure decreases to <40 mm Hg, autoregulation of subendocardial coronary flow is lost. Whenever demand increases above available reserve, signs, symptoms, and metabolic evidence of ischemia develop.
the cost of reduced reserve. Once perfusion pressure decreases to <40 mm Hg, autoregulation of subendocardial coronary flow is lost. Whenever demand increases above available reserve, signs, symptoms, and metabolic evidence of ischemia develop.
Prinzmetal et al.6 first described angina and myocardial infarction in patients with angiographically normal coronary vessels. Other causes of infarction include prolonged hypotension and acute coronary thrombosis. Unstable angina pectoris and/or acute coronary thrombosis are the results of plaque rupture with ensuing platelet activation and thrombus formation.7 The presence of the potentially hyperreactive normal vessel wall adjacent to the thrombus may result in vasospasm8 and total occlusion of the vessel lumen in the presence of a previously nonocclusive eccentric plaque or thrombus.9 This type of acute thrombosis is believed to be the cause of most acute myocardial infarctions and associated sudden death (usually from ischemia-induced cardiac dysrhythmias).

Hemodynamic Goals

Monitoring for Ischemia
The ideal monitoring technique is not yet available. Analysis of the ST segment in multiple leads (most commonly leads II and
V4 or V5) is currently the standard. Patients likely to develop right ventricular ischemia or those with disease of the right coronary artery might benefit from monitoring of leads V4R or V5R. The ST segment from various ECG leads should be continuously monitored and displayed and interactive monitors should alarm when the ST segment deviates from baseline.
V4 or V5) is currently the standard. Patients likely to develop right ventricular ischemia or those with disease of the right coronary artery might benefit from monitoring of leads V4R or V5R. The ST segment from various ECG leads should be continuously monitored and displayed and interactive monitors should alarm when the ST segment deviates from baseline.
Table 38-1. Coronary Artery Disease—Hemodynamic Goals | ||||||||||||||||
---|---|---|---|---|---|---|---|---|---|---|---|---|---|---|---|---|
|
Multiple attempts have been made to determine ischemic thresholds using commonly measured hemodynamic variables. Among the earliest of these was the rate–pressure product (RPP = heart rate × peak systolic arterial pressure). The RPP was considered an easily determined index of M[V with dot above]O2. Although RPP may correlate with oxygen demand, especially during exercise, it is not a sensitive or specific indicator of intraoperative ischemia; identical RPPs can be produced from multiple combinations of heart rate and blood pressure. Favorable conditions for oxygen balance are more likely those of lower heart rate and higher blood pressure than tachycardia and hypotension. Neither the ratio of mean arterial pressure over heart rate nor the diastolic to systolic pressure–time index is any more predictive or reliable than the RPP.13
Sudden elevations in pulmonary artery or capillary wedge pressure indicating LV dysfunction, large a waves reflecting decreased LV compliance, and v waves indicative of increased left atrial pressure because of ischemia-induced papillary muscle dysfunction and/or mitral regurgitation (MR) are purported signs of ischemia that may be detected with a pulmonary artery catheter (PAC). PAC-based monitoring of filling pressures, saturation of mixed venous blood in oxygen, and thermodilution cardiac output is common practice in cardiac surgery centers.14,15 Several studies contradict this long-held dogma and demonstrate that the PAC is of little value as a monitor of myocardial ischemia. Leung et al.16 found that only 10% of all regional wall motion abnormalities were associated with an acute rise in pulmonary capillary wedge pressure in 40 patients undergoing elective CABG surgery. Haggmark et al.17 found that neither an increase nor an abnormal pulmonary capillary wedge pressure waveform was a sensitive indicator for myocardial ischemia in 53 patients with coronary artery disease undergoing vascular surgery. A prospective study of 1,094 patients by Tuman et al.18 showed that even high-risk cardiac surgical patients may be safely managed without routine use of a PAC, and if the need for it developed intraoperatively, delayed placement of a PAC did not influence outcome. Fontes et al.19 assessed the limitations of PAC in the management of critically ill patients in the intensive care unit (ICU). Compared with transesophageal echocardiography (TEE), PAC predicted normal left ventricular function well, but performed poorly in judging preload and ventricular dysfunction. A more recent study showed no benefit from PACs in high-risk surgical patients.20 Others prefer to insert a PAC in selected patients, when needed.21 The most recent American Society of Anesthesiologists Practice Guidelines concluded that the evidence regarding the benefit that cardiac surgery patients receive from PAC is conflicting.22 However, it is difficult to determine the value of PAC in diagnosis and treatment when the physician’s ability for correct interpretation of PAC information is still in doubt.23
Since its introduction in the 1980s, TEE has become an invaluable diagnostic and monitoring tool during cardiac surgery. TEE permits assessment of ventricular volume, global and regional function, estimation and quantitation of valvular pathology, measurement of valve gradients and calculation of filling pressures, visualization of the thoracic aorta, and detection of intracardiac air. Practice guidelines for perioperative TEE have been recently published by the ASA.24 Experienced cardiac anesthesiologists who are supported by continuous quality programs perform comprehensive TEE studies25 and interpret TEE examinations at a level comparable with physicians whose primary practice is echocardiography.26
Multiple image planes are necessary to evaluate the three-dimensional structure of the heart. The recent introduction of real-time three-dimensional TEE introduced a real revolution in perioperative imaging, particularly of the mitral valve. The American Society of Echocardiography/Society of Cardiovascular Anesthesiologists task force for intraoperative echocardiography has published guidelines for performing a comprehensive intraoperative echocardiographic examination.27 These recommendations describe a series of 20 standard tomographic views of the heart and great vessels that should be included in a complete intraoperative echocardiographic examination. With experience, a thorough examination can be performed in less than 10 minutes.
