Central venous pressure
Urine output
<0.5 mL/kg/h
Urine output
≥0.5 mL/kg/h
>8
Furosemideb
Furosemidec
4–8
Fluid bolusb
Furosemidec
<4
Fluid bolusb
No interventionc
A retrospective comparison of the performance of FACTT Lite with FACTT Conservative and FACTT Liberal was recently reported. Fluid management with FACTT Lite resulted in a significantly greater average cumulative fluid balance (+2.05 L) over 7 days than FACTT Conservative but a significantly lower average cumulative fluid balance compared to FACTT Liberal (+5.07 L mL) over the same time period. In subjects without baseline shock, in whom the fluid protocol was applied throughout the duration of the study, management with FACTT Lite resulted in an equivalent cumulative fluid balance to FACTT Conservative and had similar clinical outcomes of ventilator-free days, ICU-free days, and mortality as FACTT Conservative and significantly greater ventilator-free days and ICU-free days than FACTT Liberal. Interestingly, development of new-onset shock during the study was lower in the FACTT Lite group than in the FACTT Conservative group. This might be explained by a less aggressive diuresis in the first 2 days in the FACTT Lite group compared with the FACTT Conservative group.
8.6.2 Enhancing Edema Clearance
8.6.2.1 Modulation of Oncotic Pressure
Endogenous albumin is an important regulator of endothelial integrity [70, 71]. From a hemodynamic point of view, hypoalbuminemia results in a decrease in plasma oncotic pressure; therefore, pulmonary edema forms at a lower hydrostatic pressure because the oncotic pressure gradient between plasma and the interstitium decreases [72]. Administration of albumin to raise oncotic pressure and promote the water movement from the interstitial to the intravascular space has been suggested as a method to reduce pulmonary edema. This approach can be combined with the use of furosemide to facilitate the removal of the excess fluid from the plasma. The efficacy of this approach was initially evaluated by Martin et al. in a trial of 37 hypoproteinemic patients with acute lung injury randomized to receive protocolized albumin replacement and continuous infusion of furosemide versus double placebo [73]. The patients treated with albumin and furosemide showed improvement of oxygenation with better hemodynamics and fluid balance compared with controls. Based on the results of this study, it was estimated that the albumin/furosemide protocol accelerated the edema clearance, by 3–4 days compared with its natural evolution [74]. The beneficial effect of albumin administration was subsequently evaluated in a small trial which compared the efficacy of furosemide and albumin compared to furosemide alone [75]. This trial suggested that albumin is a critical component of this regimen, for both maintenance of hemodynamic stability and improved oxygenation. While these studies suggest promise, it is important to stress, however, that both studies excluded patients with hemodynamic instability and/or receiving vasopressor support suggesting that the full-blown phase of ARDS was already overcome in the enrolled patients. Larger trials are needed to confirm the findings and define the optimal timing of colloid/diuretic administration.
8.6.2.2 Pharmacologic Therapies
The resolution of alveolar edema does not occur by normalization of vascular pressures alone but is driven by active transport of sodium and chloride ions from the alveolar luminal space across the epithelial cells, thereby creating an osmotic gradient for the reabsorption of water. The use of pharmacologic agents to promote sodium (and water) transport out of the alveoli is an appealing and complementary way to enhance the edema clearance. Even with severe epithelial injury, alveolar clearance may be pharmacologically stimulatable [76]. Several experimental studies have shown that the exogenous administration of cAMP agonists, in particular β2agonists, accelerates the resolution of edema [77]. The action of β2agonists principally occurs through an increase in the quantity and activity of Na/K pumps in the basal membrane and sodium channels in the pneumocyte apical membrane. The net effect of these actions is to increase the sodium gradient between the alveoli and the interstitium and thereby increase the absorption of water.
A randomized trial in 40 ARDS patients by Perkins et al. suggested that the infusion of salbutamol compared with placebo for 7 days was able to significantly diminish the quantity of pulmonary water measured by transpulmonary thermodilution without affecting oxygenation and duration of mechanical ventilation [78]. Unfortunately, the suggested benefit was not demonstrated in successive trials. In fact, a multicenter trial by the same investigators that randomly assigned ARDS patients to intravenous salbutamol or placebo was terminated early because of excess mortality in the treatment group [79]. In the related trial which compared aerosolized albuterol or saline treatment in acute lung injury, there was also no benefit in either ventilator-free days or mortality [80]. A number of possible explanations have been proposed for failure of β2agonist therapy to improve outcomes in these clinical trials including inadequate aerosol drug delivery, downregulation of beta-2 receptors, and inability of injured alveolar epithelium to respond to a β2agonist. Finally, it is possible that with lung-protective ventilation and a fluid-conservative hemodynamic strategy, there was little opportunity to further enhance alveolar fluid clearance with the β2agonist. While some questions remain, such as whether or not there may be a benefit with a different cAMP agonist or benefit in specific subpopulations, the available data do not support the use of β2agonist to facilitate edema clearance.
