Dahlem et al. [34]
Zimmerman et al. [159]
Bindl et al.
[17]
Kneyber et al. [80]
Hu et al. [72]
Yu et al. [156]
Lopez-Fernandez et al. [95]
Study period
2 years (1998–1999)
1 year (1999–2000)
3 months (1997, 2001, 2004)
1 year (2004–2005)
2 years (2004–2006)
1 year (2005–2006)
1 year (2004–2005)
1 year (2010–2001)
Country
The Netherlands
The USA
Germany
Australia, New Zealand
The Netherlands
China
China
Spain
Design
Prospective
Prospective
Survey
Prospective
Retrospective
Prospective
Prospective
Prospective
Sites
Single centre
Multicentre
Multicentre
Multicentre
Single centre
Multicentre
Multicentre
Multicentre
Inclusion
NAECC + MV
NAECC + MV
NAECC + MV
NAECC + MV
NAECC
NAECC
NAECC + MV
PaO2/FiO2
≤ 200
≤ 200
≤ 200
≤ 200
≤ 200
Unknown
≤ 200
≤ 200
Age
0–18 years
½–15 years
1 month–18 years
< 16 years
< 18 years
1 month–15 years
1 month–15 years
1 month–15 years
Incidence (per 100,000 per year)
Not reported
9.5
3.2
2.6
2.2
Not reported
Not reported
3.9
Prevalence (percentage of PICU admissions
Not reported
Not reported
Not reported
1.9
3.4
2.6
1.4
1.4
PARDS is associated with significant but at the same time variable mortality rates. Numerous small and large observational and epidemiological studies of children with PARDS indicate mortality rates of 15–75% [126]. Mortality rates may be the lowest in children without comorbidities [95]. A recent systematic review of all published PARDS studies suggested that the mortality was higher in non-Western countries [126]. Remarkably, there appeared to be a decrease in mortality rates over time, especially when paediatric data reported before 1994 was taken into account (Fig. 19.1) [126]. The possible decrease in mortality may be explained by differences in patient population, changes in ventilatory management of children with PARDS over time or institutional variation in management of PARDS. For instance, some group of investigators reported relatively low mortality rates when lung-protective ventilation (LPV) strategies were applied [6, 116]. Furthermore, the use of high-frequency oscillatory ventilation (HFOV) is much more common in the paediatric population, potentially biasing the observations on mortality [7, 11, 95].
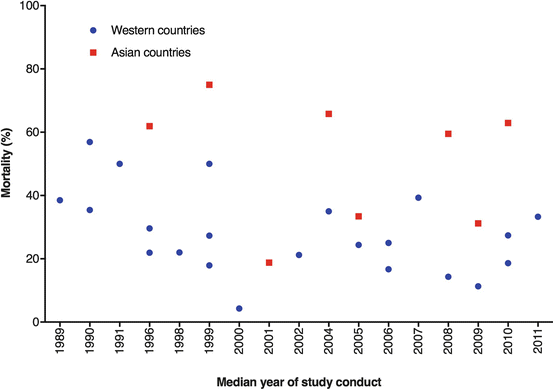
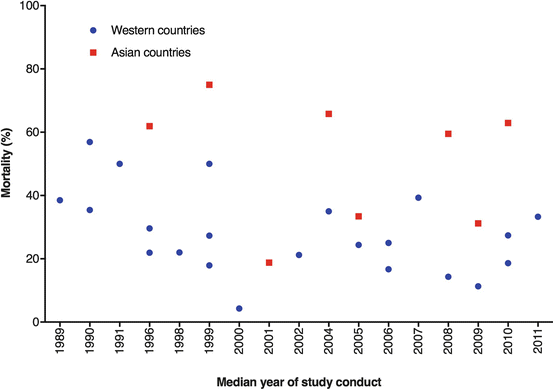
Fig. 19.1
Summary of mortality rates as function of time in children with acute respiratory distress syndrome (ARDS)
Various risk factors predicting mortality during the acute phase of PARDS have been identified, including the presence of immunodeficiency, non-pulmonary sepsis, older age, H1N1 influenza or multiple organ failure upon diagnosis of PARDS [50, 120]. The highest mortality rates (e.g. over 90%) have been reported in children with haematologic malignancies and disseminated intravascular coagulation or who have undergone bone marrow transplant [19, 42, 74]. Investigators have also observed that the degree of oxygenation impairment was associated with increased mortality in various patient cohorts [120]. Although predictive for patient outcome, commonly used metrics of oxygenation such as the PaO2/FiO2 ratio, oxygenation index, alveolar–arterial oxygen gradient, arterial–alveolar oxygen ratio and paediatric lung injury score necessitate arterial PaO2. Alternatively, observational studies have shown that provided SpO2 is less than 98%; the SpO2/FiO2 ratio, oxygen saturation index (OSI) and non-invasive paediatric lung injury score are validated non-invasive scores to estimate patient outcome [77, 79, 141]. Others have suggested that patient outcome may also be predicted by the end-tidal alveolar dead space fraction or the dead space to tidal volume ratio in PARDS [103] [58].
