Positive End-Expiratory Pressure: Introduction
Positive end-expiratory pressure (PEEP) is not a ventilator mode itself, but rather an adjunctive treatment that can be combined with all forms of mechanical ventilation, both controlled and assisted,1–7 or applied to spontaneous breathing throughout the entire respiratory cycle, so-called continuous positive airway pressure (CPAP).8–10 Following the pioneering work of Poulton and Oxon11 and Barach and associates12 who demonstrated in the mid-1930s that application of positive pressure to the airway can effectively treat patients with pulmonary edema, several pathological conditions were proved to benefit from PEEP, which is today considered by intensive care unit physicians as one of the most powerful treatments available for acute respiratory failure (ARF).13
Since publication of the second edition of this book in 2006, several relevant studies have been published on PEEP and CPAP, primarily concerning lung injury and cardiogenic pulmonary edema. To update the chapter with this new information, we have condensed material that was covered in greater depth in the second edition.
Overview of Pertinent Pathophysiology
The reversal of hypoxemia caused by intrapulmonary shunt and venous admixture requires interventions that recruit more aerated lung units for ventilation. In patients with an acute reduction of lung volume secondary to lung edema and/or atelectasis, PEEP can improve arterial oxygenation1,8 by increasing functional residual capacity (FRC),14–19 reducing venous admixture,20–24 shifting tidal volume (VT) to a more compliant portion of the pressure–volume curve,25 preventing the loss of compliance during mechanical ventilation,5,26 reducing intratidal alveolar opening and closing27 and the work of breathing.28 Figure 10-1A summarizes the rationale for PEEP in patients with ARF secondary to acute lung volume reduction.
Figure 10-1
Respiratory effects of PEEP/CPAP in acute respiratory failure secondary to (A) lung volume reduction caused by edema and/or atelectasis and (B) airway obstruction and expiratory flow limitation. FRC, functional residual capacity; PaO2, partial pressure of arterial oxygen; PEEP, positive end-expiratory pressure.
Although there is general consensus about the potential of PEEP in treating patients with hypoxemic ARF secondary to lung edema and/or atelectasis, several aspects are controversial. For many years, it has been recognized that the actions of PEEP on gas exchange and pulmonary mechanics are variable,29,30 depending on the type and severity of lung injury, which may be sustained by different pathways31,32 and pathophysiologic mechanisms.31,33 As with any treatment, PEEP is not free of side effects.34 In patients with acute respiratory distress syndrome (ARDS), PEEP may recruit nonaerated regions, but also distend normally aerated regions,35,36 contributing to barotrauma through increase in end-inspiratory plateau pressure.22,37–40 High levels of PEEP also have been shown to augment the physiologic dead space,24,41,42 and worsen gas exchange35 and tissue perfusion.43–45 Potential extrapulmonary side effects of PEEP include decreased cardiac output,46–51 increased intracranial pressure,52,53 renal dysfunction,54,55 and decreased splanchnic perfusion56–58 and oxygenation.43,44 Although the benefits and detriments of PEEP in patients with ARDS have been variously weighted for many years, debate as to optimal PEEP and the best method for detecting it is still ongoing.
In the presence of airflow obstruction, the lungs may fail to deflate to FRC at end-expiration and alveolar pressure remain positive to an extent that depends on the volume of trapped air, a phenomenon referred to as auto or intrinsic PEEP (PEEPi).59 In this setting, application of external PEEP may be beneficial. Benefit is evident during spontaneous breathing: CPAP reduces dyspnea9,60 and work of breathing.9,60 Benefit is also evident with any patient-triggered mode of ventilation:2,61–63 PEEP enhances triggering function,2,61–63 reduces patient’s respiratory drive,62 reduces inspiratory muscle effort,2,61–63 and improves patient–ventilator interaction.63,64 PEEP replaces the amount of pressure that must be generated by the inspiratory muscles to offset PEEPi (necessary to initiate inspiratory flow or trigger the ventilator).2,62 Figure 10-1B summarizes the principal effects of PEEP in patients with chronic obstructive pulmonary disease (COPD).