8.7 General Paradigm for Resuscitation in ARDS
In addition to the amount of fluid administered, it is increasingly being recognized that timing of fluid administration during the course of critical illness is crucial: both immediate commencements of resuscitation with target end points in the early phase of critical illness followed by fluid restriction and mobilization when shock resolves are of utmost importance in achieving best outcomes. This process has recently been more formally divided into four phases that are distinguishable based on the time course of critical illness denoted by the acronym “ROSE”: rescue, optimization, stabilization, and evacuation (Table 8.2) [81]. In each of these four stages of treatment modalities, goals and monitoring tools can substantially differ. Adaptation of this paradigm to the hemodynamic management of patients with ARDS may be beneficial for patient management and is described in the following section and summarized in Table 8.2.
Table 8.2
Paradigm for fluid resuscitation/hemodynamic management in patients with ARDS
Stage | Patient characteristics | Hemodynamic goals | Strategy | Targets and monitoring tools |
---|---|---|---|---|
Rescue | Severe hypotension Evidence of tissue hypoperfusion | Rapid correction of shock Maintain lower autoregulatory limits for organ perfusion | Rapid fluid bolusing to increase cardiac preload and CO | Clinical signs/symptoms Macrohemodynamics (MAP, CO) Lactate Dynamic parameters (PPV, IVC variation, fluid bolus, PLR) |
Optimization | Evidence of tissue hypoperfusion | Improve cardiac output and oxygen delivery Maintain tissue perfusion | Titrate fluids Continue fluid boluses in responders and avoid in nonresponders Initiate vasoactive agents early in fluid nonresponders Inotropic support if poor cardiac performance coupled with signs of organ hypoperfusion | Organ perfusion (MAP, CO, ScvO2) Fluid responsiveness EVLW and PVPI as safety parameters |
Stabilization | No evidence of tissue hypoperfusion Stable or decreasing doses of vasopressors | Maintain even to negative fluid balance | Restrict fluids | Daily and cumulative fluid balance, body weight |
Evacuation | No evidence of tissue hypoperfusion No requirement for vasopressors | Mobilize accumulated fluid while avoiding organ hypoperfusion | Administer diuretics +/− albumin Extracorporeal removal if poor response to diuretics | Negative daily and cumulative fluid balance, body weight Ultrasound (E/Ea ratio) |
The rescue phase encompasses the period of initial stabilization of the patient with severe systemic hypotension and evidence of tissue hypoperfusion. In this phase, the immediate goal is to correct the shock, and intravenous fluids are typically first line therapy. Fluid administration is expected to increase cardiac preload and, in the case of preload dependence, to increase cardiac output and eventually improve tissue oxygenation. Evidence is lacking to support the use of specific resuscitation protocols for patients with ARDS during this period. The volume of fluid required during this phase must be individualized and will necessarily vary between patients. However, patients with coincident conditions such as sepsis may benefit from a protocolized approach developed for that condition. Since the need for intervention is immediate, only those readily available tools and targets should be considered. Multiple fluid boluses may need to be administered to achieve a predefined blood pressure target, often with limited hemodynamic monitoring such as routine bedside vital signs. During the resuscitative phase, fluids must be administered in a timely and targeted manner with appropriate limits to fluid administration.
Maintaining the mean arterial pressure above the lower autoregulatory threshold for perfusion of the most vulnerable organs (heart, brain, kidneys) is essential. Invasive blood pressure monitoring should be initiated to allow continuous blood pressure display. Consideration of the patients’ individual premorbid conditions (e.g., hypertension, CHF) may be more beneficial in establishing resuscitation goals rather than what is recommended as a universal target in the guidelines. Maintaining adequate mean arterial pressure in mechanically ventilated patients with permissive hypercapnia is critical given that impaired cerebral autoregulation can predispose patients with decreased cerebrovascular reserve to cerebral ischemia [82]. Besides, standard hemodynamic monitors, ultrasonography, and echocardiography may offer valuable advanced information on heart function, including preload, contractility, and ventricular performance.