Selection of Anesthetic

Early extubation is common practice and is achieved by multiple approaches.28,29 The choice of anesthetic should be based on known hemodynamic, pharmacologic, and pharmacokinetic effects of each drug as they apply to the particular patient, the experience of the anesthesiologist, and the relative cost–benefit of each agent. Volatile anesthetics with low-dose narcotics or total intravenous anesthesia with short-acting drugs (e.g., midazolam, alfentanil, remifentanil, propofol) have been used to effect early extubation. The increased use of benzodiazepines and volatile agents has been associated with low incidence of awareness.30 Intraoperative clinical variables are important factors to be considered in the timing of postoperative extubation after fast-track cardiac surgery. Inotrope use and platelet transfusion were the most significant determinants of early (<10 hours postoperatively) tracheal extubation in a Veterans Administration population.31
Opioids
The primary advantages of opioids are lack of myocardial depression, maintenance of a stable hemodynamic state, and reduction of heart rate. However, they cannot provide complete anesthesia. Notable side effects include (i) hypertension and tachycardia during surgical stimulation (sternotomy and aortic manipulation), especially in patients with good ventricular function; (ii) predictable hypotension when combined with benzodiazepines; (iii) lack of titratability when used in high doses; and (iv) a low incidence of intraoperative recall if used as the sole anesthetic. The current practice is to supplement the opioid with benzodiazepines and volatile agents, and many of the previous side effects may be of historical interest. A high-dose opioid-based technique may be of value only in the patient with severe myocardial dysfunction. The planned time of extubation is now one of the major factors determining the selection and dosage of opioid. Shorter-acting opioids (sufentanil and remifentanil) produce equally rapid extubation, similar ICU stay, and similar costs to fentanyl. Thus, any of these opioids can be
used for fast-track cardiac surgery.32 The beneficial cardioprotective and anti-inflammatory effects of morphine have been reconsidered recently,33,34 bringing back into the foray the opioid that reinvigorated the practice of cardiac anesthesia.35
used for fast-track cardiac surgery.32 The beneficial cardioprotective and anti-inflammatory effects of morphine have been reconsidered recently,33,34 bringing back into the foray the opioid that reinvigorated the practice of cardiac anesthesia.35
Inhalation Anesthetics
The desirable features of volatile anesthetics include dose-dependent hemodynamic changes, easy reversibility, titratable myocardial depression, amnesia, and suppression of sympathetic responses to surgical stress and CPB. Volatile anesthetics protect the myocardium from ischemia and reperfusion injury and reduce myocardial infarct size.36 This beneficial effect has been shown when volatile anesthetics are administered before a period of prolonged ischemia (“anesthetic preconditioning”) as well as during reperfusion (“anesthetic postconditioning”).37 However, it is difficult to ascertain whether these laboratory-proven benefits have contributed to improved myocardial protection in clinical practice.38 Disadvantages include systemic hypotension (whether induced by decreased contractility or vasodilation), which may compromise the oxygen supply and lack of postoperative analgesia. Combinations of opioids and volatile anesthetics retain their advantages with minimal untoward effects. Any of the volatile agents can be used in a balanced technique.
Isoflurane is a coronary vasodilator, as are the other volatile anesthetics (although to a lesser degree). This dose-related effect is clinically insignificant in doses less than 1 MAC. Clinical studies using isoflurane to clinical rather than pharmacologic end points have not shown increased episodes of ischemia or a worsened outcome.39
Desflurane and sevoflurane have the fastest recovery of all volatile anesthetics. Desflurane has a rapid uptake and distribution, allowing it to be useful in cases in which hemodynamic changes mandate rapid changes in anesthetic depth. It has a cardiac profile similar to that of isoflurane. In patients undergoing noncardiac surgery, desflurane increases pulmonary artery pressure, wedge pressure, and pulmonary vascular resistance (PVR) compared with isoflurane.40 When studying sympathetic nervous system activity, Helman et al.41 found an increase in sympathetic activity and myocardial ischemia in patients anesthetized with desflurane as the sole anesthetic agent for coronary artery bypass surgery compared with patients anesthetized with sufentanil. Compared with isoflurane, in a technique combining fentanyl with the inhalational anesthetic, sevoflurane had an acceptable cardiovascular profile prior to CPB and similar outcome data.42
Intravenous Sedative Hypnotics
An alternative adjuvant anesthetic to a low-dose opioid technique is a titratable intravenous infusion of a short-acting sedative, such as midazolam,43 propofol, or dexmedetomidine.44 These can be continued postoperatively in the ICU and afford a predictable and fairly rapid awakening after discontinuation.45 When compared with volatile anesthetics, propofol was associated with less favorable cardiac function, higher need for inotropic support, and elevated plasma troponins after cardiac surgery in elderly patients.46
Treatment of Ischemia
The use of anesthetics or vasoactive drugs that enable the heart to return to the slower-rate, smaller-size, and well-perfused state is frequently essential during anesthesia. The principal vasoactive drugs are nitrates, β-blockers, peripheral vasoconstrictors, and calcium entry blockers. Clinical scenarios for their use are given in Table 38-2. These drugs are discussed briefly here. Volatile anesthetics can also be used to control blood pressure and reduce contractility.
Table 38-2. Treatment of Intraoperative Ischemia | ||||||||||||||||||||||
---|---|---|---|---|---|---|---|---|---|---|---|---|---|---|---|---|---|---|---|---|---|---|
|
Nitrates
Nitroglycerin (TNG) is the drug of choice for the treatment of acute myocardial ischemia. Its action is via systemic venodilation that decreases LV preload, wall tension, M[V with dot above]O2, and coronary arterial dilation, which is operative in both stenosed coronaries and collateral beds.47 The evidence for the prophylactic use of TNG for prevention of either intraoperative ischemic episodes or postoperative cardiac complications is unconvincing.48 At higher doses, TNG dilates arterial beds and may cause systemic hypotension. Compensatory tachycardia may increase heart M[V with dot above]O2. The recommended TNG dose is 0.5 to 3 μg/kg/minute and is reduced in the presence of hepatic and/or renal disease. TNG may cause methemoglobinemia especially in patients with methemoglobin reductase deficiency; this complication is more likely when large doses are administered over a prolonged time.49 TNG is administered via special intravenous tubing that does not adsorb the drug.