PEEP has substantial effects on hemodynamics. When left-ventricular function is normal, an increase in intrathoracic pressure causes a fall in cardiac output, largely secondary to decrease in venous return (i.e., preload).47,65,66 In patients with poor left-ventricular function, when filling pressure and left-ventricular diastolic volume are elevated, cardiac output is relatively insensitive to decline in venous return.67 In this condition, the dominant effect of an increase in intrathoracic pressure is to decrease left-ventricular transmural pressure (i.e., afterload), which improves left-ventricular performance.68–71 These effects (Fig. 10-2C) are also observed with relatively moderate levels of CPAP; the impact on afterload is likely to result primarily from a reduction in the inspiratory negative swings of intrathoracic pressure,72 consequent to improvement in respiratory mechanics.72 Application of CPAP in patients with severe cardiogenic pulmonary edema may accelerate the physiologic improvement and reduce the need for endotracheal intubation.10,73–75
Figure 10-2
Heart–lung interactions during spontaneous breathing in a normal subject (A), and in a patient with cardiogenic pulmonary edema resulting from a low cardiac output in the absence (B) and presence (C) of CPAP. The effects of variations in intrathoracic pressure (ITP) on left-ventricular systolic function are shown at end-expiration (left panels) and end-inspiration (right panels). LVTM, left-ventricular transmural pressure (SAP − ITP); SAP, systolic arterial (intraventricular) pressure. In a normal subject (A), tidal excursions in ITP have little effect on left-ventricular systolic function. With pulmonary edema (B), more negative swings in ITP are needed to achieve the same tidal volume. As a result, LVTM (afterload) is increased independently of systemic vascular resistance. The rise in ITP produced by CPAP 10 cm H2O (C) produces a slight reduction in LVTM during expiration. CPAP remarkably decreases LVTM during inspiration by decreasing negative swings in ITP, because of improved lung mechanics.
Several investigators have studied the use of CPAP in the treatment of the obstructive sleep apnea syndrome, which is characterized by reduction or cessation of breathing secondary to periodic narrowing and/or collapse of the upper airway. Detailed discussion of this topic is beyond the scope of this chapter. Likewise, we do not provide detailed discussion of positive expiratory pressure, a physiotherapeutic technique aimed at clearance of secretions.
Physiologic Rationale for Positive End-Expiratory Pressure
The role of PEEP in correcting hypoxemia in patients with pulmonary edema and ARF has long been recognized11,12 and extensively documented.24,42,76–79 The chief mechanism by which PEEP improves arterial oxygenation is through an increase in the amount of alveoli reached by the air. By decreasing cardiac output, however, PEEP may negatively affect mixed venous O2 tension, which, in turn, decreases the partial pressure of arterial oxygen (PaO2) to an extent that depends on alveolar ventilation–perfusion () mismatch.80–83
Arterial oxygenation is often impaired during anesthesia84,85 as a result of the intrapulmonary shunt86–90 caused by atelectasis, predominantly in the dependent zones.85,91–97 Areas of collapsed parenchyma occur in 90% of all intubated anesthetized subjects, both during spontaneous breathing and muscle paralysis.90,98–100 Other mechanisms, such as regional distribution of ventilation,101 airway closure,102–112 and pharmacologic inhibition of hypoxic vasoconstriction113–116 may also contribute. Application of PEEP during anesthesia can prevent or reverse closing of peripheral airways,105 decrease atelectasis,90,100,117,118 and improve regional ratios and oxygenation,118–120 although shunt may only be partially improved.90 In normal anesthetized dogs, however, Dueck et al found that PEEP ≥10 cm H2O produced a reduction in PaO2 and increases in dead space ventilation and partial pressure of arterial carbon dioxide (PaCO2). This was mainly caused by a drop in cardiac output associated with an increase in alveolar ventilation of both high