The role of biomarkers in predicting disease severity and outcome such as epithelial markers such as KL-6 and surfactant protein D, endothelial markers such as von Willebrand factor and Ang-2, coagulation and fibrinolysis markers and markers for inflammation is little studied and therefore remains inconclusive [104].
19.3 A New Definition for ARDS in Children
In 2015, the Pediatric Acute Lung Injury Consensus Conference (PALICC) developed a paediatric-specific definition for ARDS in children. This initiative was fuelled by the lack of a paediatric context in both the original NAECC definition of ARDS and its revised definition, the Berlin definition. By purely focusing on lung injury in adults, differences between children and adults related to risk factors, aetiologies and pathophysiology most likely have been overlooked.
The new definition of PARDS (Table 19.2) mirrors the Berlin definition in two ways, being timing of onset and exclusion of patients with cardiac failure or fluid overload that may explain the respiratory failure. Importantly, paediatric patients with causes of acute hypoxaemia that are unique to the perinatal period such as prematurity-related lung disease, perinatal lung injury (e.g. meconium aspiration syndrome, pneumonia and sepsis acquired during delivery) or other congenital abnormalities (e.g. congenital diaphragmatic hernia or alveolar capillary dysplasia) were excluded from the definition. As in adults, in order to qualify for PARDS, the symptoms of hypoxaemia and radiographic changes have to occur within 7 days of a known clinical insult. Noticeably, children with left ventricular heart dysfunction or congenital heart disease that fulfil all other PARDS criteria have PARDS if the acute hypoxaemia and new chest imaging changes cannot be explained by acute left ventricular heart failure or fluid overload. This is a huge improvement of the new PARDS definition over other pre-existing definitions.
Table 19.2
Definition of paediatric acute respiratory distress syndrome (PARDS) according to the Pediatric Acute Lung Injury Consensus Conference (PALICC)
Age | Exclude patients with perinatal-related lung disease | |||
Timing | Within 7 days of known clinical insult | |||
Origin of oedema | Respiratory failure not fully explained by cardiac failure or fluid overload | |||
Chest imaging | Chest imaging findings of new infiltrate(s) consistent with acute pulmonary parenchymal disease | |||
Oxygenation | Invasive mechanical ventilation | Non-invasive mechanical ventilation | ||
Mild | Moderate | Severe | PARDS (no severity stratification) | |
4 ≤ OI < 8 5 ≤ OSI < 7.51 | 8 ≤ OI < 16 7.5 ≤ OSI < 12.31 | OI ≥ 16 OSI ≥ 12.31 | Full face-mask bi-level ventilation or CPAP ≥5 cmH20 PF ratio ≤ 300 SF ratio ≤ 2641 | |
Special populations | ||||
Cyanotic heart disease | Standard criteria above for age, timing and origin of oedema with chest imaging with an acute deterioration in oxygenation not explained by underlying cardiac disease2 | |||
Chronic lung disease | Standard criteria above for age, timing and origin of oedema with chest imaging consistent with new infiltrate and acute deterioration in oxygenation from baseline which meet oxygenation criteria above3 | |||
Left ventricular dysfunction | Standard criteria for age, timing and origin of oedema with chest imaging changes consistent with new infiltrate and acute deterioration in oxygenation which meet criteria above not explained by left ventricular dysfunction |
There are two major differences between the Berlin definition and the PARDS definition; these are related to chest radiograph appearances and the metrics for oxygenation. Unlike the Berlin definition, diagnosis of PARDS requires the need for at least one consolidation on chest radiograph rather than mandating the need for bilateral consolidations. The rationale for this is the low sensitivity of chest radiography to detect (subtle) changes in consolidation, atelectasis or oedema and the poor correlation between consolidations on chest CT scanning and chest radiography [20, 66, 93] [24, 27, 37, 55]. In addition, the presence of bilateral infiltrates provided minimal predictive value for outcome in acute respiratory failure in both children and adults [76, 97, 98].
Aside from this, paediatric critical care practitioners also critiqued both definitions for including the PaO2/FiO2 ratio; this metric of oxygenation does not take ventilator settings or mode of ventilation (e.g. HFOV) into account. In addition, there is an increased use of pulse oximetry in children as a substitute for children without an indwelling arterial line, thereby creating an additional problem to the criteria requiring arterial blood gas measurement. Hence, the OI is incorporated in the PARDS definition. Based on a derivation set of two data sets from the USA and Australia/New Zealand, the following cut-off points were derived: OI 4–8 (mild PARDS), 8–16 (moderate PARDS) and > 16 (severe PARDS) [78]. Data from six other centres was used as a validation set, showing increased mortality with increasing PARDS severity, thereby supporting the substitution of OI for PaO2/FiO2. For patients without indwelling arterial lines, the oxygen saturation index (OSI) is used as an alternative provided that the SpO2 is 97% or less [78]. The OSI uses the SpO2 rather than the PaO2.
