The most crucial component of infection prevention is frequent and effective hand hygiene.
The ideal hand hygiene agent kills a broad spectrum of microbes, has antimicrobial activity that persists for at least 6 hours after application, is simple to use, and has few side effects.
Wearing gloves does not reduce the need for hand hygiene.
Antibiotic prophylaxis has become standard for surgeries in which there is more than a minimum risk of infection. The most commonly used antibiotic for surgical prophylaxis is cefazolin, a first-generation cephalosporin, as the potential pathogens for most surgeries are gram-positive cocci from the skin.
The exact timing for the administration of the antibiotic depends on the pharmacology and half-life of the drug, but should generally be 0 to 60 minutes before incision. Prophylactic antibiotics should be discontinued by 24 hours following surgery if postoperative dosing is selected at all. Prolonging the course of prophylactic antibiotics does not reduce the risk of infection but does increase the risk of adverse consequences of antibiotic administration, including resistance, Clostridium difficile infection, and sensitization.
Anesthesiologists should work in consultation with surgeons to use guidelines determined by the local infection control committee to take initiative for administering prophylactic antibiotics because they have access to the patient during the 60 minutes prior to incision and can optimize timing of administration.
The standard teaching that oxygen delivery depends more on hemoglobin-bound oxygen (oxygen content) than on arterial PO2 may be true of working muscle, but it is not true of wound healing.
Although oxygen consumption is relatively low in wounds, it is consumed by processes that require oxygen at a high concentration.
High oxygen tensions (>100 mm Hg) can be reached in wounds but only if perfusion is rapid and arterial PO2 is high.
Peripheral vasoconstriction, which results from central sympathetic control of subcutaneous vascular tone, is probably the most frequent and clinically the most important impediment to wound oxygenation.
Prevention or correction of hypothermia and blood volume deficits has been shown to decrease wound infections and increase collagen deposition in patients undergoing major abdominal surgery.
Modifiable risks include smoking, malnutrition, obesity, hyperglycemia, hypercholesterolemia, and hypertension. These should be assessed and corrected when possible prior to surgery.
Maintenance of a high room temperature or active warming before, during, and after the operation is significantly more effective than other methods of warming, such as circulating water blankets placed on the surface of the operating table and humidification of the breathing circuit.
Optimizing the volume of perioperative fluid administration to minimize morbidity and mortality remains a significant and controversial challenge.
Current best recommendations for volume management include replacing fluid losses based on standard recommendations for the type of surgery, replacement of blood loss, and replacement of other ongoing fluid losses (e.g., high urine output due to diuretic or dye administration, hyperglycemia, or thermoregulatory vasoconstriction).
Wounds are most vulnerable in the first few hours after surgery.
All vasoconstrictive stimuli must be corrected simultaneously to allow optimal healing.
Local perfusion is not assured until patients have a normal blood volume, are warm and pain-free, and are receiving no vasoconstrictive drugs; that is, until the sympathetic nervous system is inactivated.
Urine output is a poor, often misleading guide to peripheral perfusion.
Physical examination of the patient is a better guide to hypovolemia and vasoconstriction.
Administration of supplemental oxygen via face mask or nasal cannulae increases safety in patients receiving systemic opioids. As a side benefit, it may also improve wound healing. Pain control also appears important since it favorably influences both pulmonary function and vascular tone.
In patients with moderate to high risk of surgical site infection, anesthesiologists have the opportunity to enhance wound healing and reduce the incidence of wound infections by simple, inexpensive, and readily available means.
Multimedia
Oxygen Tension in Wound Module
Despite major advances in the management of patients undergoing surgery—including aseptic technique, prophylactic antibiotics, and advances in surgical approaches such as laparoscopic surgery—surgical wound infection and wound failure remain common complications of surgery (Fig. 13-1). Wound complications are associated with prolonged hospitalization, increased resource consumption, and even increased mortality. More than 300,000 surgical site infections (SSIs; Table 13-1) occur each year in the United States at an estimated cost of more than $1 billion.2 A growing body of literature supports the concept that patient factors are a major determinant of wound outcome following surgery. Comorbidities such as diabetes and cardiac disease clearly contribute, but environmental stressors as well the individual response to stress may be equally important. In particular, wounds are exquisitely sensitive to hypoxia, which is both common and preventable. Perioperative management can be adapted to promote postoperative wound healing and resistance to infection. Along with aseptic technique and prophylactic antibiotics, maintaining perfusion and oxygenation of the wound is paramount. This chapter discusses how knowledge of the principles of infection control and the biology and physiology of wound repair and resistance to infection can improve outcomes.