and unperfused lung regions, suggesting overdistension of nondependent lung regions.121 Similarly, Pelosi et al showed that PEEP values up to 10 cm H2O decreased, although not significantly, PaO2 during general anesthesia in normal subjects.122
Alterations in diaphragmatic position and mechanics during anesthesia also contribute to impaired gas exchange by inducing ventilation abnormalities in the dependent and caudal zones.123,124 This phenomenon has been elegantly studied in anesthetized rabbits by Heneghan et al who compared PEEP and phrenic nerve stimulation, adjusted to produce the same increase in lung volume. Phrenic stimulation caused a greater caudal movement of the diaphragm, particularly in the dependent regions, and produced greater improvement in gas exchange than did PEEP.123
Beneficial effects of PEEP on oxygenation have been reported in obese patients, who are at greater risk to develop caudal atelectasis during anesthesia, and during pneumoperitoneum induced for laparoscopic surgery causing cephalad displacement of the diaphragm.122,125,126 Pelosi et al found that PEEP induced an increase in PaO2 in obese patients; this increase was correlated with recruitment of atelectatic lung regions.122 Other authors found that the sole application of 10 cm H2O PEEP was able to reduce atelectasis but not to improve oxygenation in these patients.125,127,128 Oxygenation improved and atelectasis was further decreased, however, when PEEP application was preceded by a recruitment maneuver or associated with vertical positioning both in obese patients and healthy subjects undergoing laparoscopic surgery. In the former case, use of high airway pressures opened atelectatic lung areas subsequently kept open by PEEP, while in the latter case vertical positioning decreased the negative effect of abdominal pressure on diaphragmatic displacement. In morbidly obese patients, PEEP has also been found beneficial during induction of general anesthesia. Gander et al showed that applying 10 cm H2O PEEP during induction of anesthesia increased the duration of apnea without hypoxia by approximately 50%.129
Conflicting data on the effect of PEEP have been obtained in postoperative patients who had developed respiratory failure. In two studies, PEEP up to 10 cm H2O did not significantly modify PaO2 during the early postoperative course of patients who underwent cardiac or vascular surgery.130,131 Conversely, other investigators found that PEEP, combined with bilevel positive airway pressure ventilation administered via a nasal mask, improved oxygenation in the vast majority of patients who exhibited respiratory distress after various types of surgery.132–135 A recent randomized, clinical trial found that the use of noninvasive positive-pressure ventilation with low PEEP (approximately 4 cm H2O) increased PaO2, reduced the need for intubation, and improved survival of patients who developed ARF after lung resection.136
In summary, use of PEEP to improve oxygenation during and after anesthesia in normal subjects has generated contradictory results, probably because of the deleterious effects of PEEP on hemodynamics and lung overdistension. PEEP, however, can be effective in improving gas exchange in selected patients.
Most forms of hypoxemic respiratory failure, such as cardiogenic pulmonary edema, ARDS, and unilateral pneumonia, are characterized by a decrease in lung volume caused by atelectasis, interstitial and alveolar edema, and small airway closure. The pivotal mechanism for hypoxemia in these conditions is intrapulmonary shunt as demonstrated by the small increase in PaO2 when pure O2 is administered,137 and, to a lesser extent, mismatch.138,139 Moreover, the high fractional inspired oxygen concentration (FIO2) frequently used in these patients, may decrease lung volume by promoting alveolar denitrogenation and reabsorption atelectasis.137,140,141 In ARDS, the altered blood flow distribution, resulting from widespread involvement of the pulmonary vasculature142 and impaired hypoxic vasoconstriction, contributes to worsening of gas exchange.140