19.4 Pathobiology of Paediatric ARDS
ARDS can originate either from a direct pulmonary insult (i.e. pulmonary ARDS) such as a viral pneumonia or from extra-pulmonary insults such as sepsis (i.e. extra-pulmonary ARDS). The pathobiology of ARDS is a complex process, characterised by increased alveolo-capillary permeability, lung epithelial and endothelial injury and the accumulation of protein-rich oedema fluid during the acute phase of disease [146]. Surfactant homeostasis and haemostatic balance are altered. Much of the understanding of the pathobiology has come from clinical studies in adults or experimental models mimicking the adult clinical situation. Some of these underlying pathophysiological mechanisms are probably shared between children and adults, but it should also be appreciated that there are areas of significant difference including lung growth and development during (early) childhood, immune response and surfactant homeostasis. For instance, the lung parenchyma undergoes substantial structural remodelling and growth during childhood. In the neonatal period, the peripheral airspaces are subdivided by short and blunt tissue ridges into an increasing number of very shallow alveoli. It is by the age of 18 months that the lungs have developed into an adultlike structure [23]. After this period, the number of alveoli continues to grow through adolescence.
There are important differences in the immune response between infants and adults that are likely relevant [153]. In general, the immune system of a child < 1 year of age is relatively immature, including broad deficits in both innate and adaptive immunity [151]. Neonatal PMNs and monocytes function to a lesser degree compared with adults, with decreased chemotactic responses for as long as 1–2 years [54, 91, 100, 110]. Adaptive immunity is also different between young children and adults. At birth, the immune system has a strong T helper 2, anti-inflammatory predisposition with little ability of peripheral mononuclear cells to produce tumour necrosis factor alpha or other pro-inflammatory mediators compared with adults [13, 90]. In addition, at birth, T cells are biased towards regulatory T cells, thereby potentially suppressing the innate immune response [57, 157]. This anti-inflammatory predisposition may persist during early childhood [150]. Only a few studies have examined the role of immune responses in PARDS. Associations between elevated serum levels and interleukin (IL)-6 and PARDS and soluble intercellular adhesion molecule 1 with increased risk of death and/or prolonged duration of mechanical ventilation have been demonstrated in two small paediatric studies [44, 53]. Another small study showed an association of a genetic variant in the IL-1 receptor antagonist gene with ARDS in children with community-acquired pneumonia [114]. More recently, an association between markers of endothelial (angiopoetin 2) and pulmonary epithelial (soluble receptor for advanced glycation end products) injury and mortality in PARDS has been shown, especially in direct lung injury [152].
19.5 Mechanical Ventilation for Paediatric ARDS
Mechanical ventilation (MV) is clearly of benefit for children with ARDS although there are many questions that require study (Fig. 19.2) [81]. Both controlled and assisted modes of ventilation are used by paediatric critical care practitioners, irrespective of the size of the breath (pressure versus volume). However, superiority of one mode over the other has not been demonstrated [122]. This also applies to the use of ventilator modes that allow the patient to trigger the machine breath. Adult data has suggested that patient–ventilator asynchrony (i.e. no synchronisation between patient’s demand and the delivered breaths) is associated with increased morbidity, but paediatric data is lacking [40]. Neurally adjusted ventilatory assist (NAVA) uses the electrical activity signal of the diaphragm to trigger inspiration. So far, paediatric experience with this alternative mode of triggering is limited. Piastra and colleagues showed that infants recovering from severe ARDS had a shorter duration of respiratory support when they were weaned with NAVA compared with pressure support ventilation [118].