FIGURE 13-1. Thomas et al.1 reviewed the records of 15,000 nonpsychiatric patients discharged from a representative sample of Utah and Colorado hospitals in 1992 for adverse events. There were 17,912 adverse events identified, or 2.9 ± 0.2% of hospitalizations. Of these, almost half (45%) were related to operative care. The graph shows the distribution of adverse events within the subcategory of operative care (7,716 operative adverse events). Note that about 20% were infection related and about 15% were wound related. SSI, surgical site infection; HCAI, health care–associated infection; DVT, deep venous thrombosis; MI, myocardial infarction; PE, pulmonary embolus; CHF, congestive heart failure; CVA, cerebrovascular accident. (Data from: Thomas EJ, Studdert DM, Burstin HR, et al. Incidence and types of adverse events and negligent care in Utah and Colorado. Med Care 2000;38:261–271.)
INFECTION CONTROL
Hand Hygiene
Perhaps the most crucial component of infection prevention is frequent and effective hand hygiene. In 1847 Ignaz Semmelweis made the observation that women who delivered their babies in the First Clinic at the General Hospital of Vienna, staffed by medical students and physicians, had a mortality rate of 5% to 15%, largely the result of puerperal infections; this was substantially higher than the 2% rate of women who delivered at Clinic 2, which was staffed by midwife students and midwives.3 Students and physicians at Clinic 1 usually started the day performing autopsies (including on patients who died of puerperal fever) and then moved on to the clinic, where they performed examinations on women in labor. Semmelweis made the connection, and although germ theory was some years off, he insisted that physicians and medical students wash their hands in a chlorinated solution when leaving the pathology laboratory. This reduced the rate of puerperal fever to the same rate as at Clinic 2. Soon, Semmelweis identified cases of transmission from an infected to an uninfected patient, and instituted the use of chlorinated solution hand washing between cases as well. He also demonstrated that the chlorinated solution was more effective than soap and water. Unfortunately, his innovation was not widely adopted, resulting from a combination of his delay in publishing his results, the reluctance of his colleagues to accept that they might be responsible for transmitting disease, and his lack of tact in trying to convince health care workers to adopt his measures. Despite our current knowledge of germ theory, hand hygiene remains an inexplicably neglected component of infection control: Studies consistently demonstrate about a 40% rate of adherence (range, 5% to 81%) to hand hygiene guidelines.4
TABLE 13-1. CRITERIA FOR DEFINING A SURGICAL SITE INFECTION (SSI)
Bacteria are resident in the skin and can never be completely eliminated.4 Resident flora are embedded in the deeper folds of the skin and are more resistant to removal, but are also infrequently pathogenic. Coagulase-negative staphylococci and diphtheroids are the most common. Transient flora colonize the superficial layers of the skin and thus are easier to remove with hand hygiene. Transient flora are also the source of most health care–associated infections, as health care worker skin can become contaminated from patient contact or contact with contaminated surfaces. Contamination from surfaces is most commonly with organisms such as staphylococci and enterococci, which are resistant to drying. Even “clean” activities such as taking a patient’s pulse or applying monitors can lead to hand contamination: 100 to 1,000 colony-forming units of Klebsiella species were measured on nurses’ hands following such activities in one study.5 No studies have related hand contamination to actual transmission of infection to patients; however, numerous studies, starting with those of Semmelweis, have demonstrated a reduction in health care–associated infections following institution of hand hygiene or improved adherence to hand hygiene.4
A number of products are available for hand hygiene. The ideal agent kills a broad spectrum of microbes, has antimicrobial activity that persists for at least 6 hours after application, is simple to use, and has few side effects. The most commonly used and efficacious agents are reviewed here.
Plain (not antiseptic) soap and water are generally the least effective at reducing hand contamination.6 Although obvious dirt is removed by the detergent effect of soap and the mechanical action of washing, bacterial load is not greatly reduced. Further, soap and water hand hygiene is associated with high rates of skin irritation and drying, both of which are risk factors for an increased bacterial load. Soap and water are, however, the most effective at removing spores, and therefore should be used when contamination with Clostridium difficile or Bacillus anthracis is a concern.4
Alcohol-based rinses, gels, and foams denature proteins, and this confers their antimicrobial activity.4 Ethanol is most commonly used because it has more antiviral activity than isopropanol. Antiseptics containing 60% to 95% ethanol with a water base are germicidal and effective against gram-positive and gram- negative bacteria, lipophilic viruses such as herpes simplex, human immunodeficiency, influenza, respiratory syncytial, and vaccinia viruses, and hepatitis B and C viruses. They have little persistent activity, although regrowth of bacteria does occur slowly after use of alcohol-based products. Combination with low doses of other agents such as chlorhexidine, quaternary ammonium compounds, or triclosan can confer persistent activity. Efficacy depends on volume applied (3 mL is superior to 1 mL) and duration of contact (ideally, 30 seconds).