PEEP has long been recognized as an effective means of increasing lung volume and improving gas exchange.1 In cardiogenic pulmonary edema, CPAP (alone or in association with an inspiratory assistance) improves gas exchange10,73,143–154 by increasing aerated lung volume,155 and improving cardiac output73,143,148 and distribution.155,156 A decrease in extravascular lung water is another possible mechanism whereby PEEP reduces intrapulmonary shunt.157 Yet, PEEP does not decrease, and sometimes increases, extravascular lung water when microvascular hydrostatic pressure is high.158 Malo et al156 clarified this issue. They showed that PEEP 13 cm H2O decreased shunt and alveolar flooding without modifying extravascular lung water; indeed, perivascular cuff edema increased.156 These findings indicate that PEEP redistributes the excess alveolar water into the compliant perivascular space, thus reinflating previously flooded and collapsed air spaces, without decreasing the overall amount of lung edema.159
Unilateral pneumonia and ARDS are characterized by severe impairment of gas exchange because of increased intrapulmonary shunt,138,140 resulting mainly from flooded and/or collapsed alveoli.160–163 This observation is corroborated by the correlation between the extent of lung densities mainly found in the dependent regions on a computed tomography (CT) scan and deterioration in arterial blood-gases.18,78 In fact, the edema increases the total mass of the lung (up to more than twice that of normal lungs); consequently, the dependent zones progressively collapse under the weight of the superimposed lung (compression atelectasis), and aerated lung decreases (“baby lung” concept).164–166
Atelectasis in the lower lobes and in dependent lung regions may be caused by other mechanisms, such as external compression by the heart167,168 and the abdominal compartment.93,169–172 The severity of intrapulmonary shunt may also be affected by surfactant abnormalities,173 increased airway resistance,174–187 hyaline membranes, and inflammatory proteins and cells accumulating within the alveoli. Interstitial edema and the deposition of cells and connective tissue in the interalveolar septa may further contribute to gas exchange impairment in ARDS. The amount of nonaerated lung volume may also be increased by the combined effects of reabsorption atelectasis89,138,140,188 and ventilation occurring at low lung volumes.141,189,190 Hypoxic pulmonary vasoconstriction appears greatly impaired in most patients with ARDS.140,191 In some patients with diffuse lung injury, however, hypoxic pulmonary vasoconstriction is partially preserved, explaining a lack of hypoxemia despite diffuse loss of aeration. Conversely, hypoxic pulmonary vasoconstriction is constantly impaired in some patients with localized lung injury, explaining severe hypoxemia despite well-preserved lung aeration.191
Use of PEEP to correct gas exchange impairment in ARDS was initially proposed by Asbaugh et al.1 It remains the cornerstone of ventilatory management of these patients. Extensive literature supports the use of PEEP for improving oxygenation in hypoxemic respiratory failure, alone or combined with various ventilator modes, during both invasive5,18,42,76,78,184,192–209 and noninvasive ventilation.210–213 Several mechanisms may explain the effect of PEEP on gas exchange. PEEP promotes alveolar recruitment and increases aerated lung volume, therefore decreasing intrapulmonary shunt.214,215 PEEP-induced recruitment of aerated lung volume is strongly correlated with arterial oxygenation (Table 10-1).18,197,200,215,216 Prevention of atelectasis consequent to pure O2 breathing helps explain the beneficial effect of PEEP on oxygenation.141 In addition, a redistribution of alveolar edema to the interstitial spaces may also explain the benefit of PEEP on gas exchange.156 Following the pioneering work of Webb and Tierney,217 PEEP has been shown to