Loren D. Sacks1, Gregory B. Hammer2, and Stephen A. Stayer3, 4 1 Attending Physician, Cardiovascular Intensive Care Unit, Lucile Packard Children’s Hospital, Clinical Assistant Professor, Department of Pediatrics, Stanford University School of Medicine, Stanford, CA, USA 2 Cardiovascular Intensive Care Unit and Pediatric Cardiac Anesthesia, Lucill Packard Children’s Hospital, Professor of Anesthesiology, Perioperative and Pain Medicine, Professor of Pediatrics ‐Critical Care, Stanford University School of Medicine, Stanford, CA, USA 3 Department of Anesthesiology, Perioperative and Pain Medicine, Texas Children’s Hospital and Baylor College of Medicine, Houston, TX, USA 4 Department of Pediatrics and Anesthesiology, Baylor College of Medicine, Arthur S. Keats Division of Pediatric Cardiovascular Anesthesiology, Texas Children’s Hospital, Houston, TX, USA Airway and ventilatory management of infants and children with congenital heart disease (CHD) during diagnostic and therapeutic procedures presents unique challenges to the anesthesiologist. This is a consequence of the interplay of a range of congenital airway abnormalities, cardiopulmonary interactions, and adverse effects of surgery and cardiopulmonary bypass on the respiratory system. Few other clinical situations will tax the skills of an anesthesiologist as the management of a child with CHD and a difficult airway. Children with cardiovascular disease may be intolerant of the myocardial depressant effects of many anesthetics, limiting the options available in managing their airway and circulation during induction of anesthesia. Children with cyanotic congenital heart disease experience rapid oxygen desaturation during periods of apnea associated with tracheal intubation. Moreover, in patients with single‐ventricle disease, the very use of positive‐pressure ventilation may limit pulmonary blood flow, leading to rapid decompensation. Developing a plan that allows safe airway and ventilatory management without hemodynamic compromise requires preparation, skill, and familiarity with a range of techniques of tracheal intubation and mechanical ventilation. The narrowest portion of a child’s larynx is at the level of the cricoid cartilage, as opposed to adult patients whose limiting airway diameter is at the level of the vocal cords (rima glottidis). Uncuffed ETTs have traditionally been used in young children because a seal may be created between the tracheal mucosa and the tube at the level of the cricoid cartilage so that a cuff may not be needed to create a seal [1]. A disadvantage of uncuffed ETTs is that the placement of an ETT with a gas leak at low inflating pressures (e.g., <15 cm H2O) results in excessive leak around the endotracheal tube when lung compliance is poor. This is particularly important for thoracic or cardiovascular surgery because lung compliance may be reduced as a result of surgical traction, pulmonary edema, or atelectasis related to cardiopulmonary bypass (CPB). This results in a greater percentage of gas leak around the ETT. Gas leakage representing more than 50% of tidal volume has been demonstrated in the setting of decreasing lung compliance [2]. Associated decreases in minute ventilation may lead to hypoxemia due to atelectasis and intrapulmonary shunt as well as dangerous elevations in PaCO2 related to decreased alveolar ventilation. Table 23.1 presents a scheme for selection of ETT size in infants and children. Table 23.1 Endotracheal tube sizes used in pediatric patients In addition to the risk of inadequate alveolar ventilation, there are other hazards associated with placement of a loose fitting ETT. Lung function measurements are commonly used to guide mechanical ventilation in the postoperative period. A variable leak around the ETT results in inaccurate measurements of exhaled tidal volumes, lung compliance, and airway resistance. A significant gas leak around the ETT also results in environmental pollution from either inhaled anesthetic agents or nitric oxide [3]. Lastly, an inadequate seal around the ETT may increase the risk of pulmonary aspiration should gastric contents be regurgitated during positive pressure ventilation. Traditional