Imaging technique
Clinical role
Technical limitations
X-ray
Lung assessment
Poor contrast diagnostic resolution
Quality of the exam affected by ICU conditions
Radiation exposure
Computed tomography
Lung assessment
Lung recruitment
Overdistension evaluation
Lung perfusion analysis
Risk of critically ill patient’s transport
Radiation exposure
Lung ultrasound
Lung assessment
Lung recruitment
Operator-dependent technique
Physical shape such as obesity, subcutaneous emphysema, large rib cage
Positron emission tomography
Lung assessment
Risk of critically ill patient’s transport
Time-consuming
Short half-life of tracers
Electrical impedance tomography
Lung assessment
Lung recruitment
Lung perfusion analysis
Indirect acquisition
Detection of relative changes in functionally active structure
Magnetic resonance imaging
Lung assessment
Risk of critically ill patient’s transport
Required dedicated assistance, monitoring, and devices
Time-consuming
10.2 Diagnostic Assessment
10.2.1 Chest X-Ray
For almost 20 years, chest X-ray has represented the main tool to evaluate the extension and the distribution of ARDS lung opacities.
However, in the first 24 h following the insult, chest X-ray can be quite normal. During the following 2–3 days, the chest X-ray can show bilateral opacities due to exudation of inflammatory fluid into the interstitium and air spaces independent of its etiology [2, 3]. It has been demonstrated that chest radiography can be insufficient to detect lung alteration in the initial stages of the disease; in fact up to 50% of patients with acute respiratory failure within the first 3 days do not present any lung abnormalities on chest X-ray [4]. Currently, it has been also reported a lower accuracy in detecting pleural effusions, alveolar consolidation, and alveolar-interstitial syndrome compared to lung ultrasound [5]. In fact a recent meta-analysis concluded that a systematic but unselective daily routine chest radiographs might probably be eliminated without increasing adverse outcomes in ICU adult patients [6].
10.2.2 Computed Tomography
Computed tomography (CT) scan has substantially improved our understanding of the inhomogeneous pattern of ARDS. Differently from chest X-ray, it avoids the superimposition of different structures and also allows accurate measurement of the extent of the abnormalities and of their density [7].
The classical morphological CT description includes the recognition of normally aerated lung, poorly inflated areas, characterized by increased density but with still recognizable vessels (ground-glass opacifications) and not-inflated areas with increased density and no recognizable vessels (consolidation) [8]. The increase in lung tissue and the reduction in lung gas volume due to the combination of alveolar flooding (edema), interstitial inflammation, and compression atelectasis are typical of the acute phase of ARDS.
In the early phase, ground-glass opacity has quite a distribution over the entire lung field, whereas consolidation is more prevalent in the dependent ones. In the late phase, over 2 weeks after the first insult, the reticular pattern is the single most frequent pattern and is more represented in the nondependent regions [9]. More than 70% of patients present with CT abnormalities at 6 months follow-up, characterized by fibrosis, air cysts, and bullae mainly located in the ventral, nondependent regions. They are more evident at CT scan than at chest radiography [10].
Furthermore, differences in pathophysiology between ARDS secondary to direct pulmonary insult and ARDS secondary to extrapulmonary disease reflect different CT morphological patterns. The former presents asymmetrical focal areas of consolidation, while extrapulmonary ARDS shows a symmetrical pattern with prevalence of ground-glass areas [11].
In the past decades, the CT quantitative analysis of poorly/normally aerated lung regions has led to the discovery that the ARDS lung is not stiff but “small” (the “baby lung”) and that the residual inflated lung is nearly normal. Moreover, it was showed that ARDS lung is very heavy, weighing 2–3 times that of a normal lung with a content of air decreased to values 20–30% of normal [12].
Then, CT proved that the acute exudative lesions in ARDS have a gravitationally dependent gradient of distribution, with more consolidation in the posterobasal regions, as a result of compressive gravitational forces [13]. In conclusion regional analysis of CT scan has allowed to demonstrate a nonhomogeneous distribution of densities since the gas content progressively decreases from top to bottom because each alveolus is compressed by the weight of the superimposed structures [14]. Accordingly this physical model, in order to inflate the collapsed lung regions and to maintain open these regions, mechanical ventilation should overcome the superimposed pressure generated by the lung mass and pleural pressure.