decrease pulmonary edema,218–220 partly because of a concomitant reduction in cardiac output.219 By recruiting nonaerated alveoli and stabilizing airways, PEEP also affects the regional distribution of tidal ventilation.169,199,221,222 When the predominant effect of PEEP is recruitment, alveolar ventilation is expected to become more homogeneous, particularly in the dependent zones. Although an increase in lung volume is the main mechanism for PEEP-induced changes in oxygenation, a small decrease in cardiac output also reduces intrapulmonary shunt and improves PaO2.48 Finally, different pathophysiologic mechanisms of ARDS influences the response to PEEP,31,33,198 although understanding is far from being conclusive.223
Reference | Technique | PEEP cm H2O | FRC mL | ΔEELV mL | Vrec mL | Vrec/ΔEELV | Voverdist ml | ΔPaCO2 mm Hg |
---|---|---|---|---|---|---|---|---|
Ranieri 1991 (200) | P-V curve | 15 | – | 720 | 230 | 0.32 | – | 50 |
Ranieri 1994 (201) | P-V curve | 15 | – | 690 | 248 | 0.36 | – | – |
Ranieri 1995 (202) | P-V curve | 10 | – | 1274 | 756 | 0.59 | – | 55 |
Jonson 1999 (25) | P-V curve | 10 | – | – | 205 | – | – | – |
Richard 2001 (204) | P-V curve | 11 | – | – | 175 | – | – | – |
Maggiore 2001 (197) | P-V curve | 15 | – | 764 | 304 | 0.40 | – | 79* |
Mergoni 2001 (315) | P-V curve | 15 | – | – | 379 | – | – | 29 |
Koutsoukou 2002 (184) | P-V curve | 15 | – | 660 | 457 | 0.69 | – | 57 |
Richard 2003 (203) | P-V curve | 14 | – | – | 384 | – | – | 20† |
Maggiore 2003 (296) | P-V curve | 15 | – | 650 | 235 | 0.36 | – | – |
Vieillard-Baron 2003 (316) | P-V curve | 12 | – | 358 | 69 | 0.19 | – | 44 |
Thille 2007 (345) | P-V curve | 11 | – | – | 226 | – | – | – |
Valta 1993 (208) | Static compliance | 14 | – | 500 | 126 | 0.25 | – | – |
Gattinoni 1998 (282) | Static compliance | 15 | 576 | 721 | 131 | 0.18 | – | – |
Chelucci 2000 (314) | Static compliance | 13 | – | 446 | 102 | 0.23 | – | – |
Vieira 1998 (36) | CT scan | 13 | – | – | 320 | – | 238 | 117 |
Vieira 1999 (225) | CT scan | 16 | – | – | 332 | – | 13 | 113 |
Puybasset 2000 (199) | CT scan | 10 | 1621 | 659 | 187 | 0.28 | 41 | 45 |
Malbouisson 2001 (18) | CT scan | 15 | 1553 | 820 | 499 | 0.61 | 24 | 99 |
Nieszkowska 2004 (311) | CT scan | 15 | 1105 | 1115 | 369 | 0.33 | 63 | 80 |
MEAN | 13 | 1214 | 721 | 287 | 0.37 | 76 | 66 | |
SD | 2 | 483 | 249 | 161 | 0.16 | 93 | 32 |
Improved efficiency of alveolar ventilation and an expected decrease in alveolar dead space should produce a reduction in PaCO2. Several authors, however, have failed to find any significant relationship between PaCO2 and PEEP. The lack of response probably arises because PEEP both favors lung recruitment, which theoretically reduces PaCO2, and promotes pulmonary overdistension, which theoretically increases PaCO2.33,169,224
The benefit of PEEP on gas exchange might also be expected in unilateral pneumonia and localized lung injuries. In these conditions, however, high PEEP is more likely to produce overdistension of normally aerated regions, because of the coexistence of areas with normal, low, and very low compliances.169,199 The net effect of PEEP on gas exchange depends on the balance between overdistension of already aerated alveolar units and recruitment of collapsed (nonaerated) alveoli, and is also influenced by the level of PEEP.169,225 In patients with unilateral lung disease, PEEP may be detrimental whenever it hyperinflates the normal lung, thus directing the blood flow to the diseased lung and increasing intrapulmonary shunt.226–230 To limit this risk, unilateral delivery of PEEP to the injured lung has been proposed;231 the technical complexity of this approach greatly limits its feasibility in the clinical practice.
In summary, PEEP improves gas exchange in hypoxemic ARF, mainly through recruitment of previously nonaerated lung areas and the homogenization of regional distribution of tidal ventilation. PEEP remains the cornerstone of ventilator treatment of most forms of hypoxemic ARF. Debate continues about the optimal level of PEEP, not about its usefulness.