Chlorhexidine is a cationic bisbiguanide that disrupts cytoplasmic membranes, resulting in precipitation of cellular contents.4 It is germicidal against gram-positive bacteria and lipophilic viruses, with somewhat less activity against gram-negative bacteria and fungi, and minimal against tubercle bacilli. It has substantial persistence on the skin, and the Centers for Disease Control and Prevention (CDC) has identified it as the topical agent of choice for skin preparation in central venous catheter insertion. It may cause severe corneal damage after direct contact with the eye, ototoxicity after direct contact with the inner or middle ear, and neurotoxicity after direct contact with the brain or meninges. There are reports of bacteria that have acquired reduced susceptibility to chlorhexidine, but these are of questionable clinical pertinence since the concentrations at which resistance was found were substantially lower than that of commercially available products. Recent reports have identified immunoglobulin E–mediated allergic reactions to chlorhexidine.7 Cases are likely underreported because of the difficulty identifying the source of anaphylactic reactions perioperatively. Chlorhexidine is present in a wide range of medical and community based products, including wipes, impregnated central venous catheters, toothpaste, mouthwash, contact lens cleanser, and food preservatives. Therefore, potentially sensitizing exposures are common.
Iodine and iodophors (iodine with a polymer carrier) penetrate the cell wall and impair protein synthesis and cell membrane function.4 They are bactericidal against gram-positive, gram- negative, and some spore-forming bacteria including clostridia and Bacillus species, although inactive against spores. They also have activity against mycobacteria, viruses, and fungi. Their persistence is generally fairly poor. They cause more contact dermatitis than other commonly used agents, and allergies to this class of topical agent are common. Iodophors generally cause fewer side effects than iodine agents.
The choice of an antiseptic depends on the expected pathogens, acceptability by health care workers, and cost. In general, antiseptics cost about $1 per patient day, far less than the cost of health care–associated infections. In nine studies that examined the effect of improved hand hygiene adherence on health care–associated infections, the majority demonstrated that as hand hygiene practices improved, infection rates decreased.4
Barriers to hand hygiene include skin irritation and fear of skin irritation, inaccessibility, time, and health care worker acceptance (largely related to the other factors mentioned). Although alcohol-based agents have long been believed to cause more skin irritation, several recent trials have demonstrated less skin irritation and better acceptance with emollient-containing, alcohol-based hand rubs compared with either antimicrobial or nonantimicrobial soaps. The use of appropriate (glove-compatible) lotions twice a day also reduces skin irritation—as well as leading to a 50% increase in hand hygiene frequency in one study.4 Alcohol-based gels and foams are also generally more accessible than antiseptic soap and water, as the dispenser may be pocket-sized or placed conveniently near sites of patient care. It has been estimated that alcohol-based gels and foams require only about 25% of the time of going to a sink to wash one’s hands. However, soap and water should be used to remove particulate matter including blood and other body fluids or after five to ten applications of alcohol-based agent.
Adherence to hand hygiene guidelines (Tables 13-2 to 13-4) generally decreases as the frequency of indicated hand washing increases, as the workload increases, and as staffing decreases. CDC guidelines for health care providers traditionally focused on hand hygiene prior to entering and after leaving a patient room. More recently, the World Health Organization has developed a campaign highlighting the “5 Moments” of hand hygiene (Fig. 13-2). The campaign emphasizes the need to perform hand hygiene after each contact with a patient or their immediate environment.8
In an intensive care unit (ICU), hand hygiene for nurses is generally indicated about 20 times per hour, as compared with a normal ward where this number decreases to 8 times per hour.4 In the operating room (OR), frequent patient contact by the anesthesiologist requires frequent hand hygiene, probably at about the level of nurses in the ICU, while accessibility is often quite limited. Sinks are available only outside the OR. Therefore, alcohol-based agents should be available within hand’s reach of the anesthesia machine. Loftus et al.9 studied bacterial contamination of the anesthesia work area (adjustable pressure limiting valve complex and agent flowmeter) and cross-contamination of the sterile anesthesia stopcock during 61 first cases in their OR. They found an average increase in bacterial contamination of the work area of 115 colonies per surface area sampled during cases (95% confidence interval: 62–169; p < 0.001). Transmission of bacteria from the work area to the sterile stopcock in the patients’ intravenous tubing occurred in 32% of cases, including transmission of methicillin-resistant Staphylococcus aureus (MRSA) in two cases and vancomycin-resistant Enterococcus in one case. A high level of contamination of the work area (>100 colonies per surface area sampled) increased the risk of stopcock contamination 4.7 fold (95% confidence interval: 1.42–15.42; p = 0.011).