10.2.3 Lung Ultrasound
Nowadays there is a great interest in the application of lung ultrasound in intensive care unit (ICU). In particular lung ultrasound (LUS) is a reproducible noninvasive bedside technique [15]. It has been shown to be superior to auscultation and chest radiography in the detection of the main ARDS pathology entities (alveolar-interstitial syndrome, pleural effusions, and lung consolidation) when considering CT scan as the reference method for a correct diagnosis [5]. Recently, evidence-based and expert consensus recommendations for LUS with a focus on emergency and ICU settings were provided [16]. To summarize, four patterns corresponding to various degrees of lung aeration can be described [16]:
- 1.
Artifactual horizontal A lines parallel to the pleural line that characterize normal pulmonary aeration
- 2.
Multiple and well-separated hyperechoic vertical lines, arising from pleural line (B lines) corresponding to moderate decrease in lung aeration resulting from interstitial syndrome
- 3.
Coalescent B lines less than 3 mm apart corresponding to more severe decrease in lung aeration resulting from partial filling of alveolar spaces by pulmonary edema or confluent bronchopneumonia [17]
- 4.
Lung consolidation containing white points characterized by dynamic bronchograms corresponding to complete loss of lung aeration with persisting aeration of distal bronchioles
Alveolar-interstitial syndrome (moderate or severe) and consolidation are present in all ultrasound examinations of ARDS patients. In fact the heterogeneous LUS pattern including well-separated or coalescent B lines and the presence of posterior lung consolidations with dynamic bronchograms is typical of ARDS [18, 19] and allows its early distinction from acute cardiogenic pulmonary edema [20].
10.2.4 Positron Emission Tomography
Positron emission tomography (PET) is a functional imaging technique based on the administration of a molecule labeled with a radioactive isotope, which decays with the emission of a positron. Any molecule virtually can be labeled without altering its properties so that PET scan can be useful to investigate any physiological process characterized by the kinetics of a given molecule. Hundreds of tracers have been developed and applied, and depending on which tracer is used, we can study the in vivo imaging of several organic functions. PET with 18-fluoride-2-deoxy-D-glucose (18-FDG) has been used to study cellular metabolic activity, reflecting the activity of inflammatory cells [21, 22]. In fact, 18-FDG is an analogue of glucose that is taken up by cells at the same rate of the glucose, so its accumulation is proportional to the intensity of the glycolytic metabolism of the cells. The uptake of 18-FDG is principally related to the activation of neutrophils [21, 22].
In patients with ARDS, PET with 18-FDG revealed an increased metabolic activity; however, the inflammation is not confined to regions with parenchymal abnormalities at CT scan but also involved normally aerated regions, suggesting that no pulmonary region is spared from inflammatory process [23]. It was demonstrated that in subjects who subsequently developed ARDS, a diffuse 18-FDG uptake was identified 1–3 days before the clinical manifestation of disease [24].
Moreover, recent studies focused on the possible influence of ventilation strategies on metabolic activity. Bellani et al. found that the metabolic activity (i.e., FDG uptake) of normally aerated regions was higher at an airway plateau pressure higher than 26 cmH2O, whereas no association was found between cyclic recruitment/derecruitment and increased metabolic activity [25].
In conclusion, although its role in the daily clinical practice is not feasible, it represents a precious tool to better understand the relationship between lung imaging and lung injury and the link between ventilator-induced lung injury and ventilatory strategies and to measure the effect of anti-inflammatory interventions [26].
10.2.5 Electrical Impedance Tomography
Electrical impedance tomography (EIT) is a noninvasive, radiation-free, bedside lung imaging technique, which tracks changes in regional electrical resistivity of the lung tissue, corresponding to changes in regional gas content in relation to a reference state. Only functionally active lung structures are displayed, whereas structures – either normal or pathological (e.g., stable pneumothoraces or pleural effusions) – that do not change over time are functionally mute and cannot be represented as an image [27].
10.2.6 Magnetic Resonance Imaging
Magnetic resonance imaging (MRI) is a functional technique that can provide information about ventilation-perfusion heterogeneity, pulmonary end-capillary diffusion of oxygen, and lung microstructure. According to the physical properties of the noble gas used as contrast agent, it can provide different information; in fact 3helium remains into the airways and alveolar spaces, and 129xenon follows the diffusion pathway of oxygen. An experimental study showed the redistribution of alveolar gas due to the application of recruitment maneuvers and PEEP using 3 helium in mechanically ventilated rats with atelectasis [28]. At now, MRI data on ICU patients are limited; however, this technique could offer the possibility to match information about regional lung microstructure, function, and ventilation [27].
10.3 Recruitment
Lung recruitment is the amount of lung-collapsed tissue that can be reopened by applying high transpulmonary pressure for an adequate period of time. The decrease of the lung gas volume with the development of non-aerated regions mainly in the more dependent part is typical of the ARDS because of the increase of lung edema and of lung weight.