Sodium Nitroprusside
Sodium nitroprusside (SNP) decreases peripheral vascular resistance by metabolic or spontaneous reduction to nitric oxide. Similar to TNG, SNP improves ventricular compliance in the ischemic myocardium. The recommended SNP dose is 0.5 to 3 μg/kg/minute and should be reduced in the presence of hepatic and/or renal disease. Adverse effects include cyanide and thiocyanate toxicity, rebound hypertension, intracranial hypertension, blood coagulation abnormalities, increased pulmonary shunting, and hypothyroidism. In vitro findings suggest that cardiac surgical patients may be at increased risk of cyanide toxicity in response to the perioperative administration of SNP.50 Cyanide is produced when SNP is metabolized; toxic blood levels (>100 μg/dL) occur when >1.0-mg/kg SNP is administered within 2 hours or when >0.5 mg/kg/hour is administered within 24 hours. The presenting signs of cyanide toxicity include the triad of elevated mixed venous O2 (Pvo2), requirements for increasing SNP dose (tachyphylaxis),
and metabolic acidosis.51 In addition, the patient may appear flushed. Greater risk of cyanide toxicity exists in patients who are nutritionally deficient in cobalamine (vitamin B12 compounds) or in dietary substances containing sulfur. Measurement of blood cyanide and pH will enable detection of abnormalities in high-risk patients for whom larger than recommended amounts of SNP have been used (8 to 10 μg/kg/minute). Treatment should consist of discontinuing infusion, administering 100% O2, administering amyl nitrate (inhaler) or intravenous sodium nitrite and intravenous thiosulfate, except in those patients with abnormal renal function, for whom hydroxocobalamin is recommended. Circulating levels of thiocyanate increase when renal function is compromised, and central nervous system abnormalities result when thiocyanate levels reach 5 to 10 μg/dL. Lowering the SNP dose requirement or, better, replacing SNP with nicardipine, metoprolol, and esmolol reduces or eliminates the consequent buildup of cyanide. Once dissolved, SNP deteriorates in the presence of light. The container, therefore, should be wrapped in aluminum foil. An unstable SNP ion in aqueous solution reacts with various substances within 3 to 4 hours, forming colored salts. Other drugs should not be infused in the same solution as SNP.
and metabolic acidosis.51 In addition, the patient may appear flushed. Greater risk of cyanide toxicity exists in patients who are nutritionally deficient in cobalamine (vitamin B12 compounds) or in dietary substances containing sulfur. Measurement of blood cyanide and pH will enable detection of abnormalities in high-risk patients for whom larger than recommended amounts of SNP have been used (8 to 10 μg/kg/minute). Treatment should consist of discontinuing infusion, administering 100% O2, administering amyl nitrate (inhaler) or intravenous sodium nitrite and intravenous thiosulfate, except in those patients with abnormal renal function, for whom hydroxocobalamin is recommended. Circulating levels of thiocyanate increase when renal function is compromised, and central nervous system abnormalities result when thiocyanate levels reach 5 to 10 μg/dL. Lowering the SNP dose requirement or, better, replacing SNP with nicardipine, metoprolol, and esmolol reduces or eliminates the consequent buildup of cyanide. Once dissolved, SNP deteriorates in the presence of light. The container, therefore, should be wrapped in aluminum foil. An unstable SNP ion in aqueous solution reacts with various substances within 3 to 4 hours, forming colored salts. Other drugs should not be infused in the same solution as SNP.
Vasoconstrictors
Vasoconstrictors (phenylephrine, norepinephrine, vasopressin) are useful adjuncts in the prevention and treatment of ischemia because they increase systemic blood pressure, thereby improving coronary perfusion pressure, albeit at the expense of increasing afterload and perhaps M[V with dot above]O2. In addition, concomitant venoconstriction increases venous return and LV preload. TNG is sometimes added to counteract any increase in preload. In most situations, the increase in coronary perfusion pressure more than offsets any increase in wall tension. Peripheral vasoconstriction is indicated during episodes of systemic hypotension, especially those caused by reduced surgical stimulation or drug-induced vasodilation. No one vasoconstrictor is superior to all others. Occasionally, a combination of vasoconstrictors (e.g., norepinephrine and vasopressin) may be needed to achieve the desired blood pressure.52
β-blockers
β-adrenergic blockade improves myocardial oxygen balance by preventing or treating tachycardia and by decreasing contractility. Any concomitant myocardial depression is counterbalanced by the anti-ischemic effects. Indications for β-blockers include treatment of sinus tachycardia not resulting from the usual causes (e.g., light anesthesia, hypovolemia); prophylaxis of, and slowing the ventricular response to, supraventricular dysrhythmias; decreasing heart rate and contractility in hyperdynamic states; and control of ventricular dysrhythmias.53,54 The use of β-blockers should aim at reducing the heart rate and increasing the diastolic filling time without, at the same time, decreasing the perfusion pressure and cardiac output. These therapy targets are even more important, since the POISE study revealed that death and stroke were side effects of β-blockers.55,56 Intravenous preparations include propranolol, metoprolol, labetalol, and esmolol. Propranolol is a nonselective β-blocker with an elimination half-life of 4 to 6 hours. Metoprolol is similar to propranolol but has the purported advantage of β1-selectivity and is less likely to trigger bronchospasm in patients with reactive airway disease. Labetalol combines β-blocking properties with those of α-blockade and is useful in treating hyperdynamic and hypertensive situations. Esmolol is a short-acting β1-blocker that is cardioselective, with a half-life of only 9.5 minutes. It is particularly useful in treating transient increases in heart rate owing to episodic sympathetic stimulation.