In a follow-up study, Koff et al.10 demonstrated that increased hand hygiene episodes (7–9 per hour compared to <0.5 per hour during the control period) triggered by an alarm and encouraged by education decreased work area contamination, decreased stopcock contamination from 32% to 8%, and decreased health care–associated infections significantly. Opportunities were not measured and hand hygiene episodes were not necessarily coordinated with one of the 5 Moments. Thus, transmission of bacterial contamination by the anesthesia provider appears to be common, a potential source of nosocomial infections, and largely preventable.9 Frequent hand hygiene by anesthesia providers has a direct and positive impact on patient outcomes.
Wearing gloves does not reduce the need for hand hygiene. Although gloves provide protection, bacterial flora from patients may be cultured from up to 30% of health care workers who wear gloves during patient contact.4 Therefore, hand hygiene should be practiced both before putting on gloves and immediately after removal. Moreover, gloves should be removed or changed immediately after each procedure, including vascular access, intubation, and neuraxial anesthesia, because gloves become contaminated by patient contact just as hands do. Balancing hand hygiene with close attention to the patient during critical portions of the case (e.g., securing the airway) can be challenging. Double gloving and providing a convenient location for contaminated equipment have been suggested as effective approaches.11
TABLE 13-2. INDICATIONS FOR HAND HYGIENE
TABLE 13-3. HAND HYGIENE TECHNIQUE
Artificial and long fingernails, as well as chipped fingernail polish, are associated with higher concentrations of bacteria on the hands of health care workers. Artificial nails have been identified as a source in several hospital-associated outbreaks of infection with gram-negative bacilli and yeast, and CDC guidelines discourage wearing of artificial nails by health care workers in high-risk settings; many hospitals have banned wearing of artificial nails by any employee who has direct patient contact.4 It may also be appropriate to counsel patients scheduled for surgery that artificial nails may increase their risk of infection, although this has not been investigated. Large quantities of bacteria are typically trapped under the fingernails, and 2002 CDC guidelines recommend that health care workers keep their nail tips trimmed to less than ¼ inch.4
TABLE 13-4. SKIN CARE
FIGURE 13-2. World Health Organization schematic of the “5 Moments” for hand hygiene.
Bacteria may be cultured at higher concentrations from the skin beneath a ring. On the other hand, wearing a ring does not increase overall bacterial levels measured on the hands of health care workers. Therefore, it remains unclear whether transmission of infection could be reduced by prohibiting health care workers from wearing rings.4
Antisepsis
Masks have long been advocated as preventing SSI, and are used almost universally in the US operating rooms. Tunevall.12 studied the rate of wound infections in 3,088 patients over 115 weeks. In alternating weeks, OR personnel either wore masks or did not (personnel with active respiratory infections continued to wear masks). There was no difference in the rate of surgical wound infections (4.7% vs. 3.5%, respectively) in the two groups, nor in bacterial species cultured from the wounds. Friberg et al.13 demonstrated comparable air and surface contamination during sham surgery in a horizontal laminar airflow unit whether OR personnel wore a nonsterile hood and mask or a sterilized helmet aspirator system. When the head cover but not the mask was omitted, however, contamination increased three- to fivefold. These data suggest that wearing a head cover is useful for preventing SSI, while wearing a mask is not. Nonetheless, the study by Tunevall is a small one, and most hospital personnel continue to require a mask in the OR while surgical instruments are open. Moreover, the mask does serve the purpose of protecting the health care provider, particularly when combined with eye protection, and thus should most likely be used during tracheal intubation, emergence from anesthesia, and at other times when exposure to body fluids is likely.
Although the preponderance of postoperative surgical infections is caused by flora that are endogenous to the patient, environmental and airborne contaminants may also play a causative role. An important, but frequently overlooked, consideration is the role that traffic patterns into an OR can play in patient exposure to airborne organisms. A recent Israeli study of risk factors for surgical infection after total knee replacement demonstrated a trend toward increased infection rates within increased number of orthopedic surgeons or anesthetists present in the OR.14 This study reconfirmed a prior study showing a trend toward increased incidence of SSI as the number of people in the operating suite increases.15 However, it has been noted in one audit that physicians and nurses did little to limit the number of people through ORs during procedures.16 Current recommended practices are that traffic patterns should limit the flow of people through an OR that is in use, and that no more people than necessary should be in an OR during a procedure.17 The anesthesiologist is clearly in a position to play a leadership role in controlling human traffic through the OR.
Mermel et al.18 in 1991 demonstrated that central venous lines placed by the anesthesiologist in the OR became infected more often (relative risk [RR], 2.1; p = 0.03) than those placed by surgeons or other providers, whether in or out of the OR. Contributing factors appeared to be site of placement and the stringency of aseptic technique. Internal jugular vein insertion has a greater risk of infection (RR, 4.3; p < 0.01) compared with subclavian vein, although its other benefits may outweigh this risk. Raad et al.19 demonstrated that use of a maximal sterile barrier technique versus sterile gloves and small sterile drapes led to a significant reduction in central venous catheter-related infection from 7.2% to 2.2% (p = 0.03). Therefore, gowning and gloving, careful aseptic technique, and use of a wide sterile field should be routine.20 In anesthetized patients, the central line is ideally placed before the surgical site is draped in order to avoid contamination of the wire on the underside of the surgical drape.