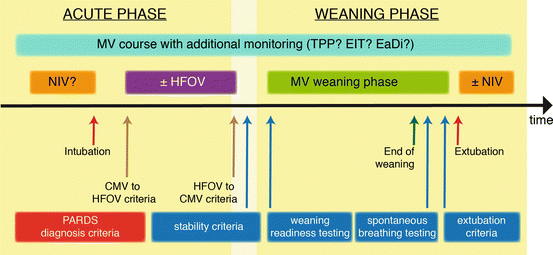
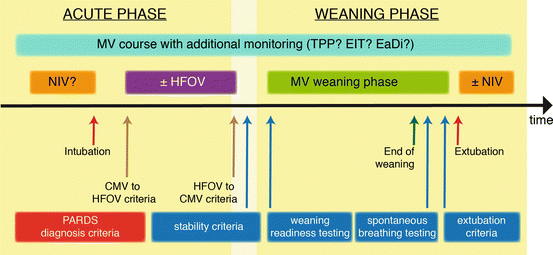
Fig. 19.2
Graphic summary of issues in the ventilatory management of paediatric acute respiratory distress syndrome (PARDS) (Reprinted with permission from Kneyber et al. [81])
MV may also exacerbate or even initiate lung injury and has therefore been identified as a predictor for poor outcome [131]. The development of this so-called ventilator-induced lung injury (VILI) has led to the concept of lung-protective ventilation (LPV). In brief, LPV encompasses the delivery of small tidal volume (Vt) to avoid end-inspiratory overdistension (i.e. volutrauma) and the application of a certain level of positive end-expiratory pressure (PEEP) to prevent repetitive opening and closure of alveoli (atelectrauma). The issue of LPV in PARDS is the subject of scientific debate. A large randomised controlled trial (RCT) in adult patients with ARDS according to the NAECC criteria showed improved patient outcome with 6 mL/kg ideal body weight (IBW) compared with 12 mL/kg/IBW [108]. However, other adult studies could not demonstrate a positive effect on mortality when 7 mL/kg IBW was compared with 10 mL/kg IBW [117]. Subsequent meta-analyses concluded that a Vt ≤ 10 mL/kg per predicted body weight was associated with higher survival [21, 22]. To date, a paediatric counterpart of the ARDS Network trial has not been performed. Remarkably however is the data coming from observational studies, showing an inverse or no relationship between Vt and mortality in children [46, 76](20) (Table 19.3). A systematic review of the available paediatric data could also not identify a specific threshold when Vt could be considered safe, irrespective of the presence of ARDS [39]. Experimental work has shown that very young animals were less susceptible to VILI compared with adult animals when subjected to injurious ventilation with supra-physiologic Vt (i.e. 30–40 mL/kg body weight) [84]. All of this challenges the role of volutrauma in PARDS. Various explanations may be proposed, including the incorrect measurement of Vt. The ventilator overestimates the Vt delivered to the patient in young children, signifying the need for correct measurement near the Y-piece of the patient circuit in children below 10–15 kg [25]. In addition, in adults, the Vt is calculated by predicted body weight for age, height and gender. In paediatric practice Vt is usually calculated by actual body weight. A paediatric counterpart of the ARDS Network trial is eagerly awaited, although such a trial seems very unlikely for various reasons including the need for a large sample size (i.e. > 500 patients) [82]. Interestingly, paediatric critical care practitioners may have already found the solution in targeting the optimal Vt in PARDS. Unlike in adult critical care, there is a large tendency to use pressure-controlled (PC) ventilation instead of volume-controlled (VC) ventilation. In contrast with the paediatric data showing an inverse relationship between tidal volume and mortality, these same studies did confirm an association between inspiratory pressures and mortality (Table 19.3). This is in line with the assumption that the patient with a more severe lung injury characterised by small residual inflatable lung volume available for alveolar ventilation and therefore gas exchange (which is also known as the baby lung concept) should receive tidal volumes below physiologic values [56]. Patient subgroup analysis from the ARDS Network trial showed that only patients with poor respiratory system compliance at study entry had a benefit in terms of survival when randomised to the 6 mL/kg study arm [41]. This observation suggests strongly that physicians tend to use lower Vt resulting in higher inspiratory airway pressures in the sicker patients (i.e. patients with lower respiratory system compliance) at the onset of ARDS and mechanical ventilation. This latter observation is in alignment with the data from the other paediatric observational study showing that patients with higher initial lung injury scores were ventilated by smaller tidal volumes and showed worse outcome [76]. This goes along with the concept of keeping lung tissue strain (the ratio between inflated volume and functional residual capacity) low in order to protect the lung [94]. Reanalysis of adult data also showed the importance of limiting the driving pressure (i.e. the ratio of Vt of compliance at a zero-flow state) in ARDS [30]. However, given the absence of paediatric data, no recommendation can be made on the upper limit of the plateau pressure that is allowable. The same applies to the level of PEEP. Levels of PEEP should be set to prevent lung unit collapse at expiration and to avoid tidal recruitment at each breath cycle (collapse–opening–recollapse injury). Although adult data indicated that higher levels of PEEP may be associated with lower mortality, such issues remain to be resolved in paediatrics. Remarkably, paediatric physicians tend to tolerate a higher level of FiO2 instead of turning up the PEEP because they worry about haemodynamic consequences.