Calcium Channel Blockers
Calcium channel blockers are useful in slowing the ventricular response in atrial fibrillation and flutter, as coronary vasodilators, and in the treatment of perioperative hypertension.57,58 In vitro, all calcium entry blockers depress contractility, reduce coronary and systemic vascular tone, decrease sinoatrial node firing rate, and impede atrioventricular conduction. Unlike the β-blockers, which are similar in both structure and pharmacodynamic effect, the calcium entry blockers vary remarkably in their predominant pharmacologic action. The negative inotropic effect is greatest with verapamil and less with nifedipine, diltiazem, and nicardipine (in decreasing order). Verapamil is useful in the treatment of supraventricular tachycardia and slowing the ventricular response in atrial fibrillation and/or flutter; however, its myocardial depressant effects may limit its usefulness in some patients. In patients with reduced myocardial function, intravenous diltiazem is effective in the treatment of atrial fibrillation and flutter by slowing atrioventricular conduction with minimal myocardial depression. It is also useful in decreasing sinus rate. Calcium channel blockers have been found to have cardioprotective effects during reperfusion. Nicardipine in particular has coronary antispasmodic and vasodilatory effects more than systemic arterial vasodilatory effects.59
Nifedipine, amlodipine, and nicardipine are prominent peripheral vasodilators, effective in the treatment of postoperative hypertension in cardiac surgical patients, with minimal side effects.60 The newer agent clevidipine was a better antihypertensive agent than SNP or TNG and was equivalent to nicardipine.61 Magnesium has coronary artery vasodilating properties, reduces the size of myocardial infarction in the setting of acute ischemia, and decreases mortality associated with infarction.62 In addition, it is an antiarrhythmic and minimizes myocardial reperfusion injury. While magnesium was found to prevent atrial fibrillation in coronary artery surgery,63 in patients treated with β-blockers, the addition of prophylactic iv magnesium did not reduce the incidence of atrial arrhythmias.64
The most recent guidelines for CABG surgery have been published in 2011.65 They recommend (i) volatile agent–based anesthetic aimed at early tracheal extubation; (ii) adequate perioperative analgesia; (iii) anesthetic care by a fellowship-trained or experienced, board-certified, TEE-trained anesthesiologist; (iv) utilization of intraoperative TEE for evaluation of acute, persistent, and life-threatening hemodynamic changes, for monitoring of ventricular function and regional wall motion abnormalities, and for concomitant valvular surgery; (v) management that augments the coronary arterial perfusion pressure to reduce the risk of perioperative myocardial ischemia and infarction; (vi) administration of β-blockers to reduce the incidence (or complications) of atrial fibrillation and cardiac mortality (particularly in patients with LVEF >30%); (vii) administration of angiotensin-converting enzyme (ACE) inhibitors or angiotensin receptor blockers perioperatively; (viii) selective use of PAC; and (ix) multimodal approach for management of perioperative bleeding and transfusion, based on use of lysine analogues, point-of-care testing, discontinuation of antiplatelet medications for at least 5 days preoperatively, among others. The reader is encouraged to consult the full document at http://circ.ahajournals.org.easyaccess1.lib.cuhk.edu.hk/content/124/23/e652.
Valvular Heart Disease
Alterations in loading conditions are the initial physiologic burdens imposed by valvular heart lesions, both stenotic and
regurgitant. For example, the LV is pressure overloaded in aortic stenosis (AS) and volume overloaded in aortic insufficiency and MR. In mitral stenosis, however, the LV is both volume underloaded and pressure underloaded, whereas the right ventricle (RV) faces progressively increasing left atrial and pulmonary artery pressure. Compensatory mechanisms consist of chamber enlargement, myocardial hypertrophy, and variations in vascular tone and level of sympathetic activity. These mechanisms in turn induce secondary alterations, including altered ventricular compliance, development of myocardial ischemia, chronic cardiac dysrhythmias, and progressive myocardial dysfunction.
regurgitant. For example, the LV is pressure overloaded in aortic stenosis (AS) and volume overloaded in aortic insufficiency and MR. In mitral stenosis, however, the LV is both volume underloaded and pressure underloaded, whereas the right ventricle (RV) faces progressively increasing left atrial and pulmonary artery pressure. Compensatory mechanisms consist of chamber enlargement, myocardial hypertrophy, and variations in vascular tone and level of sympathetic activity. These mechanisms in turn induce secondary alterations, including altered ventricular compliance, development of myocardial ischemia, chronic cardiac dysrhythmias, and progressive myocardial dysfunction.
Myocardial contractility in patients with mitral insufficiency is often transiently depressed but may progress to irreversible impairment even in the absence of clinical symptoms. Conversely, the patient with AS may complain of dyspnea, not because of impaired systolic function, but because of reduced ventricular compliance, increased left ventricular end-diastolic pressure, and pulmonary vascular congestion.
The patient presenting for valve repair or replacement often has pulmonary hypertension, severe ventricular dysfunction, and chronic rhythm disorders. Anesthetic management is predicated on understanding the altered loading conditions, preserving the compensatory mechanisms, maintaining circulatory homeostasis, and anticipating problems that may arise during and after valve surgery. In this section, we briefly describe the pathophysiology, desirable hemodynamic profile, and other pertinent anesthetic considerations for each valvular lesion.
TEE has become the standard of care in the perioperative management of patients undergoing valve surgery. TEE can further refine the preoperative diagnosis, identify valvular pathology and the mechanism of disease, and quantify the degree of stenosis and/or regurgitation. A detailed review of the perioperative role of TEE is presented in Chapter 26.
Aortic Stenosis
AS is the most common valvular disease in the United States. In a normal adult, the AV is composed of three semilunar cusps attached to the wall of the aorta. The normal AV diameter is 1.9 to 2.3 cm with an AV area of 2 to 4 cm2. The AV cusps are suspended from symmetric outpouchings of the aortic wall that are called the sinuses of Valsalva. The AV cusps and the corresponding sinuses are named according to their relation to the coronary ostia: Left, right, and noncoronary (opposite the interatrial septum). On the ventricular side of the AV is the oval-shaped LV outflow tract (LVOT). Its borders are the inferior surface of the anterior leaflet of the mitral valve, the interventricular septum, and the LV free wall. The normal diameter of the LVOT is 2.2 ± 0.2 cm.
Calcific AV disease has many similar features with coronary artery disease. What in the past was thought to be “degenerative” is a disease continuum, similar to atherosclerosis. Increased mechanical stress (higher on the aortic side of AV cusps, in the flexion area) causes endothelial disruption, which leads to lipoprotein deposition, chronic inflammation, and active cusp calcification.66 These histologic changes result in macroscopic, progressive valve thickening. Increased calcification eventually leads to leaflet immobility and outflow obstruction. Clinical factors associated with aortic sclerosis include older age, male gender, smoking, hypertension, and hyperlipidemia. Patients with bicuspid AV (increased mechanical stress) or with altered mineral metabolism (Paget disease, renal failure) have a higher prevalence of calcific AS disease. Rheumatic disease (an autoimmune disease, rarely seen in developed countries, leading to calcification and fusion along the commissures) causes mixed AS and AV regurgitation and usually coexists with mitral valve disease.