10.3.1 Computed Tomography
Quantitative CT scan analysis is the reference method for computing lung recruitment. It requires dedicated software and manual delineation of the perimeter of the lungs in each CT image.
It can be computed as the amount of non-inflated tissue that regains inflation [29], or as the increase in gas volume in the poorly and non-aerated tissue [30] at two different levels of pressures (Fig. 10.1). However, these two methods are not interchangeable; in fact the first method underestimated lung recruitment compared to the second one probably because it did not take into account lung recruitment in the poorly aerated lung regions. Due to the different amount of lung edema, the lung recruitability, estimated by lung CT scan, was found in whole population of ARDS patients, to range from 0% up to 70% of the total lung weight [19].
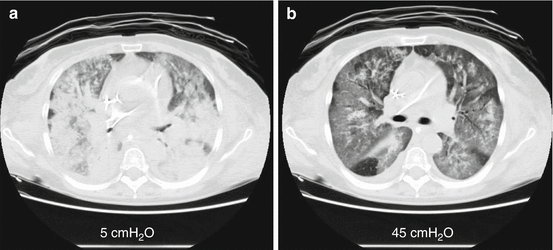
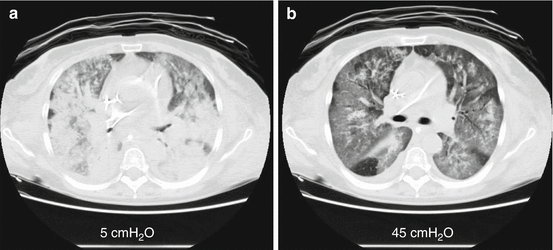
Fig. 10.1
Computed tomography and lung recruitment. The use of computed tomography scanning to identify potential for lung recruitment. Differences in aerated and non-aerated tissue at different pressure levels are presented. Panel (a) shows CT scan performed at end-expiration at 5 cmH2O of PEEP; Panel (b) shows the CT scan of the same lung slice performed at inspiratory plateau pressure of 45 cmH2O
In clinical practice, to quantify lung recruitability may be important for assessing the severity of ARDS, planning recruitment maneuvers, and setting adequate PEEP levels during mechanical ventilation. In fact, lung recruitability affects the response to the applied PEEP levels: the higher is the lung recruitability, the higher will be the end-expiratory alveolar collapse that PEEP can prevent.
Recently Chiumello et al. compared CT scan-based and respiratory mechanics-based methods to assess the lung recruitment, and they found that they measure different phenomena related to the pressure increase: the CT scan measures the amount collapsed tissue which regains inflation, while the respiratory mechanics-based methods measure gas entering in already open pulmonary units which improve their mechanical properties at higher PEEP that is the overall improvement of inflation [31].
Obviously, quantitative analysis of the whole lung is labor-intensive and time-consuming. To increase the feasibility of the use of CT, Chiumello and colleagues demonstrated that visual anatomical analysis of lung CT had a good accuracy in detecting patients with high or low recruitability, although this method is unable to estimate the hyperinflation [32].
10.3.2 Lung Ultrasound
It was demonstrated that an improvement in lung aeration might be detected by corresponding changes in LUS patterns. If the whole lung is examined, LUS using scores based on number of B lines can monitor extension of pulmonary edema, the amount of extravascular lung water, and the corresponding decrease in lung aeration.
Stefanidis and colleagues [33] estimated the size of non-aerated tissue in dependent lung regions at different PEEP levels, considering only not-inflated regions. Moreover, Bohaemad et al. realized a reaeration score to quantify the PEEP-induced lung recruitment that was demonstrated reliable as the pressure-volume curve method [34].
The LUS reaeration score should be performed on 12 regions of interest, including upper and lower posterior regions. In each region examined, changes in ultrasound pattern following the application of PEEP are detected, and numbers are attributed as follows:
One point from multiple B lines to normal, from coalescent B lines to multiple B lines, and from consolidation to coalescent B lines
Three points from coalescent B lines to normal or from consolidation to multiple B lines
Five points from consolidation to normal
Interestingly in this study, the essential of recruitment results from the reaeration of poorly aerated lung regions. It means that coalescent B lines are transformed into separated B or A lines, and separated B lines are transformed into A lines. The reaeration of non-aerated lung regions remains marginal; in fact consolidations transformed into coalescent or separated B lines, or A lines, are a rarely observed event, occurring mostly in the lower parts of anterior, lateral, and posterior lung regions.