Epidural abscess formation is an extremely rare but potentially catastrophic complication of neuraxial anesthesia and epidural catheter placement. Therefore, careful attention to aseptic technique and infection control is required. The most important consideration is to prevent contamination of the needle and catheter. Thus, hand washing, skin preparation, draping, and maintenance of a sterile field should be carefully observed. Gowning and wearing a mask likely play a smaller role, but are reasonable given the devastating consequences of infection. Finally, epidurals should probably be avoided in patients known or suspected to have bacteremia or deferred until after appropriate antibiotics are administered.
Antibiotic Prophylaxis
After antibiotics came into widespread use in the 1940s and 1950s, there was much debate over the possibility that antibiotic prophylaxis might prevent SSI. In 1957 Miles et al.21 used a guinea pig model for the proof of principle that administration of an antibiotic prior to contamination (incision) could reduce the risk of SSI. When appropriate antibiotics were given within 2 hours before or after intradermal injection of bacteria, they were effective in preventing invasive infection and necrosis. When given outside this window, they were not effective. This gave rise to the concept of a “decisive period” in which antibiotics will be effective, which remains a guiding principle of antibiotic prophylaxis. Miles et al. also demonstrated that injection of epinephrine intradermally prior to administration of antibiotics led to antibiotic failure, as demonstrated in an increased wound infection rate. This demonstrated the crucial role of local perfusion in delivering antibiotics to the site. Knighton et al.,22 using the same model, demonstrated that increased inspired oxygen was equally as effective as antibiotics in preventing infection, and that the two effects were additive (Fig. 13-3). Knighton et al.23 also delayed the administration of oxygen for up to 6 hours after inoculation and demonstrated no reduction in effect. Thus, the decisive period for oxygen is considerably longer than that of antibiotics.
FIGURE 13-3. The effect of oxygen and/or antibiotics on lesion diameter after intradermal injection of bacteria into guinea pigs. Note that at every level, oxygen adds to the effect of antibiotics and that increasing oxygen in the breathing mixture from 12% to 20% or from 20% to 45% exerts an effect comparable to that of appropriately timed antibiotics. (From: Rabkin J, Hunt TK. Infection and oxygen, Problem wounds: The Role of Oxygen. Edited by Davis J, Hunt TK. New York, Elsevier, 1988, pp 1, with permission.)
Two surgeons at Washington University in St. Louis, Harvey Bernard and William Cole,24 reported on the first controlled clinical trial of the efficacy of antibiotic prophylaxis in 1964 and demonstrated a benefit in abdominal operations. Thereafter, numerous clinical trials were performed with somewhat variable results. Eventually these served to define the timing and population in which prophylactic antibiotics work. By the 1970s antibiotic prophylaxis for high-risk surgery—meaning clean-contaminated and contaminated cases—was becoming well accepted and widely used, although some skeptics remained. In 1992, Classen et al.25 published their prospective series including 2,847 patients undergoing clean or clean-contaminated surgical procedures at LDS Hospital in Salt Lake City, UT (Fig. 13-4). They demonstrated that the decisive period for SSI in humans undergoing surgery was essentially the same as for experimental infections in guinea pigs. That is, they found the lowest infection rate when antibiotics were given within 2 hours before or after incision and a rapid increase in SSI rate when they were given outside that range. The best results, though only by a small margin and not statistically significant, were within 0 to 60 minutes of surgery, and this subsequently became the clinical standard.
FIGURE 13-4. The figure demonstrates rates of surgical wound infection corresponding to the temporal relation between antibiotic administration and the start of surgery. The number of infections and the number of patients for each hourly interval appear as the numerator and denominator, respectively, of the fraction for that interval. The trend toward higher rates of infection for each hour that antibiotic administration was delayed after the surgical incision was significant (z score = 2.00; p < 0.05 by the Wilcoxon test). (From: Classen DC, Evans RS, Pestotnik KS, et al. The timing of prophylactic administration of antibiotics and the risk of surgical-wound infection. N Engl J Med. 1992:326;281, with permission.)