airway management has included the use of uncuffed ETTs in children under the age of 8 years. However, there is limited scientific evidence to support this practice. Since the mid‐1990s, advancements in the materials used in the ETT cuff, the shape of the cuff itself, and the relative outer diameter of the inflated cuff have lowered the risk for airway injury with the use of cuffed ETT’s [4–6]. Cuffed endotracheal tubes are now routinely placed in infants and children, and the incidence of clinically significant airway complications is very low [7]. The use of cuffed ETTs during anesthesia reduces the need for repeated laryngoscopy and tracheal intubation, allows use of lower fresh gas flows, and limits environmental contamination with anesthetic gases [2]. There is no difference in the incidence of airway complications in pediatric patients intubated with cuffed vs. uncuffed endotracheal tubes during anesthesia or in pediatric intensive care units [7, 8]. However, the need to exchange endotracheal tubes is significantly greater when using uncuffed ETTs. Decreased lung compliance from the effects of cardiopulmonary bypass may increase the leak around the endotracheal tube in children after heart surgery, and a cuffed ETT may be partially deflated to compensate for such changes. In a retrospective review of 809 children <2 years old undergoing cardiac surgery over a 4‐year period, the incidence of subglottic stenosis (17/809 or 1.08%) was not affected by the use of uncuffed vs. cuffed ETTs. The most important risk factors for subglottic stenosis were younger age and prolonged (>96 hours) postoperative intubation [9]. There is some evidence suggesting that children with CHD require larger endotracheal ETTs regardless of the duration of surgery [10]. Given this information, the pediatric patient requiring lengthy surgical intervention for congenital heart disease and/or a significant period of post‐operative mechanical ventilation will generally benefit from the use of a cuffed endotracheal tube. A disadvantage of using cuffed ETTs is that their outer diameter is approximately 0.3–0.5 mm larger than uncuffed ETTs with the same inner diameter. As a result, a tube with an inner diameter one size (i.e., 0.5 mm ID) smaller is recommended when a cuffed ETT is placed. The reduction in ETT internal diameter results in greater resistance to gas flow and an increased risk of occlusion of the ETT with blood and tracheal secretions. While a reduction of the tracheal tube diameter by only 0.5 mm might not be expected to effect a clinical change, gas flow resistance increases exponentially at smaller tube diameters. While a reduction in tracheal tube size from 8.0 mm ID to 7.5 mm ID increases airway resistance by 29%, a change from a size 4.0 mm ID to a 3.5 mm ID tube results in an increase of 71%, and a change from a 3.5 mm ID tube to a 3.0 mm ID tube increases resistance by 85%. The increase in resistance is even more profound if turbulent airflow occurs in smaller tracheal tubes. In addition, use of ETTs with a smaller internal diameter may result in the need to use smaller suction catheters, limiting the ability to effectively clear blood and secretions from the airways. The Microcuff® endotracheal tube (Kimberly Clark; Roswell, GA) has been developed for pediatric patients. This ETT includes a true high‐volume / low‐pressure cuff, eliminates the Murphy eye in order to optimize cuff position at the tip of the endotracheal tube, and includes an ultrathin cuff that provides an outer diameter‐to‐inner diameter ratio similar to uncuffed endotracheal tubes. The Microcuff® ETT may decrease the incidence of micro‐aspiration around the ETT cuff. While conventional PVC cuffs create folds and channels when inflated that allow fluid to leak past the cuff, the cuff on the Microcuff® ETT is designed to minimize those channel openings to create a more effective tracheal seal. The cuff length and shape of Microcuff® ETTs are also designed for better contact with tracheal contours to increase protection against fluid leakage. Of note, the lack of a Murphy eye eliminates a channel for gas exchange in the case of an ETT placed too distally in the trachea