A high correlation was found between LUS reaeration score and CT reaeration. However, further studies using CT of the whole lung as gold standard are required to evaluate ability of LUS to reliably estimate PEEP-induced lung recruitment [35].
10.3.3 Electrical Impedance Tomography
In ARDS patients, the amount of potentially recruitable lung volume and the difference between the open, fully recruited, and the not recruited lung volume at 40 cmH2O was computed as 26% ± 11% (range 11–47%) using EIT [36]. Thanks to its dynamic analysis, EIT has also allowed to identify alveolar opening of collapsed regions, the overdistension of opened areas, the collapse of previously opened regions, and the recovery of previously overdistended alveoli, during stepwise changes of mean airway pressure [37]. In particular, it was used to further study the regional intratidal gas distribution during the lung recruitment at different PEEP levels. For these purposes, EIT image can be divided in four anteroposterior quadrants (dorsal and ventral regions for the left and right lungs), and a regional analysis can be performed for each quadrant.
The study of the dynamic behaviors of the lung during slow recruitment maneuvers, in particular the delay time needed for the regional impedance in each quadrant to reach a certain threshold value of the maximal local impedance change, has allowed to construct maps of regional delays in ventilation that correlate with the amount of recruited lung and lung inhomogeneity and showed that distribution of gas started in the nondependent lung regions and ended in the dependent lung regions [38, 39].
The advantage of EIT is that the image might change following recruitment maneuvers, physiotherapy, or prone positioning, indicating a response to such treatments. In this context, EIT can open new scenarios to guide the PEEP titration strategy aimed at a decrease of the regional ventilation inhomogeneity, minimizing tidal collapse and hyperinflation.
10.4 Overdistension
10.4.1 Computed Tomography
The application of high levels of PEEP in patients with low lung recruitability can lead to overdistention of regions that are already aerated without opening the collapsed tissue (i.e., strain) [19]. CT scan is a unique tool to differentiate between recruitment and hyperinflation. Overdistension is defined by quantitative lung CT analysis as lung regions with a density threshold below – 900 Hounsfield units [18]. However, the quantitative computation of lung overdistension can be affected by the size of the voxel or the CT section thickness and by the type of reconstruction filter. In fact, a thick CT section (10 mm) underestimates the overinflated lung volume by 3–5% compared to thin CT sections (1.5 mm) [18]. The evaluation of hyperinflation can give information about the safety of our mechanical ventilation. In fact Terragni et al. have found that one-third of ARDS patients with large not aerated lung regions presented a substantial tidal hyperinflation, even when airway plateau pressure was between 28 and 30 cmH2O [40].
Recently Cressoni et al. have quantified the lung inhomogeneities by CT scan. They found that the extent of lung inhomogeneities increased with the severity of ARDS, correlated with the physiologic dead space, and it was associated with overall mortality. Interestingly they also demonstrated that in most of the patients, lung inhomogeneities decrease by increasing PEEP or airway pressure, suggesting a more homogeneous lung at higher pressure. However, in a small part of patients, lung inhomogeneity extent increased, instead of decreased while increasing PEEP or plateau pressure. This was due to the increase of poorly aerated tissue and increasing pressures, while in most patients where lung inhomogeneities decreased increasing pressure, the poorly aerated tissue also decreased [41].
Once again CT scan has provided physiological information to better understand the complex interaction between mechanical ventilation and ventilator-induced lung injury.
10.5 Lung Perfusion
As known, arterial oxygenation results from the coupling between alveolar ventilation and perfusion. In fact in ARDS patients, hypoxemia is closely related to the consolidation or collapse of lung regions, which increases the intrapulmonary shunt. Accordingly, an important objective in the setting of mechanical ventilation is to optimally match ventilation with lung perfusion in order to improve gas exchange [27, 42]. Different imaging techniques such as computed tomography and electrical impedance tomography have been used to investigate the distribution of aeration and/or ventilation in ARDS. The understanding of the regional lung perfusion can be useful for the daily clinical practice for different reasons. Firstly, the response in oxygenation to different ventilatory strategies can be due to redistribution of lung perfusion and not to lung recruitment or derecruitment. Consequently, the level of PEEP should be titrated on respiratory mechanics changes and not on oxygenation. Then intravenous antibiotics cannot reach the affected regions because of the unpredictable distribution of blood flow.