Antibiotic prophylaxis has now become standard for surgeries in which there is more than a minimum risk of infection. Although not every surgery and situation has been studied, a strong rationale for the approach to prophylactic antibiotics has emerged. Several groups separately developed guidelines for use, culminating in recommendations published in 2004 by the National Surgical Infection Prevention Project.26 These guidelines emphasize timing and choice of appropriate agents. Guidelines generally do not specify antibiotic agents, although they give rationales for various choices.26 The agent for antibiotic prophylaxis must cover the most likely spectrum of bacteria presented in the surgical field (see Tables 13-5 and 13-6). The most commonly used antibiotic for surgical prophylaxis is cefazolin, a first-generation cephalosporin, as the potential pathogens for most surgeries are gram-positive cocci from the skin.26,27
TABLE 13-5. RECOMMENDED DRUGS FOR COMMON PROCEDURES
TABLE 13-6. DRUGS AND DOSES AVAILABLE ROUTINELY FOR ANTIBIOTIC PROPHYLAXIS
By definition, prophylactic antibiotics are given pre- or intraoperatively. The exact timing for the administration of the antibiotic depends on the pharmacology and half-life of the drug. It has been suggested that administration of prophylatic antibiotics is ideal within 30 minutes to 1 hour of incision.27,28 Drugs given by bolus administration (e.g., cefazolin) achieve adequate tissue concentration rapidly, so that giving these drugs within 0 to 30 minutes of incisions appears equally efficacious. Giving the antibiotics too early (so that the incision is more than 60 minutes after the dose) is a recurrent issue at many hospitals, especially for cases that require complex patient positioning. Giving the antibiotics closer to the incision time prevents this problem. Providing timely prophylactic antibiotics is relatively uncomplicated for antibiotics that can be given as a bolus dose (e.g., cephalosporins) or as an infusion over a few minutes (e.g., clindamycin) and thus provide tissue levels within minutes. For drugs like vancomycin that require infusion over an hour, coordination of administration is more complex. In general, it is considered acceptable if the infusion is started prior to incision. When a tourniquet is used, the infusion must be complete prior to inflation of the tourniquet. An appropriate dose based on body weight and volume of distribution should be given. Depending on the half-life, antibiotics should be repeated during long operations or operations with large blood loss.29 For example, cefazolin is normally dosed every 8 hours but the dose should be repeated every 4 hours intraoperatively.29 Finally, prophylactic antibiotics should be discontinued by 24 hours following surgery if postoperative dosing is selected at all. Prolonging the course of prophylactic antibiotics does not reduce the risk of infection but does increase the risk of adverse consequences of antibiotic administration,22 including resistance, Clostridium difficile infection, and sensitization.
Unfortunately, MRSA is becoming a more common pathogen. Although it varies by country, region, and hospital, about 60% of S. aureus are MRSA. Independent risk factors identified for MRSA infection include prolonged use of prophylaxis, use of drains for more than 24 hours, and increasing number of procedures performed on the patient. Hand hygiene is among the most effective means of preventing development of MRSA since alcohol-based gel or foam used properly kills over 99.9% of all transient pathogens including MRSA. There does not appear to be a justification for using antibiotics effective against MRSA for prophylaxis in most clinical settings.
Because they have access to the patient during the 60 minutes prior to incision and can optimize timing of administration, anesthesiologists should work in consultation with the surgeon to use guidelines determined by the local infection control committee to take initiative for administering prophylactic antibiotics. In this way, anesthesiologists can make a major contribution to preventing SSI. The Centers for Medicare and Medicaid Services has identified timely and appropriate antibiotic prophylaxis administration as a cornerstone of SSI prevention. Physician and hospital reimbursements are increasingly tied to such performance measures, meaning anesthesiologists also have an economic interest in ensuring adherence to guidelines.
MECHANISMS OF WOUND REPAIR
Wound healing is a complex process, requiring a coordinated repair response including inflammation, matrix production, angiogenesis, epithelization, and remodeling (Fig. 13-5). Many factors may impair wound healing. Systemic factors such as medical comorbidities, nutrition,30 sympathetic nervous system activation,31 and age32–34 have a substantial effect on the repair process. Local environmental factors in and around the wound including bacterial load,35 degree of inflammation, moisture content,36 oxygen tension,37 and vascular perfusion38 also have a profound effect on healing. Although all of these factors are important, perhaps the most critical element is oxygen supply to the wound. Wound hypoxia impairs each of the components of healing.39
FIGURE 13-5. Schematic of the processes of wound healing. (From Hunt T. Fundamentals of wound management in surgery, wound healing: Disorders of Repair. South Plainfield, NJ, Chirugecom, Inc., 1976, with permission.)
Although the role of oxygen is usually thought of in terms of aerobic respiration and energy production via oxidative phosphorylation, in wound healing oxygen is required as a cofactor for enzymatic processes and for cell-signaling mechanisms. Oxygen is a rate-limiting component in leukocyte-mediated bacterial killing and collagen formation because specific enzymes require oxygen at a partial pressure of at least 40 mm Hg.40,41 The mechanisms by which the other processes are oxygen-dependent are less clear, but these processes also require oxygen at a concentration much above that required for cellular respiration.42–45
The Initial Response to Injury
A surgical incision disrupts the skin barrier, creating an acute wound, and an effective initial response to injury depends on the ability to clean foreign material and to resist infection. This response initiates a sequence of events that starts with any source of injury that disrupts homeostasis in the local environment and eventually leads to healing.