or in the right mainstem bronchus. Two studies including 575 patients age 0–5 years have found a low incidence of post‐extubation stridor, and a tracheal tube exchange rate of <3% when cuffed ETTs are used in children [11, 12]. A combined analysis of 3 controlled randomized trials of cuffed vs. uncuffed ETTs in 2804 children less than 8 years of age concluded that there was no difference the incidence of post‐extubation stridor requiring treatment with racemic epinephrine or corticosteroids, or of the need for reintubation or need for ICU care [13]. In patients who underwent surgery for congenital heart disease and were managed with Microcuff® ETTs, a retrospective review of 208 patients weighing less than 5 kg found no cases of tracheal stenosis. However, 20.9% of patients developed stridor requiring treatment with racemic epinephrine and 2 patients had severe stridor requiring reintubation [14]. While orotracheal intubation is performed more commonly than nasotracheal intubation for most surgery in children, many centers routinely place nasal ETTs in children undergoing cardiac surgery. Intraoperative transesophageal echocardiography (TEE) may cause dislodgement of an oral ETT, although a low incidence of airway complications during pediatric TEE among children who were orally intubated has been reported [15]. Of the 1650 patients studied, 3 (0.2%) developed a right mainstem advancement of the ETT and 8 (0.5%) were inadvertently extubated. Nasal ETTs are more readily secured to the face, and movement of the ETT is less likely during manipulation of the TEE probe. While nasal intubation may minimize risk of pressure injury from the ETT to the lips, there is a greater risk of damage to nasal alae and, in older children, of sinusitis from long‐term nasotracheal intubation [16–18]. These risks may increase if nasotracheal intubation is utilized infrequently and care providers in the post‐operative phase (e.g., respiratory therapists, bedside nurses, etc.) are unaware of the need to monitor for such complications. Therefore, for patients whose operative course predicts a lengthy ICU stay, institutional familiarity with nasotracheal intubation should be considered in advance. The risk of bleeding from nasal mucosal or adenoidal trauma is especially problematic in the fully anticoagulated patient. This risk is minimized by the routine use of topical vasoconstrictor drugs (e.g., oxymetazoline), adequate lubrication, and warming of the ETT prior to placement. Excessive pressure should not be used during advancement of the tube through the nose and nasopharynx. Prior to advancing the ETT into the nasopharynx, a soft, lubricated, tapered suction catheter can be affixed to the tip of the ETT and advanced through the nasopharynx; it can be gently pulled through the mouth using a laryngoscope and Magill forceps, guiding the ETT into the oropharynx. The catheter acts as a guide permitting easier passage of the nasal ETT through the nasal passages and nasopharynx. The catheter is then removed from the ETT, allowing placement of the ETT into the trachea using the laryngoscope and Magill forceps [19]. Because ETTs can be placed more easily and rapidly via the oral route, oral intubation is preferred for rapid sequence intubation or during emergencies. Once adequate ventilation and oxygenation have been provided and the stomach is suctioned, the ETT may be exchanged for a nasal tube under direct visualization. In general, the nasal passages of children will accommodate the same size ETT as would be used for oral intubation. An analysis of 26,754 cardiac surgery cases from the multicentered Congenital Cardiac Anesthesia Society database compared the outcome of patients who had oral vs. nasal endotracheal intubation [20]. Nasal intubation was performed in 41% of neonates, 38% of infants, 15% of school aged children, and 2% of adolescents; about 80% of neonates and infants had cuffed ETT. There were fewer accidental extubations in neonates who were nasally intubated (0% vs. 0.37%). However, after 12 months of age nasal intubation was associated with an increased risk of infection (mediastinitis, wound infection, pneumonia, septicemia). The duration