Impaired gas exchange in obstructive lung diseases is caused by complex disturbances of regional relationships associated with alveolar hypoventilation; diffusion impairment is not a major factor.232 During an acute exacerbation of COPD, high
areas predominate in patients who mostly have the emphysematous variant with loss of blood flow consequent to alveolar wall destruction.232–234 Conversely, low
regions are mostly seen in patients with a predominant obstructive component.232–234 In general, application of PEEP does not affect gas exchange.2,235–238 Nevertheless, Rossi and coworkers reported that low PEEP (50% of PEEPi) induced a moderate increase in PaO2 and slight decrease in PaCO2 secondary to improved
distribution without alteration in respiratory mechanics or hemodynamics.239 Further increases in PEEP (up to 100% of PEEPi) did not produce further improvement in gas exchange.239 In other small studies, low to moderate PEEP (5 to 9 cm H2O) produced similar improvements in PaCO2 and PaO2.240–243 Other authors, however, reported little or no change in arterial oxygenation with PEEP2,60,237,238 unless high levels were applied;237,238 PaCO2 did not change with up to 15 cm H2O PEEP.2,60,236–238
PEEP may have some benefit on gas exchange. Unless hypoxemia is caused by a large intrapulmonary shunt, the benefit is small and of little clinical importance and PEEP may have negative hemodynamic effects that worsen oxygen delivery.236–238 The balance between positive and negative effects depends to some extent on the level of applied PEEP; nevertheless, the chief reason for using PEEP in COPD is not to improve gas exchange.
The static behavior of the respiratory system is described by the pressure–volume curve.244–246 In healthy subjects, the pressure–volume curve, from residual volume to total lung capacity, has a sigmoidal shape.246 Above FRC, however, the curve is linear. Thus, the tangential slope of the curve (i.e., the linear or chord compliance, reflecting the elasticity of the respiratory system) is constant over the range of tidal ventilation. FRC is the volume of gas in the lungs at the end of a passive expiration. It corresponds to the point of equilibrium between lung and chest-wall elastic recoil.
The shape of the pressure–volume curve reveals two important features: stiffening of the lungs at high lung volumes, and closure of peripheral airways and lung units at low lung volumes.247 At volumes above 75% to 80% of total lung capacity, the slope of the pressure–volume curve varies, with a sharp decrease in compliance, indicating that the lungs are close to their maximum stretch limit, although the chest wall is normally not stiffer. Further increases in pressure beyond this point, the so-called upper inflection point (UIP), have progressively less effect on lung volume. When lung volume falls below FRC and approaches residual volume, the smallest airways tend to collapse under the influence of surface tension of alveolar lining fluid.248–251 During the subsequent inflation, these structures remain closed until a much higher pressure is applied. From this point on, compliance rapidly increases during inflation, as the closed lung units progressively pop open. This action produces a knee on the pressure–volume curve, termed the lower inflection point (LIP).
During constant-flow controlled ventilation, the elastic and resistive properties of the respiratory system can be assessed using the occlusion technique, which consists of occluding the airway at end-inspiration for 3 to 5 seconds.252–258 As soon as flow is interrupted, there is a rapid drop in airway pressure from the peak (PPEAK) to a lower value (P1), followed by a gradual decay to an apparent pressure plateau (PPLAT) (Fig. 10-3). Ohmic (flow-dependent) resistance (Rmax) is calculated as the initial drop in airway pressure (PPEAK − P1) divided by inspiratory flow. The slower pressure change (P1 − PPLAT) divided by the inspiratory flow preceding the occlusion yields the additional tissue resistance (ΔR). The sum of airflow and tissue resistances provides the total respiratory resistance (RTOT = (PPEAK – PPLAT)/). By occluding the airway at end-expiration, it is possible to determine the total PEEP (PEEPTOT), which is the sum of the externally applied PEEP and PEEPi (Fig. 10-4). The difference between PPLAT and PEEPTOT is the recoil pressure. It is then possible to compute the static compliance (Cst) of the respiratory system according to the equation Cst = VT/(PPLAT − PEEPTOT). The failure to take into account PEEPi may result in a substantial underestimation of Cst, especially when low or no PEEP is used.259,260
Figure 10-4
End-expiratory occlusion. Airway pressure rises following an end-expiratory occlusion and reaches a plateau, which corresponds to total PEEP (PEEPTOT). Auto or intrinsic PEEP (PEEPi) is the difference between PEEPTOT and the externally applied preset PEEP (PEEPe). PEEPi measured by an end-expiratory occlusion is termed static.