Pathophysiology

The costs of the concentric hypertrophy are decreased diastolic compliance and a precarious balance between myocardial oxygen supply and M[V with dot above]O2. Hypertrophy-induced impairment of diastolic relaxation (“stiff” LV) impedes early LV filling, and LA contraction becomes critical for maintaining adequate ventricular filling and subsequent stroke volume. In AS, the “atrial kick” may account for up to 30% to 40% of LV end-diastolic volume. The ventricular filling pressure, as reflected by pulmonary capillary wedge pressure, may vary widely with only small changes in ventricular volume (reduced compliance).
The hypertrophied muscle mass has increased basal M[V with dot above]O2, while demand per beat rises because of the elevated intraventricular systolic pressure. Because the capillary density is often inadequate for the hypertrophic muscle, a reduction in perfusion pressure (as when the aortic diastolic pressure is decreased and/or the ventricular filling pressure is increased) may compromise supply and total vasodilator reserve. This situation is compounded in the presence of coronary obstruction. Patients with AS often present with heart failure.
There is an inverse relationship between wall stress (afterload) and LV ejection fraction. In patients with a substantial transvalvular pressure gradient (mean >40 mm Hg), AV replacement corrects the afterload excess and improves outcome. Decreased contractility results in decreased stroke volume and a hemodynamically (and echocardiographically) decreased pressure gradient (<30 mm Hg: “Pseudo-AS” or “low-gradient AS”) despite the echocardiographic presence of AS. The response to pharmacologic intervention with dobutamine or nitroprusside will clarify the diagnosis: If the calculated AV area does not change there is true AS (therefore, AV replacement is indicated), if the stroke volume increases well out of proportion to the increase in valvular gradient there is relative AS, while little or no increase in stroke volume is diagnostic of severe LV dysfunction that carries increased operative risk. However, surgical intervention has better long term than medical management alone.68
Anesthetic Considerations
The ideal hemodynamic environment for the patient with AS is summarized in Table 38-3. Noncardiac surgery in patients with asymptomatic severe AS may not be associated with complications (one death in 23 patients with general anesthetic, no death in 25 patients with local anesthetic), provided that their hemodynamics are invasively monitored.69 Subsequent larger studies in these patients present conflicting results. Some show a marked increase in perioperative death and infarction rates and others do not.70,71 The reasons for these differences are not clear, but maintenance of adequate ventricular volume and sinus rhythm is crucial.
Hypotension must be prevented and treated promptly if it develops. Anticipation of likely hemodynamic changes is essential (e.g., expected decreases in blood pressure following spinal or epidural anesthesia); treatment should be immediate. Coronary perfusion pressure must be maintained to prevent the vicious cycle of hypotension-induced ischemia, subsequent ventricular dysfunction, and worsening hypotension. Bradycardia is a common clinical cause for hypotension in the patient with AS. Slowing the heart rate and increasing diastolic time will not increase stroke volume in the LV with concentric hypertrophy. Therefore, bradycardia will induce a fall in total cardiac output and systemic arterial pressure. This is especially pertinent in the elderly or diabetic patient, in whom sinus node disease and reduced sympathetic responses may predispose to significant bradycardia. Tachycardia must be avoided because it reduces the duration of diastolic coronary perfusion.
Hypotension must be prevented and treated promptly if it develops. Anticipation of likely hemodynamic changes is essential (e.g., expected decreases in blood pressure following spinal or epidural anesthesia); treatment should be immediate. Coronary perfusion pressure must be maintained to prevent the vicious cycle of hypotension-induced ischemia, subsequent ventricular dysfunction, and worsening hypotension. Bradycardia is a common clinical cause for hypotension in the patient with AS. Slowing the heart rate and increasing diastolic time will not increase stroke volume in the LV with concentric hypertrophy. Therefore, bradycardia will induce a fall in total cardiac output and systemic arterial pressure. This is especially pertinent in the elderly or diabetic patient, in whom sinus node disease and reduced sympathetic responses may predispose to significant bradycardia. Tachycardia must be avoided because it reduces the duration of diastolic coronary perfusion.
Table 38-3. Aortic Stenosis—Hemodynamic Goals | ||||||||||||||||
---|---|---|---|---|---|---|---|---|---|---|---|---|---|---|---|---|
|
Ischemia may be difficult to detect because the characteristic electrocardiographic changes are often obscured by signs of LV hypertrophy and strain. Elevated LV filling pressures, although not necessarily reflecting increased volume, often require treatment to optimize coronary perfusion pressure.68 TNG is useful in this regard, but it must be remembered that minimal reductions in ventricular volume are required. Therefore, very low doses of TNG should be used and titrated to effect. In the presence of LV dysfunction, an arterial dilator, such as nicardipine (preferred over SNP),72 should be carefully titrated to lower afterload without affecting ventricular volume. The utility of TEE in diagnosing and grading the severity of AS is described in Chapter 26.
Hypertrophic Cardiomyopathy
Hypertrophic cardiomyopathy is a genetically determined disease characterized by histologically abnormal myocytes and myocardial hypertrophy developing a priori, in the absence of a pressure or volume overload. The LV has a relatively small cavity relative to the wall thickness and is hyperdynamic.73
Pathophysiology
The physiologic consequences of hypertrophic cardiomyopathy are similar to those detailed for AS and are depicted in Figure 38-5. A subset of patients (20% to 30%) have some degree of subvalvular obstruction (hypertrophic obstructive cardiomyopathy), which may result in an LVOT gradient from a combination of anatomic
(systolic septal bulging into the LVOT, malposition of the anterior papillary muscle) and functional (drag forces, and hyperdynamic LV contraction causing a Venturi effect) factors. The LVOT gradient is dynamic in nature, increased by any intervention that reduces the LV size (increased contractility and heart rate or decreased preload or afterload). As blood is ejected rapidly through this area, the anterior mitral valve leaflet is pulled closer to the septum (systolic anterior motion), resulting in a variable MR jet, which, in the absence of organic mitral disease, is directed posteriorly.74
(systolic septal bulging into the LVOT, malposition of the anterior papillary muscle) and functional (drag forces, and hyperdynamic LV contraction causing a Venturi effect) factors. The LVOT gradient is dynamic in nature, increased by any intervention that reduces the LV size (increased contractility and heart rate or decreased preload or afterload). As blood is ejected rapidly through this area, the anterior mitral valve leaflet is pulled closer to the septum (systolic anterior motion), resulting in a variable MR jet, which, in the absence of organic mitral disease, is directed posteriorly.74
![]() Figure 38.5. Pathophysiology of primary left ventricular (LV) hypertrophy in hypertrophic cardiomyopathy. BP, blood pressure. |
In hypertrophic cardiomyopathy the myocardial oxygen balance is tenuous, and angina during exercise occurs even in the absence of coronary artery disease, if the coronary blood flow is unable to meet the demands of the hypertrophied myocardium.