of mechanical ventilation and hospital stay were not different between groups. The incidence of noncardiac congenital airway anomalies is believed to be greater among children with congenital heart disease than in the general population. Certain genetic disorders are clearly associated with both airway anomalies and congenital heart disease, including CHARGE and velocardiofacial (DiGeorge) syndrome (22q11 deletion). Children with these anomalies should have a complete airway examination, including endoscopic subglottic examination, by an otolaryngologist prior to cardiac surgery. In older, cooperative patients, the airway should be examined as with adult patients, including mouth opening, dentition, mandibular size, hyomental distance, and neck mobility. Studies in adults have shown that examination of the airway can help predict difficulty of intubation and mask ventilation [21]. No such studies have been performed in infants and children, and it is not known whether assessing mouth opening using a tongue depressor in a nonverbal child is predictive of difficulty with intubation. Assessment of an infant’s airway should include an assessment of neck mobility and the appearance of the mandibular size when viewed in profile. Children with micro‐ or retrognathia are more likely to manifest difficult mask ventilation and/or difficult intubation. The incidence of difficult intubation is higher among children with CHD. A study of 11,219 children from the population at large undergoing general anesthesia found 1.35% of patients had difficult intubation, defined as a Cormack‐Lehane view of Grade 3 or higher [22]. The incidence for cardiac surgical patients was 3.6%, a higher incidence than any other group surgical group, including craniofacial surgery. Neonates and infants had a higher incidence of difficult intubation, 2.5% for the general population and 6.9%, for the cardiac surgical group. These same authors reported a detailed analysis of 1,177 pediatric cardiac surgery cases [23]. Interestingly, Trisomy 21 was not associated with difficult intubation; however, 9 of 29 patients (31%) with other genetic/dysmorphic syndromes were found to have difficult intubation (Table 23.2) [23]. They again found a higher incidence associated with younger age; difficult intubation was found in 5.6% of patients less than 12 months vs. 1.7% for older children. A separate retrospective database review of 4,797 patients with CHD from a single center undergoing 8,657 anesthetics for cardiac and non‐cardiac procedures found the incidence of difficult intubation to be about the same as that reported for the general pediatric population ‐ 53 (1.1%) of the patients had a Cormack‐Lehane grade 3 or higher [24]. In this report, 16.7% of patients had a genetic or dysmorphic syndrome, including Trisomy 21 (6%), velocardiofacial syndrome (3.6%), and CHARGE association (3%). Altogether 2.4% of patients required 3 or more intubation attempts. Video laryngoscopy was utilized in 7.7% of patients and fiberoptic bronchoscopy in only 4 patients (0.1%). A significant percentage (18.2%) of patients had a congenital or acquired upper or lower airway anomaly (Tables 23.3 and 23.4). Table 23.2 The number of patients with concomitant congenital syndromes and laryngoscopy grade [23] Abbreviation: CML, Cormack and Lehane classification During embryologic development, the tracheobronchial tree and the pulmonary vasculature develop together, and therefore, abnormalities of the pulmonary vasculature are often associated with abnormalities of the airway. This was confirmed in a review of 564 patients with tetralogy of Fallot/pulmonary atresia/major aortopulmonary collateral arteries (TOF/PA/MAPCAs) where 37% of the patients had velocardiofacial syndrome. Difficult intubation was noted in 5% of patients, and the incidence was not different among those with or without a chromosome anomaly [25]. Over 40% of the patients had perioperative bronchoscopy and/or chest CT scan and half of these patients had an upper or lower airway abnormality. Table 23.3 Incidence of congenital and acquired airway abnormalities Source: Reproduced with permission from Foz et al. [24]. Table 23.4 Patient