Wound healing has traditionally been described in four separate phases: Hemostasis, inflammation, proliferation, and remodeling.46 Considerable overlap exists between each of these phases, and differentiating precisely when one phase ends and the next begins is virtually impossible. Each phase is composed of complex interactions between host cells, contaminants, cytokines, and other chemical mediators that, when functioning properly, lead to repair of injury. These processes are highly conserved across species,47 indicating the critical importance of the inflammatory response that directs the process of cellular/tissue repair. When any component of healing is disturbed and interrupts the orderly progression of repair, wound failure may result.48
Injury damages the local circulation and causes platelets to aggregate and release a variety of substances, including chemoattractants and growth factors.46 The initial result is coagulation, which prevents exsanguination but also widens the area that is no longer perfused. Platelet degranulation releases platelet-derived growth factor, transforming growth factor beta (TGF-β), epidermal growth factor, and insulin-like growth factor-1 (IGF-1), which conjointly initiate the inflammatory process.46 Bradykinin, complement, and histamine released by mast cells cause vasodilation and increased vascular permeability. Polymorphonuclear leukocytes arrive at the wound almost immediately and are followed by macrophages at 24 to 48 hours. These inflammatory cells activate in response to endothelial integrins, selectins, cell adhesion molecules, cadherins, fibrin, lactate, hypoxia, foreign bodies, infectious agents, and growth factors.46 In turn, macrophages and lymphocytes produce more lactate49 and growth factors, including IGF-1, leukocyte growth factor, interleukins (ILs) 1 and 2, TGF-β, and vascular endothelial growth factor (VEGF).50 This early inflammatory phase is characterized by erythema and edema of the wound edges.
Activated neutrophils and macrophages also release proteases, including neutrophil elastase, neutrophil collagenase, matrix metalloproteinase, and macrophage metalloelastase.46 These proteases degrade damaged extracellular matrix components to allow their replacement. Proteases also degrade the basement membrane of capillaries to enable inflammatory cells to migrate into the wound.
In wounds, local blood supply is compromised at the same time that metabolic demand is increased. As a result, the wound environment becomes hypoxic and acidotic with high lactate levels.51,52 This represents the sum of three effects: (1) decreased oxygen supply due to vascular damage and coagulation, (2) increased metabolic demand due to the heightened cellular response (anaerobic glycolysis), and (3) aerobic glycolysis by inflammatory cells.53,54 Leukocytes contain few mitochondria and therefore acquire energy from glucose, primarily by production of lactate and even in the presence of adequate oxygen supply.53 In activated neutrophils, the respiratory burst, in which oxygen and glucose are converted to superoxide, hydrogen ion, and lactate, accounts for up to 98% of oxygen consumption; in the setting of injury, this activity increases by up to 50-fold over baseline.55,56
Local hypoxia is a normal and inevitable result of tissue injury.57,58 Hypoxia acts as a stimulus to repair,59 but also leads to poor healing37 and increased susceptibility to infection.60,61 Numerous experimental models21,60,62,63 as well as human clinical experience61,64 have led to the conclusion that wound healing is delayed in hypoxic wounds. The partial pressure of oxygen in dermal wounds is heterogeneous, ranging from 0 to 10 mm Hg in the central (“dead space”) portion of the wound, to 80 to 100 mm Hg (near arterial) adjacent to perfused arterioles and capillaries (Fig. 13-6).58 The PO2 of a given area depends on diffusion of oxygen from perfused capillaries, and thus wound PO2 depends on capillary density, arterial PO2, and the metabolic activity of the cells, with some contribution from shifts in the oxyhemoglobin dissociation curve associated with wound pH and temperature.
FIGURE 13-6. The varying oxygen tension in the wound module. Cross-section of the wound module in a rabbit ear chamber is in left upper corner of figure. Note that PO2, depicted graphically above the cross-section (red line), is highest next to the vessels, with a gradient down to zero at the wound edge. Note also the lactate gradient (green line), high in the dead space and lower (but still above plasma) toward the vasculature. Hydrogen peroxide (H2O2) is present at fairly high concentrations (blue line) and is also a major stimulus to wound repair.65 Growth factors such as VEGF are produced by inflammatory cells in the hypoxic, high lactate, high hydrogen peroxide “Signaling Zone” and then diffuse to the “Response Zone” where they act on fibroblasts and endothelial cells to promote healing. VEGF, vascular endothelial growth factor. (Modified version reprinted from: IA Silver. The physiology of wound healing, Fundamentals of wound management. Edited by TK Hunt, JE Dunphy. New York, Appleton-Century-Crofts, 1980, p 30, with permission.)