Anesthetic Considerations
Treatment options for hypertrophic cardiomyopathy include pharmacologic “thinning” of the proximal interventricular septum with intracoronary alcohol injection (provided that the diastolic septal thickness at the site of injection is <15 mm), cardiac pacing, and surgical septal myectomy that is aimed to decrease the flow gradient (target is <30 mm Hg at rest or <50 mm Hg during exercise). The anesthetic management of patients with hypertrophic cardiomyopathy focuses on maintaining ventricular filling and avoiding the factors predisposing to outflow tract obstruction or ischemia (Table 38-4). Myocardial depression is desirable, and volatile anesthetics are useful. Because of the dependence of preload on atrial contraction, control of atrial rate and rhythm is mandatory. If junctional rhythm occurs, these patients may need atrial pacing with a transesophageal pacing probe or a PAC with pacing capability.
Table 38-4. Hypertrophic Cardiomyopathy—Hemodynamic Goals | ||||||||||||||||
---|---|---|---|---|---|---|---|---|---|---|---|---|---|---|---|---|
|
Although infrequent, hypertrophic cardiomyopathy occasionally coexists with valvular AS and may explain unanticipated difficulties in separating from bypass or unexpected increased pressure gradient following a seemingly uncomplicated AV replacement. A careful TEE examination of the LVOT area proximal to the newly replaced AV will resolve this dilemma. A dynamic left ventricular outflow obstruction may result following mitral valve repair, if an elongated anterior mitral leaflet is brought closer to the interventricular septum (anterior septal motion observed echocardiographically). In such cases, hypotension should be treated with volume replacement and vasoconstrictors, not inotropes.
Aortic Insufficiency
Aortic valve insufficiency (AI) is the result of annular dilatation or structural AV cusp lesions. Annular dilatation occurs with aneurysms (Marfan syndrome) or due to dissection of the ascending aorta. Calcific degeneration, rheumatic disease, bicuspid AV, endocarditis, trauma, or a jet lesion due to dynamic or fixed subvalvular stenosis results in abnormal leaflet motion and loss of coaptation and causes AI. Acute AI is caused by bacterial endocarditis, aortic dissection, or trauma.
Pathophysiology

contrast to AS, considerable alterations in LV volume can occur with only minimal changes in LV filling pressure. Although the LV may pump more than twice the normal cardiac output, M[V with dot above]O2 does not increase extraordinarily because the oxygen cost for muscle shortening (volume work) is low. The diastolic runoff and the moderate vasodilation reduce the LV afterload and increase the arterial pulse pressure. Thus, patients may be relatively symptom-free even when contractility is reduced. This is important in terms of planning the anesthetic, but perhaps even more so with respect to the timing of AV replacement. Ideally, the valve should be replaced just prior to the onset of irreversible myocardial damage. The outcome is better in patients with LV ejection fraction >55% or an end-diastolic LV diameter <55 mm.75 Therefore, continued follow-up of these patients emphasizes repeated noninvasive estimations of contractility, usually after some form of afterload stress, either pharmacologic-induced or exercise-induced.
![]() Figure 38.6. Pathophysiology of aortic insufficiency. LVEDP, left ventricular end-diastolic pressure; LV, left ventricle; ART, arterial. |
In acute AI, the previously normal in size and compliance LV is faced with a large end-diastolic volume, and the diastolic aortic to LV pressure gradient is acutely decreased.76 As a result, the LV end-diastolic pressure rises rapidly (along the steep portion of the diastolic pressure–volume relation), the myocardial perfusion gradient is decreased, and myocardial contractility becomes impaired with severe congestive heart failure as the cardinal clinical sign. Tachycardia and peripheral vasoconstriction are the compensatory mechanisms, but they further increase the myocardial oxygen needs. In acutely ill patients, emergent AV replacement is required, whereas in less severe circumstances, mild systemic vasodilation and inotropic support may return hemodynamics toward normal.
Anesthetic Considerations

Table 38-5. Aortic Insufficiency—Hemodynamic Goals | ||||||||||||||||
---|---|---|---|---|---|---|---|---|---|---|---|---|---|---|---|---|
|
Ventricular distention may occur with the onset of CPB if the heart rate slows or if there is unexpected ventricular fibrillation (ineffective systolic ejection). Monitoring of heart size, rate, rhythm, and ventricular filling pressure is especially important in these patients. If LV distention occurs, insertion of an LV vent or immediate cross-clamping of the aorta should alleviate the problem. The presence of moderate-to-severe AI will affect the approach to CPB. After application of the aortic cross-clamp, cardioplegic solution is normally injected into the aortic root, delivering this solution to the coronary system, producing diastolic arrest of the heart. An incompetent AV prevents delivery of cardioplegia to the coronary system. Instead, cardioplegia will fill and distend the LV, increasing the ischemic insult incurred during CPB, and diastolic arrest of the heart becomes difficult. As a result, in the presence of AI the heart is arrested by injecting cardioplegia directly into the coronary ostia (after aortotomy) or into the coronary sinus (“retrograde”).