Table 19.3
Summary of studies reporting on ventilation practices and outcomes in paediatric acute respiratory distress syndrome (PARDS)
Reference | Study design | Age (years) | Type of lung disease | N | PaO2/FiO2 ratio | Overall mortality (%) | Median Vt (mL/kg) | Findings |
---|---|---|---|---|---|---|---|---|
Flori [52] | Prospective, multicentre | <18 years | ALI (67% ARDS) | 320 | 161 ± 741 | 22% | 10 | No association between Vt and mortality |
Erickson [46] | Prospective, multicentre | <16 years | ALI | 117 | Not reported | 35% | 8.0 | High Vt associated with lower mortality. Higher mortality with high inspiratory pressures |
Albuali [6] | Retrospective, single centre Two periods | <17 years | ALI 79.2% ARDS) | 164 | 153 ± 59.9 (P1), 139.2 ± 53.1 (P2)1 | 28% | 10.2 ± 1.7 vs 8.1 ± 1.41 | High Vt associated with higher mortality after adjusting for disease severity, ventilator settings and use of HFOV. |
Khemani [75] | Retrospective, single centre | <18 years | AHRF (48% ALI/ARDS) | 389 | 138 (83–192)1 | 20% | 7 | High Vt associated with lower mortality. Higher mortality with high inspiratory pressures |
Hu [72] | Retrospective, multicentre | <16 years | AHRF (11.2% ALI, 66.3% ARDS) | 461 | 115 (76–168)1 | 41.6% (AHRF) 43.5% (ARDS) | 8.8 | No association between Vt and mortality at cut-off of 7 or 8 mL/kg |
Zhu [158] | Prospective, multicentre | <18 years | AHRF (92.3% ALI, 78.6% ARDS | 439 | 141 (106–192) | 18.5% (AHRF), 32.2% (ARDS) | 7.7 | No association between Vt and mortality in children > 1 year, lower mortality with higher Vt in children < 1 year |
From a theoretical perspective, high-frequency oscillatory ventilation (HFOV) is an ideal LPV mode [83]. With HFOV, a continuous distending pressure (CDP) is generated maintaining lung volume, with superimposed small oscillations in a frequency range of 5–15 Hz allowing for gas exchange. Paediatric critical care practitioners cherish HFOV despite the lack of scientific evidence. To date, there has been only one randomised controlled trial comparing high-frequency oscillatory ventilation to conventional mechanical ventilation in 70 children with diffuse alveolar disease and/or air leak syndrome [8]. This study showed that HFOV utilising an aggressive volume recruitment strategy resulted in a significant improvement in oxygenation and a decreased requirement for supplemental oxygen at 30 days. However, 30-day mortality was not changed in this particular trial. A meta-analysis of all six paediatric and adult clinical trials demonstrated improved mortality in patients randomised to HFOV [133]. However, two large randomised studies in adults with moderate-to-severe (early) ARDS troubled the discussion on HFOV in PARDS [49, 155]. Whereas in the OSCillation for ARDS (OSCAR) trial no difference in 30-day mortality was observed, and the OSCILLation for ARDS Treated Early (OSCILLATE) trial was prematurely stopped (after the 500 patient analyses) by the steering committee because of a significantly higher in-hospital (47% vs 35%) and 60-day mortality (47% vs 38%) in the HFOV group. In the absence of paediatric trials, a post hoc analysis of data of paediatric patients enrolled in a protocolised sedation trial showed similar mortality rates but prolonged duration of MV among patients managed with HFOV, calling for a paediatric RCT to examine the role of HFOV in PARDS [12].
Weaning from the ventilator should start as early as possible, although no paediatric data is available identifying a specific threshold when to start and how to do the weaning itself. Also, at present no paediatric criteria have been validated to discriminate extubation success and failure as well as commonly used adult parameters to monitor weaning including the rapid shallow breathing index (RSBI) [109]. The same applies to the application of spontaneous breathing trials (SBT) and extubation readiness test (ERT). It is unclear what the optimal SBT in PARDS is (i.e. using either continuous positive airway pressure (CPAP), a low level of pressure support ventilation or T-piece ventilation).
Non-invasive positive pressure ventilation (NPPV) is increasingly being used to treat respiratory failure from a variety of aetiologies in children, including paediatric ARDS [124]. Despite this growing, there is much uncertainty about the indications, strategies and effects on patient outcome for the use of NPPV, especially in paediatric ARDS [47, 128]. There are several paediatric prospective and retrospective cohort studies describing the use of NPPV for acute respiratory failure, but most include a heterogeneous population that includes mild-to-severe ARDS. Thus, it remains the subject of debate if NPPV actually prevents the need for endotracheal intubation. Observational studies indicated that the median intubation rate for children with severe PARDS is 57% [47]. In only one randomised controlled trial of 50 children with acute hypoxaemia respiratory failure, the intubation rate was significantly lower (28% vs 60%, p = 0.045) when NPPV was compared with standard care. However, this particular study included also patients with mild PARDS.
19.5.1 Extracorporeal Life Support
Extracorporeal life support (ECLS) is a modified form of cardiopulmonary bypass. During ECLS, blood is pumped through an extracorporeal circuit containing a membrane oxygenator in which O2 is added and CO2 removed from blood, which is then returned to the patient. An increasing number of paediatric patients with respiratory failure are managed with veno-venous or veno-arterial ECLS despite the absence of a great deal of data showing improved patient outcome [36]. No randomised trials have been performed in PARDS, making it difficult to determine the efficacy of ECLS. In one retrospective study, it was found that the use of ECLS was associated with a reduced mortality in paediatric acute respiratory failure [63]. Thus, ECLS should be considered in severe PARDS where the cause of the respiratory failure is believed to be reversible [36].