demographics and intubation details Source: Reproduced with permission from Foz et al. [24]. Abbreviations: IQR, interquartile range. Efficient airway management with minimal periods of interrupted oxygenation and ventilation is of the utmost importance in infants and children with heart disease. Especially at risk are patients with cyanotic lesions, poor ventricular function (systolic and/or diastolic), and pulmonary hypertension. In children with cyanotic heart disease, the oxygen saturation of coronary blood is decreased. Additional oxygen desaturation during airway management may result in critically diminished oxygen delivery to cardiac myocytes. Coupled with the increase in plasma catecholamines associated with airway maneuvers, causing increased myocardial oxygen demand, significant myocardial ischemia may ensue. Children with low cardiac output have compromised coronary blood flow at baseline, and a decrease in oxygen saturation in coronary blood occurring while oxygen demand is increased during airway management may also result in severe myocardial hypoxia‐ischemia. In children with pulmonary hypertension, a similar increase in plasma catecholamine concentrations coupled with any rise in PaCO2 may cause an acute exacerbation in pulmonary vascular resistance and cardiac arrest. While anesthetic agents may prevent marked increases in sympathetic response to laryngoscopy and tracheal intubation, they may also cause myocardial depression and systemic hypotension, resulting in reduced coronary blood flow causing ischemia. Given these elevated risks for decompensation, and the relatively high frequency of airway anomalies, robust pre‐operative airway assessment and the preparation for a potential difficult intubation (e.g., ensuring availability of a video laryngoscope) is recommended. A modification of the American Society of Anesthesiologists algorithm for the management of the patient with a difficult airway can be applied to children [26] (Figure cssStyle=” cssStyle=”font-family:monospace” 23.1). Specialized airway equipment should be prepared, checked and available in the operating room. Box 23.1 lists recommendations for equipping a difficult airway cart. In addition to equipment, additional personnel skilled in airway management should be immediately available for assistance. When the difficult airway is recognized prior to the induction of anesthesia, control of the airway can be performed with the patient awake, following sedation, or after the induction of anesthesia with inhalation agents. Awake, non‐sedated, direct laryngoscopy can be accomplished in neonates but may be difficult, traumatic, and have significant adverse hemodynamic consequences. Placement of a supraglottic airway (SGA) after application of topical anesthesia to the airway in an awake infant has been described as an alternative to awake direct laryngoscopy [27]. Fiberoptic‐guided intubation of the older, cooperative patient using topical anesthesia and intravenous sedation is a safe and effective alternative. Maintaining oxygenation via nasal cannula, nasopharyngeal airway with 15 mm connector, modified Ring‐Adair‐Elwyn ETT, or heated humidified high‐flow nasal cannula should be considered during difficult airway management [28]. Figure 23.1 Difficult airway infographic: Pediatric patient example. (A) Time Out for identification of the airway management plan. A team‐based approach with identification of the following is preferred: the primary airway manager and backup manager and role assignment, the primary equipment and the backup equipment, and the person(s) available to help. Contact an ECMO team/otolaryngologic surgeon if noninvasive airway management is likely to fail (e.g., congenital high airway obstruction, airway tumor, etc.). (B) Color scheme. The colors represent the ability to oxygenate/ventilate: green, easy oxygenation/ventilation; yellow, difficult or marginal oxygenation/ventilation; and red, impossible oxygenation/ventilation. Reassess oxygenation/ventilation after each attempt and move to the appropriate box based on the results of the oxygenation/ventilation check. (C) Nonemergency pathway (oxygenation/ventilation adequate