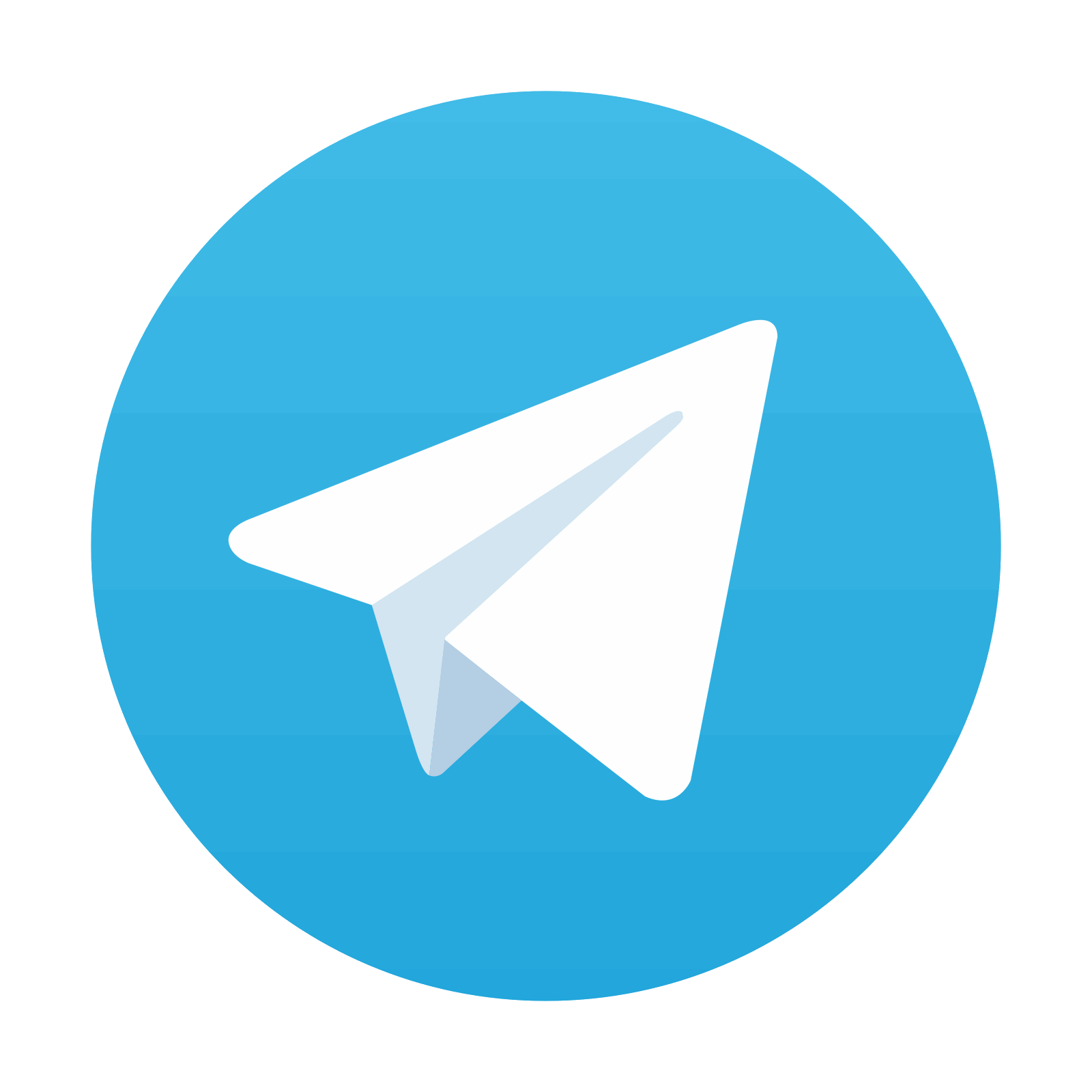
Stay updated, free articles. Join our Telegram channel

Full access? Get Clinical Tree
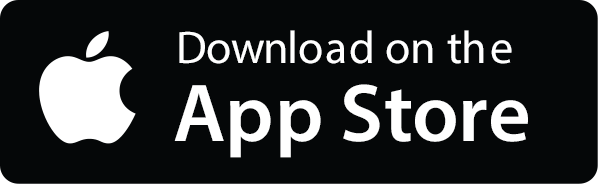
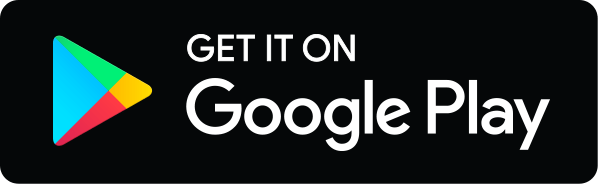