Anesthetized healthy subjects may have decreased aerated lung volume, consequent to the decline in FRC associated with the supine posture93,127,128,261,262 and the potential for airway closure and atelectasis.85,96,100,127,128,190 Both anesthesia and paralysis affect the mechanical characteristics of the respiratory system,85,263,264 which can be also impaired by abdominal and thoracic surgical procedures.125,128,265–267 It is thought that anesthesia primarily alters the elastic properties of the chest wall, causing a fall in FRC, whereas the changes in lung compliance may result from breathing at low lung volumes.128,263,268,269 Application of PEEP in anesthetized and paralyzed subjects produces an increase in end-expiratory lung volume125,128,270–272 and upward displacement of respiratory system, lung and chest wall pressure–volume relationships (Fig. 10-5A).272–274 Several authors, however, report little or no increase in static respiratory compliance with PEEP.272,275,276 In some studies, PEEP increased both chest wall and lung compliance.272 In other studies, chest wall compliance increased and lung compliance decreased,276 or no effect was observed.275
Figure 10-5
Pressure–volume (P-V) curves recorded from different PEEP levels in a healthy subject (A), a patient with ARDS (B), and a patient with an acute exacerbation of COPD (C). All curves are related to the relaxation volume of the respiratory system. For each curve, end-expiratory and end-inspiratory points are indicated by circles. In the healthy subject, PEEP 10 cm H2O generates an increase in end-expiratory lung volume and a rightward shift of the P-V curve. The new curve is superimposed on the curve acquired without PEEP, indicating the absence of recruitment. In the patient with ARDS, increasing PEEP produces increases in end-expiratory lung volume and significant lung recruitment, as indicated by the upward shift of the P-V curves. In the patient with COPD, the application of PEEP results in end-expiratory lung volume increase with worsening of hyperinflation, as indicated by the superposition of P-V curves and the progressive decrease in the slope (compliance) of the curves. The reduction in aerated volume in ARDS produces a lower inflection point and flattening of the P-V curve at PEEP 0 cm H2O. The presence of PEEPi during ventilation without PEEP in ARDS, and more so in COPD, induces a rightward shift of the initial P-V curve. In the healthy subject (left panel), the PEEP-induced increase in end-expiratory lung volume is indicated by the dotted line.Vr, Relaxation volume of the respiratory system.
The effect of PEEP on the elastic properties of the respiratory system varies with the amount of applied PEEP273 and preexisting derangement in respiratory mechanics. Compared with zero end-expiratory pressure (ZEEP), D’Angelo et al273 found that PEEP of 9 cm H2O increased both respiratory system and lung static compliance; the compliances, however, decreased when PEEP was raised above 20 cm H2O, indicating lung overdistension. Dechman et al277 also observed an increase in lung compliance at PEEP 10 cm H2O in patients undergoing closed-chest surgery, but not in patients undergoing open-chest surgery, who exhibited a progressive decrease in dynamic lung compliance. The mechanical characteristics of the chest wall may influence the effect of PEEP in subjects with normal lungs during anesthesia.263 In patients undergoing abdominal surgery, Pelosi et al122 reported that 10 cm H2O PEEP did not improve respiratory function in normal subjects, although it increased end-expiratory lung volume and lung and chest wall compliance in morbidly obese patients. Others reported similar improvements in end-expiratory lung volume and/or in respiratory system compliance both in obese patients125,127,128 and in healthy subjects with impaired chest wall compliance caused by pneumoperitoneum during laparoscopic surgery.125,267
In general, PEEP decreases airway resistance.122,267,272,273 The decrease in airway resistance is generally small and mainly related to increase in lung volume,272 although other mechanisms, such as a PEEP-induced modification of basal vagal tone,278 might also play a role.