for an intubation known or anticipated to be challenging): deliver oxygen throughout airway management; attempt airway management with the technique/device most familiar to the primary airway manager; select from the following devices: supraglottic airway, videolaryngoscopy, flexible bronchoscopy, or a combination of these devices (e.g., flexible bronchoscopic intubation through the supraglottic airway); other techniques (e.g., lighted stylets or rigid stylets may be used at the discretion of the clinician); optimize and alternate devices as needed; reassess ventilation after each attempt; limit direct laryngoscopy attempts (e.g., one attempt) with consideration of standard blade videolaryngoscopy in lieu of direct laryngoscopy; limit total attempts (insertion of the intubating device until its removal) by the primary airway manager (e.g., three attempts) and one additional attempt by the secondary airway manager; after four attempts, consider emerging the patient and reversing anesthetic drugs if feasible. Clinicians may make further attempts if the risks and benefits to the patient favor continued attempts. (D) Marginal/emergency pathway (poor or no oxygenation/ventilation for an intubation known or anticipated to be challenging): treat functional (e.g., airway reflexes with drugs) and anatomical (mechanical) obstruction; attempt to improve ventilation with facemask, tracheal intubation, and supraglottic airway as appropriate; and if all options fail, consider emerging the patient or using advanced invasive techniques. (E) Consider a team debrief after all difficult airway encounters: identify processes that worked well and opportunities for system improvement and provide emotional support to members of the team, particularly when there is patient morbidly or mortality. Developed in collaboration with the Society for Pediatric Anesthesia and the Pediatric Difficult Intubation Collaborative: John E. Fiadjoe, M.D., Thomas Engelhardt, M.D., Ph.D., F.R.C.A., Nicola Disma, M.D., Narasimhan Jagannathan, M.D., M.B.A., Britta S. von Ungern‐Sternberg, M.D., Ph.D., D.E.A.A., F.A.N.Z.C.A., and Pete G. Kovatsis, M.D., F.A.A.P [26]. Inhalation induction of anesthesia using sevoflurane with maintenance of spontaneous ventilation is commonly performed in infants and children with a difficult airway. Intravenous access is established prior to mask induction. If the patient develops airway obstruction during the induction of anesthesia, immediate attempts are made to relieve the obstruction by head extension, jaw thrust, use of continuous positive airway pressure, and insertion of an oropharyngeal or nasopharyngeal airway. Oropharyngeal airway use may be of particular benefit in patients with glossomegaly such as those with Trisomy 21. If mask ventilation is inadequate and not improved with the above maneuvers, insertion of an SGA should be attempted. If positive pressure ventilation can be delivered via the SGA, the SGA can be used as a guide for insertion of an ETT or can be exchanged for an ETT with the use of a fiberoptic bronchoscope (FOB). If an SGA fails to provide a patent airway, preparations should be made for an emergency cricothyrotomy (see below). If mask ventilation is possible with either a face mask or SGA, neuromuscular relaxation can be used in order to optimize laryngoscopy. Recent data indicates that neuromuscular blockade can facilitate difficult mask ventilation and successful tracheal intubation. Moreover, with the ability of sugammadex at 16 mg/kg dose to reverse even profound neuromuscular blockade from non‐depolarizing drugs, this approach can now be utilized with more confidence [3]. If mask ventilation is possible, but tracheal intubation is not readily achieved, one should optimize head and neck positioning and consider use of an alternate laryngoscope blade or video‐laryngoscope to better visualize the larynx (the latter is often utilized as a primary device when difficult intubation is anticipated). Repeated attempts at direct laryngoscopy may lead to swelling and/or bleeding in the airway and the inability to perform adequate mask ventilation. After 3 failed attempts at direct laryngoscopy, the use of an SGA