19.6 Pulmonary Specific Treatment of PARDS
The pathophysiologic and clinical features have triggered many investigators to study numerous pharmacological approaches to ARDS in critically ill adults [127]. Few of these approaches have been explored in critically ill children. As a consequence, much of the routine treatment for PARDS is based on data from adults or paediatric anecdotal experiences (Table 19.4). Despite this lack of scientific evidence, many pulmonary-specific therapies including inhaled nitric oxide (iNO), surfactant or steroids are used in daily practice [124, 125].
Table 19.4
Summary of studies reporting on the use of pulmonary specific ancillary treatment in paediatric acute respiratory distress syndrome (PARDS)
Reference | Number of patients Disease | Intervention | Findings and conclusions |
---|---|---|---|
Exogenous surfactant | |||
Willson et al. [22] | N = 42 Acute hypoxaemic respiratory failure (OI >7) | Calf surfactant vs air placebo | Improved oxygenation, decreased ventilation time and shorter PICU stays |
Willson et al. [24] | N = 153 Acute lung injury (OI > 7) | Calf surfactant vs air placebo | Improved oxygenation and decreased mortality. No difference in ventilator-free days (primary study outcome) |
Willson et al. [25] | N = 109 Direct lung injury only | Pneumosurf vs air placebo | No effect on oxygenation or mortality |
Thomas et al. [26] | N = 165 Acute hypoxaemic respiratory failure | Lucinactant (synthetic surfactant) vs air placebo | Improved oxygenation, but no effect on mortality, length of ventilation or length of stay |
Nitric oxide | |||
Day et al. [33] | N = 22 Acute bilateral lung disease | 10 ppm iNO vs control | Improved OI and PVRI, but not mortality |
Dobyns et al. [34] | N = 108 AHRF | 10 ppm iNO vs control | Improved OI and PVRI, but not mortality |
Ibrahim et al. [35] | N = 32 ARDS | 5 ppm iNO ± prone positioning vs control | Improved OI, but not mortality |
Prone positioning | |||
Curley et al. [73] | N = 102 Acute lung injury (PaO2/FiO2 ratio ≤ 300 mmHg) | Prone positioning for 20 hours vs standard therapy | Improved oxygenation, no effect on ventilator-free days or mortality |
19.6.1 Exogenous Surfactant
Animal studies, uncontrolled case reports and case series suggested a potential benefit to exogenous surfactant replacement therapy in PARDS [136]. However, clinical trials of exogenous surfactant outside of the neonatal population provided mixed results. Nonrandomised studies showed improved oxygenation but no effect on mortality in paediatric patients with moderate-to-severe ARDS according to the NAECC criteria. Calf lung surfactant (calfactant) was compared to placebo in a large, multicentre, randomised, placebo-controlled, masked trial in 153 children with acute lung injury [148]. Surfactant administration significantly improved oxygenation and reduced mortality (19% vs 36%) compared with placebo. However, this beneficial effect of surfactant disappeared after adjusting for immunocompromised state in multivariate analysis. The same group of investigators joined an international, multicentre, placebo-controlled trial comparing calfactant with placebo in both adult and paediatric patients with direct lung injury [149]. The trial utilised a novel form of surfactant, which was twice as concentrated as traditional calfactant. However, the trial was stopped prematurely due to futility. Recent attempts on identifying a possible role for exogenous surfactant through a multinational, prospective, blinded, randomised, controlled phase II trial of intratracheal instillation trial using a synthetic formulation of surfactant (lucinactant) among infants less than 2 years of age also showed no effect on patient outcome [140].