Mitral Stenosis
Mitral valve stenosis (MS), caused by rheumatic fever, is rare in the United States. MS develops from leaflet thickening, commissural fusion, and chordal shortening and fusion due to chronic inflammation. The most frequent cause of MS is atherosclerosis-associated mitral annular calcification and endocarditis.77
Pathophysiology


where flow is cardiac output/diastolic filling time; pressure gradient is the difference between LAP and LVDP; and K is a hydraulic pressure constant (this calculation assumes no regurgitant flow). At a constant MVA, rearrangement of the Gorlin formula reveals the clinical variables determining the elevated LAP in MS:

Therefore, increased cardiac output or decreased diastolic filling period results in increased LAP by the square of the original changes. This explains why tachycardia or increased forward flow, seen classically with pregnancy, thyrotoxicosis, or infection, can precipitate pulmonary edema in a patient with MS. Thus, the development of atrial fibrillation causes hemodynamic deterioration, not so much because of the loss of atrial contraction and associated decrease in preload, but because of the rapid rate, which by decreasing the diastolic filling time increases the LAP pressure even more. Upstream from the LA, the persistently elevated LAP, which eventually leads to LA dilatation (and atrial fibrillation, usually the first manifestation of MS), is reflected through the pulmonary circulation (pulmonary congestion and increased work of breathing), leading to right ventricular pressure overload with compensatory right ventricular hypertrophy. The progression and severity of pulmonary hypertension is variable; further narrowing of the valve orifice causes irreversible reactive changes in the pulmonary vasculature (rales on auscultation, hemoptysis). Once pulmonary hypertension has developed the
operative risk is increased (12% vs. 3% to 8%).79 Right ventricular dysfunction, tricuspid annular dilatation, and insufficiency (engorged neck veins) may develop as the right heart function worsens.
operative risk is increased (12% vs. 3% to 8%).79 Right ventricular dysfunction, tricuspid annular dilatation, and insufficiency (engorged neck veins) may develop as the right heart function worsens.
![]() Figure 38.7. Pathophysiology of mitral stenosis. RV, right ventricle; PA, pulmonary artery; LA, left atrium; AFib, atrial fibrillation; LV, left ventricle. |
MS mimics left heart failure, with pulmonary congestion and decreased forward flow. The only definitive treatment is relief of obstruction with mitral valve replacement; balloon valvuloplasty and open commissurotomy are palliative maneuvers. The medical interventions should aim at decreasing the heart rate (with β- or calcium channel blockers) or treating the cause(s) responsible for the increased transmitral flow. The pulmonary capillary wedge pressure is higher than the true LVDP at least by the amount of the pressure gradient and can be used as a relative index of LV filling, even during episodes of tachycardia or increased flow.
Anesthetic Considerations

Treatment of hypotension in patients with MS can present a challenging dilemma. Although these patients normally take diuretics, hypovolemia is not usually the cause; hence, the response to volume administration is often disappointing. Use of a vasoconstrictor to offset mild peripheral vasodilation is acceptable, bearing in mind the effect of pulmonary vasoconstriction on right ventricular function. It is often prudent to select a drug with some inotropic effect such as ephedrine or epinephrine instead of relying on a pure vasoconstrictor, such as phenylephrine. In separating from CPB, attention is on avoiding right ventricular failure (discussed subsequently); more commonly, however, there is LV dysfunction. This may be because of intraoperative injury or sudden increase in flow to, and distention of, the chronically underloaded LV. After bypass, prominent v waves may be present in the pulmonary capillary wedge pressure waveform. This almost always reflects increased LV filling rather than MR provided that the cardiac output is increased when compared with preinduction values. The echocardiographic evaluation of MS is described in Chapter 26.
Table 38-6. Mitral Stenosis—Hemodynamic Goals | ||||||||||||||||||
---|---|---|---|---|---|---|---|---|---|---|---|---|---|---|---|---|---|---|
|
Mitral Regurgitation
In MR the valve is incompetent during systole and blood regurgitates from the LV to the LA. Etiologies of MR include excessive motion of one or both leaflets, prolapse or flail due to degenerative disease or ruptured chordae tendineae following acute myocardial infarction, and restricted leaflet motion due to rheumatic heart disease or myocardial ischemia or leaflet perforation from endocarditis. In functional MR the leaflet structure is normal, but the valve is incompetent due to either decreased LV systolic pressure (ischemic cardiomyopathy) that fails to “close” the leaflets or a dilated annulus (the fiber ring from where the leaflets are suspended) that decreases the coaptation surface of the leaflets (Table 38-7).
Pathophysiology

enlargement (due to “back-and-from” blood flow), ventricular wall hypertrophy (to compensate for the increased chamber size), and increased blood volume. The LV compliance is increased so that the large LV end-diastolic volume does not cause striking increase in pressure. There is no concomitant increase in oxygen requirements because there is little pressure development. As a result, patients may have minimal symptoms as well as normal or slightly reduced ejection fraction, despite progressive myocardial dysfunction and decreased contractility The regurgitant blood volume is related to the size of the regurgitant mitral orifice, the systolic time, the pressure gradient across the valve, and the compliance of the LA. The regurgitant orifice size, in turn, depends on the valvular defect and ventricular size. Therefore, increases in heart rate, reduction of preload, and arterial dilators are effective in reducing the regurgitation of blood volume from the LV to LA.
Table 38-7. Mechanisms of Mitral Regurgitation | |||||||||||||||||||||||||||||||||||||||
---|---|---|---|---|---|---|---|---|---|---|---|---|---|---|---|---|---|---|---|---|---|---|---|---|---|---|---|---|---|---|---|---|---|---|---|---|---|---|---|
|
Repairing or replacing the mitral valve increases the LV afterload and unmasks any underlying myocardial dysfunction. Administration of inotropes and/or vasodilators, as well as judicious increase in preload, may be necessary to successfully separate from bypass.
Table 38-8. Mitral Regurgitation—Hemodynamic Goals | ||||||||||||||||
---|---|---|---|---|---|---|---|---|---|---|---|---|---|---|---|---|
|
Acute-onset MR has a different hemodynamic picture; acute LA and LV volume overload occurs in the absence of compensatory LV enlargement. Ventricular filling pressures increase dramatically, as do pulmonary pressures. Cardiac output decreases, and pulmonary edema develops. In the setting of acute myocardial infarction, contractility may be inadequate despite pharmacologic support, and intra-aortic balloon assistance and emergency surgery may be lifesaving.
Anesthetic Considerations

Aortic Diseases
Acquired (hypertension, inflammation, deceleration trauma, or iatrogenic trauma) and genetic (connective tissue disorders, bicuspid AV) conditions are the cause of aortic diseases: Aortic
dissection, intramural hematoma, and aortic aneurysm. Weakening the media layers of the aorta (the term cystic medial degeneration denotes the disappearance of smooth muscle cells and the degeneration of elastic fibers) leads to higher wall stress, which induces dilatation and aneurysm formation, eventually resulting in intramural hemorrhage, aortic dissection, or rupture.
dissection, intramural hematoma, and aortic aneurysm. Weakening the media layers of the aorta (the term cystic medial degeneration denotes the disappearance of smooth muscle cells and the degeneration of elastic fibers) leads to higher wall stress, which induces dilatation and aneurysm formation, eventually resulting in intramural hemorrhage, aortic dissection, or rupture.