should be considered. For the majority of pediatric patients, insertion of an SGA provides a patent airway, a method of delivering positive pressure ventilation, and facilitates fiberoptic intubation. For the patient with the known difficult airway requiring endotracheal intubation (e.g., those with predictably lengthy OR courses or predicted to require mechanical ventilation after surgery), visualization of the airway may be aided by video laryngoscopy. Devices such as the Glidescope video laryngoscope (Verathon Medical, Bothwell, WA, USA) provide a highly angulated blade with a distal camera lens. The curve of this blade is designed to visualize structures “behind” the anatomic curve of the larynx, thereby aiding practitioners in obtaining a meaningful view of the vocal cords. The device is available in a wide range of pediatric sizes and some clinical studies demonstrating reduced time to intubation through its use [29]. However, the curve of this device requires a unique placement technique and not all practitioners may be familiar with its use. In addition, the disposable plastic blade cover is bulky and may interfere with passage of the ETT. The C‐MAC (Karl Storz Endoscopy, Tuttlingen, Germany) couples standard Miller and Macintosh blades of multiple sizes with a distal fiberoptic lens. This allows for both direct laryngoscopy and/or video laryngoscopy using one device. Moreover, the angulation and distal position of the fiberoptic lens can provide an improved view of the difficult airway. Several studies have demonstrated improved pediatric intubation times with the use of this device [30]. Video laryngoscopy has become an essential tool in the management of the difficult intubation in patients of all ages, and a number of devices are available. Recent studies report the first attempt success rate is significantly higher with a video larygoscope than standard direct laryngoscopy in infants with normal airways [31]. In addition, a standard‐blade video laryngoscope has a higher success rate on the first attempt (and eventual successful intubation) compared to a hyper‐angulated blade video laryngoscope among infants less than 5 kg [32] (Figure 23.2). The development of FOBs with small diameters has facilitated the use of fiberoptic‐guided intubation in infants and young children. The FOBs designed for use with a 3.5 mm ID ETT or smaller do not have an associated suction channel. Fiberoptic guided tracheal intubation can be performed via the nose, mouth, or LMA under sedation and topical anesthesia or under general anesthesia. The nasal route may be preferred because it tends to maintain the scope in the midline, facilitating visualization of the larynx. Repeated practice in children with normal airways is recommended in order to acquire and maintain the skills essential to be proficient with the use of the FOB. Fiberoptic‐guided tracheal intubation can also be performed through a supraglottic airway device (SGA)(Figure 23.3) [28]. This technique is reported to have higher first attempt success rate than video‐laryngoscopy (54% vs. 36%) in infants less than 1 year of age with difficult intubations; with no difference at older ages [33]. Once the SGA is appropriately positioned, the FOB is passed through the opening of the SGA into the trachea. An ETT is passed into the trachea over the FOB, which is then used to confirm appropriate positioning. When a standard ETT is used, only a short length of the ETT remains outside the SGA, making maintenance of ETT position and removal of the LMA difficult. A variety of techniques have been described to facilitate safe removal of the LMA while maintaining the ETT in place. First, a specially designed, long ETT can be placed over the FOB. Second, a tracheal tube exchange catheter (Cook Critical Care, Inc., Bloomington, IN) can be passed through the ETT into the trachea after which both the ETT and SGA are removed. An ETT is passed over the exchange catheter into the trachea. Third, the ETT can be made temporarily longer by wedging a half size smaller ETT into the proximal end, or by cutting the 15 mm adapter and using it to connect two ETTs of the same size together. After placement of