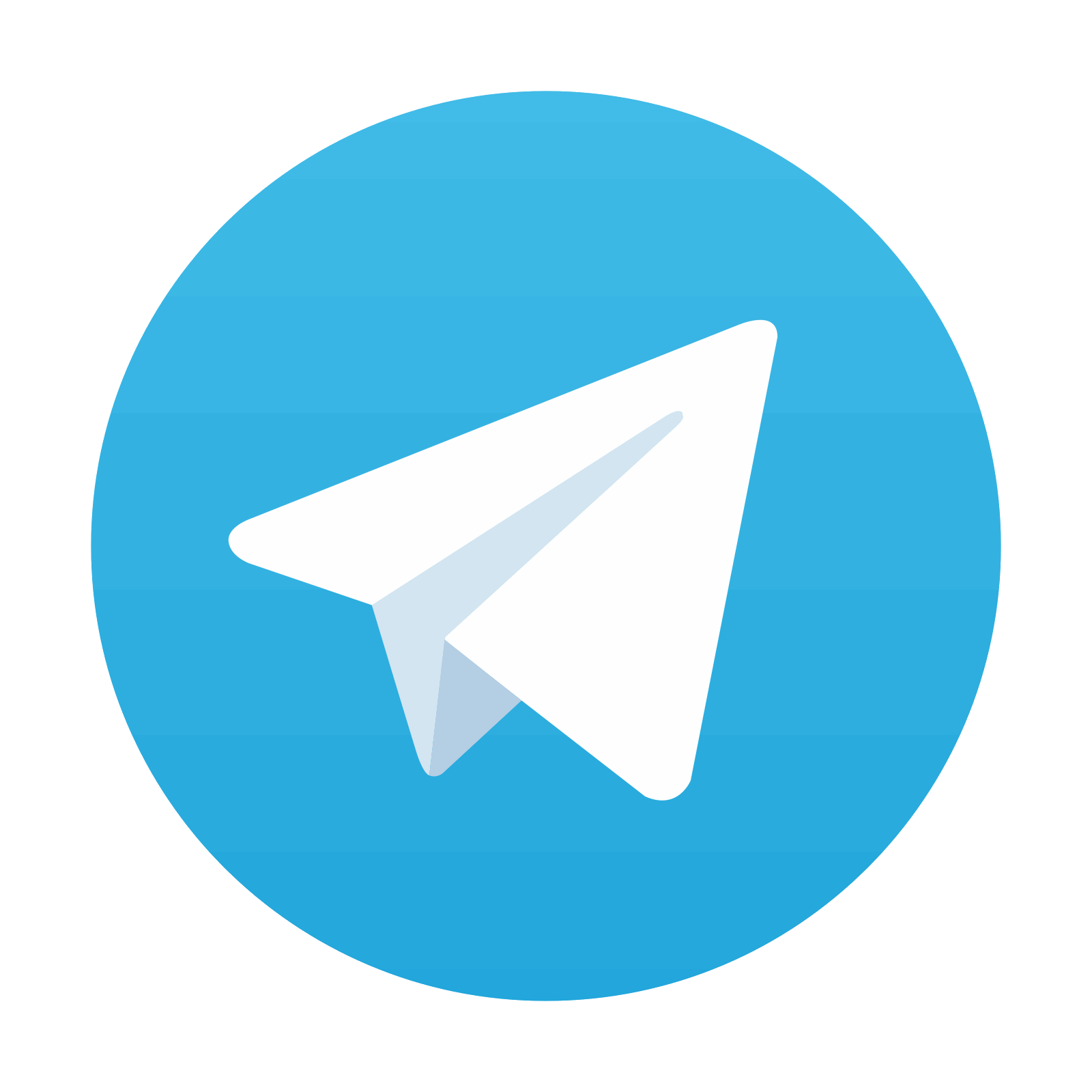
Stay updated, free articles. Join our Telegram channel

Full access? Get Clinical Tree
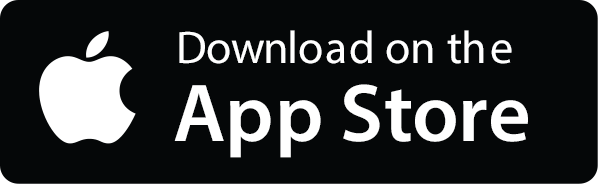
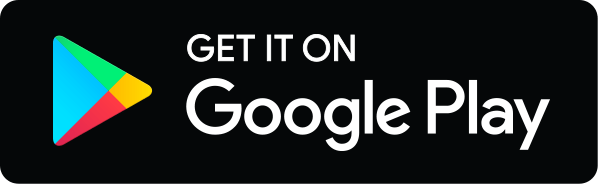