In the optimization phase, the therapeutic goal is to maintain tissue perfusion. This phase should be monitored using clinical judgment as well as physiologic and biochemical parameters individualized to the patient. Conventional indicators of the adequacy of resuscitation, such as the resolution of oliguria, a decrease in lactate levels, and improving ScvO2, can be helpful but may not occur in every patient. However, it is important to acknowledge that none of these indices have a high enough sensitivity on their own to be used as a target in every patient; therefore, a “multimodal” approach to optimization may be necessary. Monitoring of EVLW and PVPI may be valuable to prevent fluid overload. Vasoactive agents should be initiated early in fluid nonresponders in order to minimize the pathological pooling of blood and help mobilizing the unstressed volume. Providing inotropic support should be reserved for those patients who after optimizing both pre- and afterload still show unsatisfactory cardiac performance coupled with signs of organ hypoperfusion.
Fluid administration is often a necessary component of optimization, but unmonitored volume resuscitation in patients with persistent hypoperfusion increases the likelihood of fluid overload. The absence of fluid responsiveness or evidence of fluid overload should be used to determine when to stop giving fluid challenges in patients who fail to achieve their resuscitation goals. The importance of this approach in patients with ARDS is demonstrated by a recently published retrospective analysis of the FACTT trial which examined the effect of fluid bolus administration in the patients randomized to the pulmonary artery catheter arm of the study [83]. Importantly, less than a quarter of the patients were fluid responders (increase in CI >15%). Furthermore, fluid boluses were unlikely to result in an increase in urine output, and only small changes in heart rate or mean arterial pressure after a fluid bolus were noted. Therefore, routine administration of fluids to patients with shock or oliguria who were previously resuscitated may not improve organ function.
In the stabilization phase, there is adequate tissue perfusion, and although patients may still require vasopressor support, the doses of these medications are stable or decreasing. In the absence of evidence suggesting hypoperfusion, fluid administration should be restricted. Fluid challenges should not be routinely given, even in those who are fluid responsive. Maintenance fluids are usually not required and should be discontinued when possible as critically ill patients frequently receive sufficient fluids with medications and nutrition to account for insensible losses.
In the evacuation phase, interventions are targeted at fluid removal. There is no consensus about the optimal timing of fluid removal, and there are limited data to guide a specific recommendation. In the FACTT and FACTT Lite trials, patients were required to have been off vasopressor therapy for at least 12 h before diuretics were administered. A reasonable approach is to begin with restricting fluids when patients achieve hemodynamic stability and/or normal tissue perfusion and then to consider initiating diuresis in patients with clinical evidence of fluid overload that are unable to achieve a negative fluid balance spontaneously. Negative fluid balance may be achieved by the combined administration of furosemide alone or together with albumin. However, in some patients the response to diuretics remains poor. In these cases an active de-resuscitation by extracorporeal means may be considered. There are concerns about the risk of organ hypoperfusion associated with active fluid removal, and the data providing guidance for this phase of management are still limited. Consequently, fluid removal should be performed cautiously with clear limits and appropriate safety monitoring to avoid inducing hypovolemia. The use of hemodynamic indices such as the E/Ea ratio, EVLWI, and PVPI may aid in facilitating edema clearance without compromising end-organ function.
Conclusion
Fluid overload in patients with ARDS is a preventable complication that is associated with increased morbidity and mortality. Two aspects of fluid management appear to be helpful in preventing fluid overload in patients with ARDS. First, during resuscitation, excessive fluid administration should be avoided. Second, removal of excess fluid should be promoted in patients whose shock has been resolved. A stage-targeted approach to hemodynamic management for patients with ARDS which combines clinical judgment along with sophisticated monitoring tools may be beneficial to guide management. Rational fluid management strategies should be implemented during each phase of resuscitation to help mitigate the effects of fluid overload and, thereby, improve outcomes. Initial fluid resuscitation should be guided by an assessment of fluid responsiveness until stabilization occurs, while later management requires a conservative fluid approach or even active fluid removal. Whether specific protocol-directed resuscitation for ARDS-associated shock improves outcome remains to be determined, while in established ARDS, a fluid-conservative approach is the minimum requirement. Further research on how to best personalize fluid therapy for the critically ill patients with ARDS is needed.
References
1.
2.
3.
Roch A, Guervilly C, Papazian L (2011) Fluid management in acute lung injury and ards. Ann Intensive Care 1:16CrossRefPubMedPubMedCentral
4.
5.
Matthay MA, Ware LB, Zimmerman GA (2012) The acute respiratory distress syndrome. J Clin Invest 122:2731–2740CrossRefPubMedPubMedCentral
6.