the combined ETTs through the SGA, the SGA and proximal ETT are removed and the 15 mm adapter is replaced. Lastly, long forceps typically used for rigid bronchoscopy, can be used to hold the ETT while the SGA is removed. An alternative to fiberoptic intubation through the SGA, is “blind” passage of the ETT through the SGA into the trachea. Intubating SGAs have been developed for this purpose and are now available as smaller sizes. In younger children, the epiglottis frequently folds inside the SGA [34]. While this may not cause airway obstruction, traumatic injury to the epiglottis may occur from blind passage of an ETT. Figure 23.2 Direct laryngoscopy and video laryngoscopy blades demonstrating different angulations. From left to right: Miller 1 DL blade, Stortz C‐Mac Miller 1VL blade (standard blades); Truview EVO, McGrath series 5 Mac 3 blade, King Vision VL blade, Storz C‐Mac Pedi‐D blade, Glidescope Cobalt AVL sheath, Glidescope Spectrum blade (all hyperangulated). (Source: Peyton et al. [32]. Reproduced with permission from Elsevier.) Figure 23.3 Supraglottic airway with endotracheal tube, bronchoscopic swivel adapter, and anesthesia circuit. (Source: Hsu & Fiadjoe [28]. Reproduced with permission from Cambridge University Press.) Several other methods for controlling the airway of patients with a difficult airway have been described. These include the use of a lighted stylet, Combitube ® (esophageal tracheal airway), retrograde transtracheal intubation, blind nasal intubation, use of other specialized laryngoscopes with or without video capability, and digital (tactile) techniques. The reader is referred to a textbook of general pediatric anesthesiology for more detailed descriptions of these alternative techniques for intubation. In the rare instance in which mask ventilation and placement of an SGA do not provide adequate oxygenation and ventilation and tracheal intubation cannot be performed, percutaneous cricothyrotomy may be lifesaving. Percutaneous tracheotomy kits are available (Cook Critical Care, Inc., Bloomington, IN) that are designed to facilitate pediatric tracheotomy tube placement through the cricoid membrane using the Seldinger technique [35].
CHAPTER 23
Airway and Ventilatory Management
Introduction
Choosing the appropriate endotracheal tube (ETT)
Age
Size (mm ID)
Preterm
<1000 gm
2.5
1000–2500 gm
3.0
Term Neonate – 6 months
3.5
6 months to 1 year
4.0
1–2 years
4.0–5.0
Beyond 2 years
Orotracheal vs. nasotracheal intubation
The difficult airway
Syndrome
CML I, II
CML III, IV
Microdeletion 22q11 / DiGeorge
6
4
VACTERL / CHARGE syndrome
5
3
Ullrich‐Turner syndrome
5
0
Goldenhar syndrome
0
1
Pierre Robin sequence
0
1
Marfan syndrome
4
0
Patients
n = 4797n (%)
Airway abnormality
Acquired airway abnormality
463 (9.7)
Vocal paralysis unilateral partial
179 (3.7)
Vocal paralysis unilateral total
137 (2.9)
Bronchomalacia
103 (2.1)
Subglottic stenosis
73 (1.5)
Vocal paralysis bilateral partial
15 (0.3)
Vocal paralysis bilateral total
2 (0.05)
Congenital airway abnormality
408 (8.5)
Laryngomalacia
227 (4.7)
Bronchomalacia
223 (4.6)
Laryngeal cleft
199 (4.1)
Subglottic stenosis
171 (3.6)
Tracheomalacia, tracheal stenosis, tracheal disease
134 (2.8)
Agenesis of lung
48 (1.0)
Variable
Median (IQR) or n (%)
N (patients)
4,797
Age (y)
1.3 (0.2–6.0)
Weight (kg)
9.2 (4.3–19.2)
Sex
Female
2,156 (45)
Male
2,638 (55)
Prematurity (≤36 weeks)
1,141 (23.8)
Airway (n = 8,657 patient‐encounters)
Airway in situ
1,724 (20.0)
Tracheostomy
29 (0.3)
Natural airway
46 (0.5)
Intubated at time of the procedure
6,858 (79.2)
Intubation attempts (n = 6,139 intubated patient‐encounters)
1
5,359 (87.3)
2
628 (10.2)
3
119 (1.9)
4
20 (0.3)
5+
13 (0.2)
Intubation technique
Direct laryngoscopy
5,567 (92.3)
Video laryngoscopy
462 (7.7)
Flexible fiberoptic
4 (0.1)
Outcomes
Difficult intubation
53/4,797 (1.1)
Intubation of the patient with a difficult airway
Fiberoptic‐guided tracheal intubation
Emergency cricothyrotomy
The difficult extubation
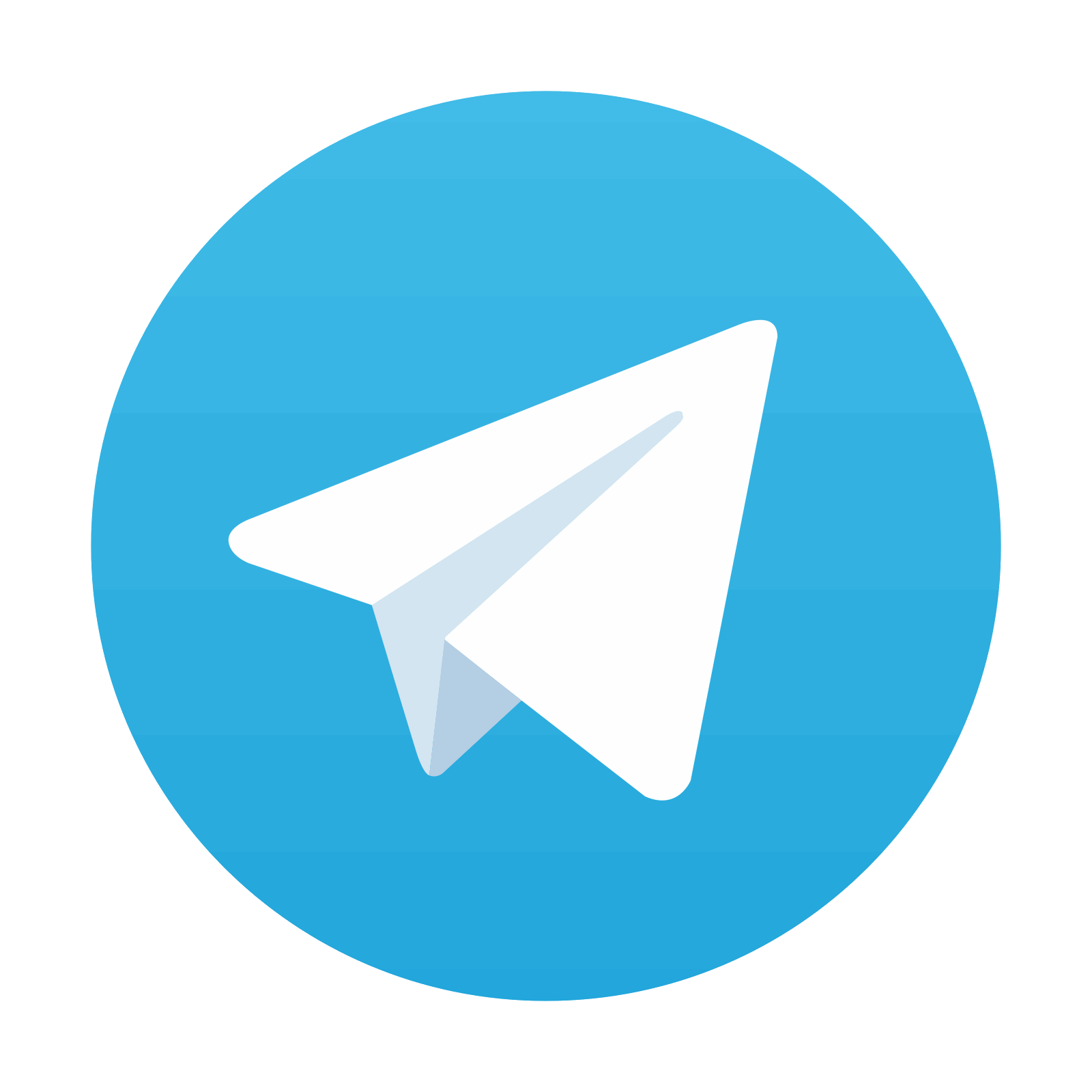
Stay updated, free articles. Join our Telegram channel

Full access? Get Clinical Tree
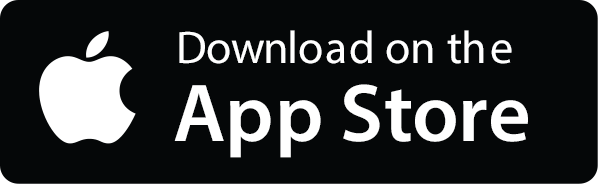
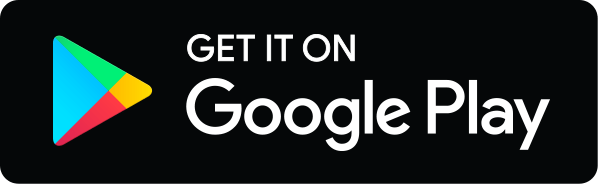