Aortic Dissection
Aortic dissection80 is part of the acute aortic syndrome that includes aortic dissection, intramural hematoma, and penetrating ulcer. Aortic dissection affects mostly men (7/3 ratio) and whites (4/1 ratio), while connective tissue disorders, such as Marfan syndrome and Ehlers-Danlos syndrome affect mostly the young (age <40 years). Hypertension is the most common risk factor in older patients. Aortic dissection is caused by a tear in the aortic intima and media, which propagates proximal and distally, creating a false lumen within the aortic media. The dissection often involves aortic branches and causes poor perfusion of vital organs (brain, spinal cord, abdominal organs), cardiac tamponade, or AI. Acute aortic dissection of the ascending aorta (type A) is highly lethal, with a mortality rate of 1% to 2% per hour after onset of symptomatology and is a true surgical emergency. An aortic dissection distal to the left subclavian artery is called type B, has a 30-day mortality of 10%, and may be managed medically or with placement of a scaffold (stent). Intramural hematoma is considered a precursor to classic dissection and usually originates from ruptured vasa vasorum in the media. Intramural hematoma has the same prognosis as aortic dissection and is treated similarly.
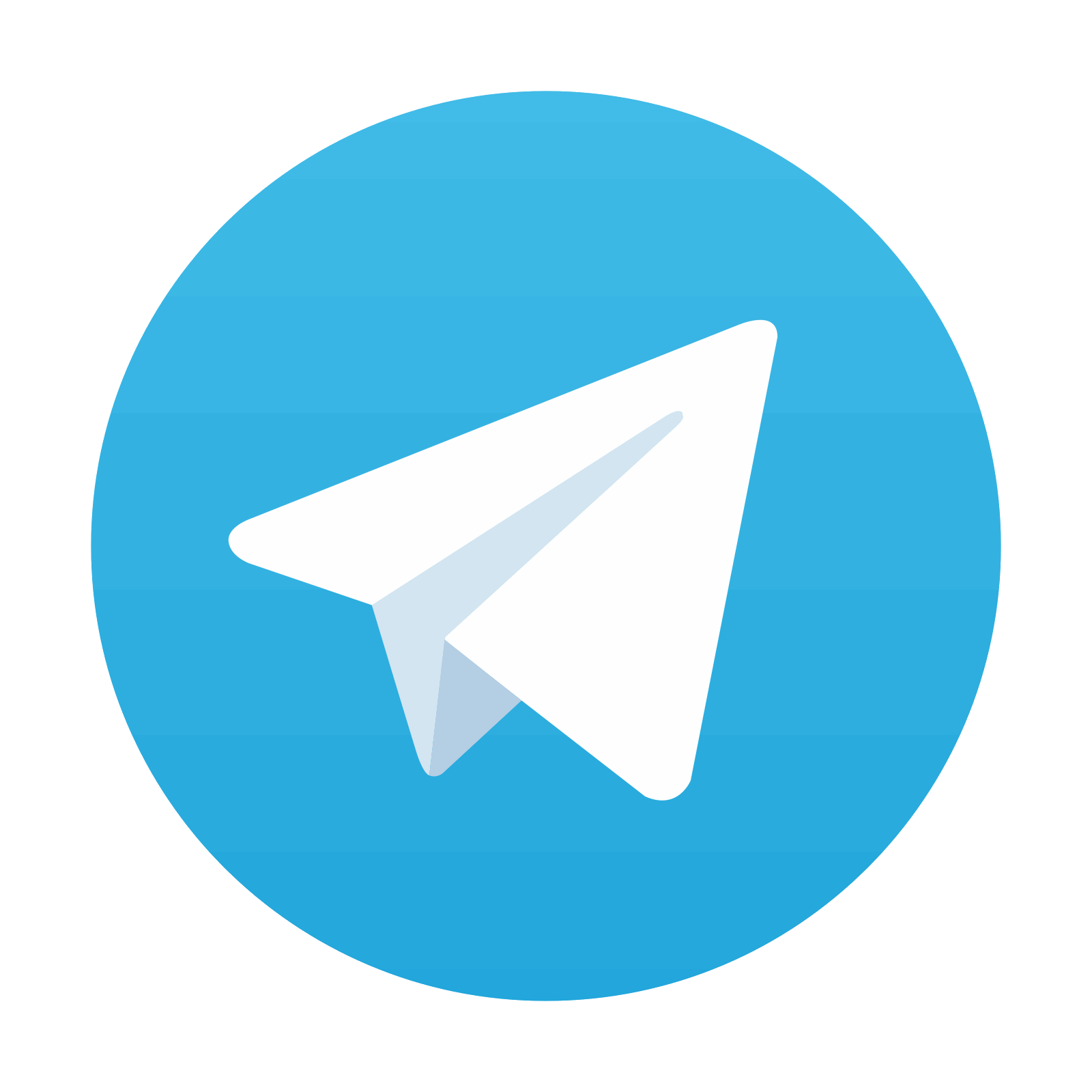
Stay updated, free articles. Join our Telegram channel

Full access? Get Clinical Tree
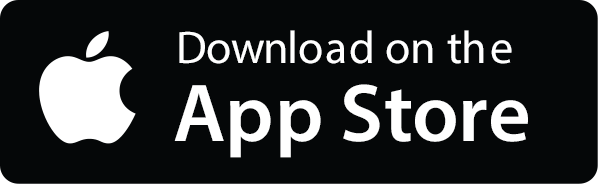
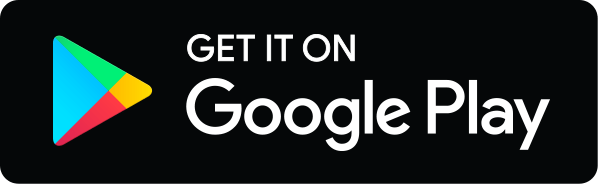