Matthay MA, Zimmerman GA (2005) Acute lung injury and the acute respiratory distress syndrome: four decades of inquiry into pathogenesis and rational management. Am J Respir Cell Mol Biol 33:319–327CrossRefPubMedPubMedCentral
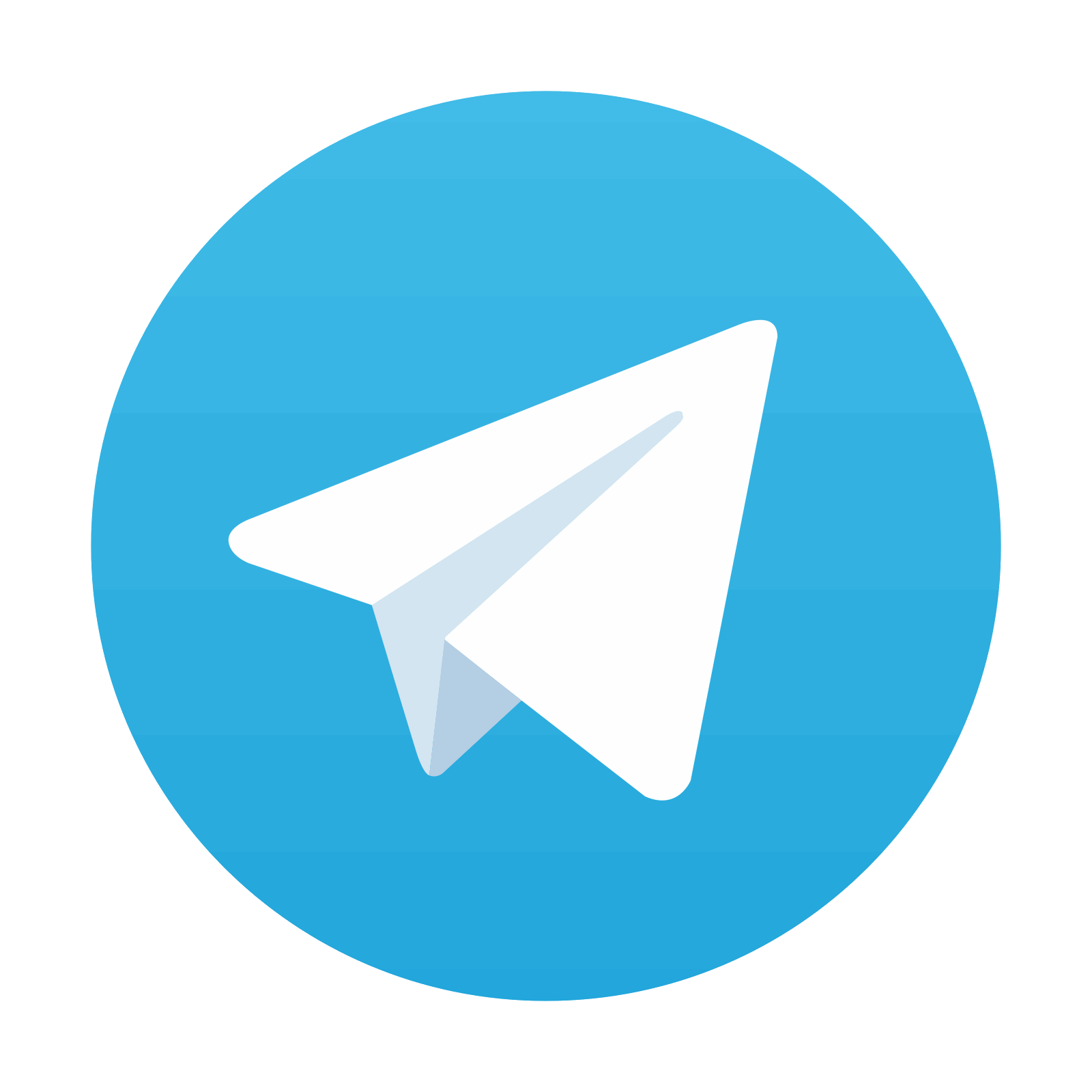
Stay updated, free articles. Join our Telegram channel

Full access? Get Clinical Tree
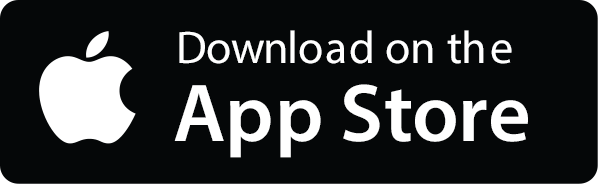
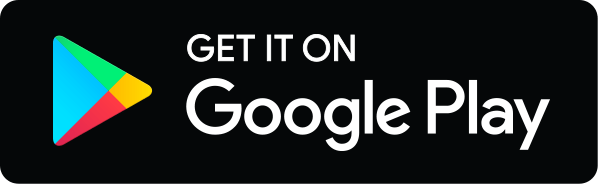