19.6.1.1 Nitric Oxide
The main effect of nitric oxide (NO) is smooth muscle relaxation by increasing intracellular cGMP. Vasodilation mainly occurs in areas that are adequately ventilated causing blood to shunt away from poorly ventilated areas [119]. Its use in ARDS may therefore be considered to reduce ventilation/perfusion mismatch by reducing dead space ventilation and, thereby, improving oxygenation. The clinical response to iNO has been reported in various (individual) case series, demonstrating a rapid improvement in oxygenation even with a concentration as low as 1 part per million (ppm) [1, 111, 129, 138]. Day and co-workers compared the effects of 10 ppm iNO in ten paediatric patients with acute bilateral lung disease requiring a positive end-expiratory pressure (PEEP) > 6 cmH2O and a fraction of inspired oxygen (FiO2) > 0.5 for > 12 h with 12 control patients [38]. Although there was an immediate, but unsustained improvement in pulmonary vascular resistance and systemic oxygenation defined by the OI, no beneficial effect on mortality was observed although this study was not designed to assess mortality. Dobyns et al. performed a prospective, multicentre, placebo-controlled RCT of 108 children > 1 month of age with severe acute hypoxaemic respiratory failure (i.e. OI > 15) randomised to iNO 10 ppm (N = 53 children) or control (N = 55) [43]. Patients with a congenital heart defect or after cardiac surgery were not included in this study, and nearly half of the patients suffered from underlying diseases (i.e. chronic lung disease or immunodeficiency). The positive effect of iNO on oxygenation was confirmed in just about half of the patients. Mortality was comparable between the two groups, although again this trial was also not designed to assess this. Subgroup analysis revealed a possible beneficial effect of iNO in immunocompromised patients and those with severe hypoxaemia (i.e. OI > 25), although the small sample size in these analyses precludes the robustness of these findings. Ibrahim et al. randomised 32 children aged 2 months–10 years with severe ARDS (PaO2/FiO2 ≤ 200 mmHg, positive inspiratory pressure ≥ 30 cmH2O and a FiO2 ≥ 0.5) to one of the three groups: (1) 24 h of iNO at 5 ppm in the prone position, (2) 24 h of iNO at 5 ppm in the supine position or (3) no iNO in the prone position [73]. In line with the two other studies, these investigators observed a significant improvement in oxygenation, but not in mortality. It may thus be concluded that although iNO improves oxygenation in PARDS, it does not positively affect patient outcomes. This conclusion is strengthened by the outcome of a Cochrane analysis of 604 children and adults with ARDS [3]. Oxygenation was improved, but there was no improvement in mortality, duration of mechanical ventilation, ventilator-free days or length of intensive care unit (ICU) or hospital stay in the iNO cohort [3]. Additionally, there appeared to be an increased occurrence of renal impairment in patients managed with iNO. Therefore, the use of iNO for PARDS should only be considered in patients with documented pulmonary hypertension or severe right ventricular dysfunction [99]. In patients with intractable hypoxaemia, iNO may be considered as rescue therapy from or as a bridge to extracorporeal life support (ECLS).
19.6.1.2 Corticosteroids
ARDS is characterised by an overwhelming inflammatory process [102]. Paediatric data on the effectiveness of steroids is limited to the case series, including the use of methylprednisolone (initial loading dose 5 mg/kg and subsequent maintenance therapy for 2 weeks of 2 mg/kg every 6 h) in a 12-month-old infant with late ARDS, and a case series of six children treated with high-dose steroids [60, 65, 68, 101]. To date, no RCTs have been performed investigating the efficacy of glucocorticoids in PARDS. The role of glucocorticoids as therapy for PARDS remains to be determined. Studies performed in adults with ARDS also fail to provide a clear answer. In fact, two systematic reviews of published adult data reported conflicting results [115, 137].
19.6.1.3 Other Pharmacological Therapies
Inhaled prostaglandin I2, a natural pulmonary vasodilator, may be considered in a similar manner as iNO [35, 71, 113]. Dahlem observed a significant median improvement of 26% in the OI following nebulisation of 30 ng/kg/min epoprostenol, but the effect on patient outcome remains unknown [35]. To date, the effect of inhaled beta-adrenergic receptor agonists as the current adult evidence discourages the use of β2-agonist among ARDS patients, heliox, N-acetylcysteine or other drugs such as of ipratropium bromide, dornase alpha outside the cystic fibrosis population, plasminogen activators, fibrinolytics or other anticoagulants on patient outcome that remains to be established [2, 107, 130, 154].
19.6.2 Non-pharmacological Interventions
19.6.2.1 Prone Positioning
Preliminary data in children with acute lung injury subjected to prone positioning showed improved oxygenation without serious adverse events [136]. To date, only one RCT has been performed in children to investigate the efficacy of prone positioning on patient outcome [32]. In this particular study, N = 102 patients with acute lung injury were randomised to prone position for 20 h each day or supine. Despite the significant improvement in oxygenation, the study was stopped at the planned interim analysis on the basis of futility. Prone positioning did not exert a beneficial effect on ventilator-free days, all-cause mortality, time to recovery from lung injury, the number of organ-failure-free days, cognitive function or overall health. However, the drawback of this trial was that it was not limited to paediatric patients with severe PARDS. A meta-analysis of adult ARDS patients showed that the effect of prone positioning was the greatest in patients with severe disease, i.e. a PaO2/FiO2 < 100 mmHg [132]. This conclusion was supported by the adult PROSEVA trial, showing a significant improvement in mortality in patients with severe ARDS (i.e. PaO2/FiO2 < 150 mmHg [64].
19.6.2.2 Endotracheal Suctioning
Maintaining a patent airway is essential to the safe care of any mechanically ventilated patient, thereby making it one of the most performed interventions despite the lack of scientific evidence including in PARDS [28, 59, 106]. Also, the technique used to perform endotracheal suctioning also requires further study. So far, no RCT evaluating the effect of a closed versus open suctioning on patient outcome in PARDS is available. However, a drop in dynamic compliance and expired Vt indicative of a loss of lung volume may occur during open suctioning, thereby favouring closed suctioning systems [10, 105].
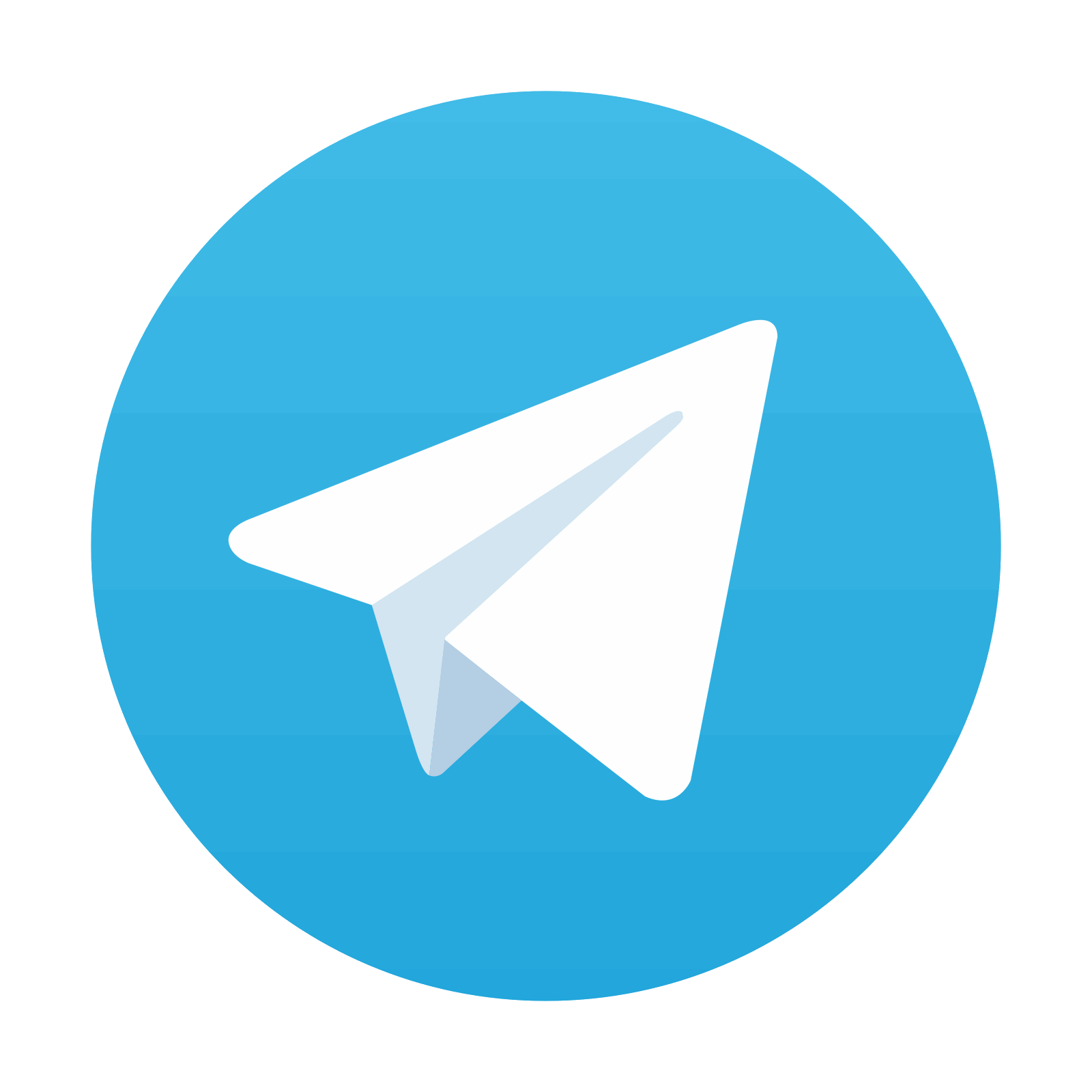
Stay updated, free articles. Join our Telegram channel

Full access? Get Clinical Tree
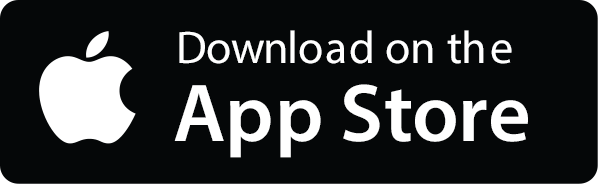
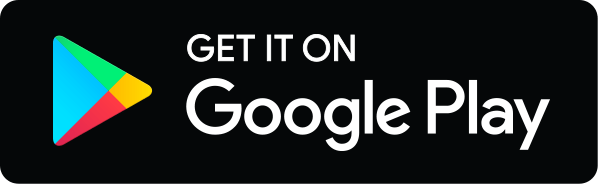