Since the first description by Asbaugh et al,1 ARDS has been recognized as a condition characterized by reduction in aerated lung and respiratory system mechanical derangements.76,79,279,280 In ARDS, massive lung edema, atelectasis, and tissue consolidation cause a marked decrease in FRC.76,79 This fall in normally ventilated lung volume is the main cause of the impaired respiratory mechanics.165 Conversely, an increase in aerated lung volume is long recognized as the main cause of the benefit of PEEP on lung function.42,76 The increase in lung volume may result from two different mechanisms: recruitment of terminal lung units not accessible to ventilation because of collapse or flooding, and distension or overdistension of already open lung units.
Several authors reported an increase in end-expiratory lung volume and FRC following the application of PEEP in patients with ARDS (see Fig. 10-5B).14,18,36,42,76,199,214,281,282 In absence of PEEPi, the increase in lung volume above FRC at end-expiration, or change in end-expiratory lung volume (ΔEELV), depends on the amount of applied PEEP and the compliance of the respiratory system. Different techniques can be used to measure FRC and EELV, such as helium dilution,283–288 nitrogen285,288–290 and sulphur hexafluoride288,291–294 washout, positron emission tomography,295 and CT scan.36,78,199 These measurements are not routinely obtained in the clinical setting because of technical limitations and/or logistic complexities.
A surrogate approach has been used for assessing PEEP-induced ΔEELV; this involves measuring the volume exhaled between PEEP and elastic equilibrium volume of the respiratory system (Fig. 10-6).25,197,200–204,266,296,297 While the ventilator is delivering VT, the preset PEEP is rapidly brought to zero. During the following expiration, sufficient time (5 to 10 seconds) is provided to enable complete exhalation; thus the elastic equilibrium, relaxation volume, will be reached.25,176,197,200,202 The difference between the volume exhaled during this prolonged expiration and the volume insufflated during the preceding inspiration represents the ΔEELV produced by PEEPTOT. The excess volume caused by PEEPi should be separately assessed, and accounted for, to avoid overestimation of PEEP-induced ΔEELV. PEEP-induced variations in EELV can also be measured by inductive208,296,298–301 or optoelectronic plethysmography.19,302
Figure 10-6
Measurement of PEEP-induced change in end-expiratory lung volume (ΔEELV). PEEP 10 cm H2O is abruptly brought to zero. A prolonged expiration ensues, and relaxation volume is achieved. The difference between the volume exhaled during this expiration and the tidal volume delivered by the ventilator equals the increase in EELV induced by PEEP.
An increase in EELV may also occur when PEEP distends or overdistends already aerated lung regions (Fig. 10-7 and see Table 10-1). Hence, measurement of ΔEELV, without quantification of lung recruitment, may be misleading. Lung recruitment refers to re-aeration of collapsed or fluid-filled terminal airways and alveoli. These areas become accessible to ventilation (anatomic recruitment) and may participate in gas exchange if local perfusion is preserved (functional recruitment). Lung recruitment can be quantified by using pressure–volume curves, traced from different levels of PEEP, or by CT scan analysis (see Table 10-1). Other techniques have been proposed, but their application in clinical practice is at present very limited.295,303–306
Figure 10-7
Pressure-volume (P-V) curves at different levels of PEEP (0, 5, 10, and 15 cm H2O), related to relaxation volume (Vr) of the respiratory system, in two patients with ARDS. In the left panel (A), PEEP induces progressive upward shifts of P-V curves, indicating recruitment. At PEEP 15 cm H2O, PEEP-induced increase in end-expiratory lung volume above Vr is indicated by the dotted line between the two open circles. Alveolar recruitment induced by PEEP 15 cm H2O, compared to PEEP 0 cm H2O, and measured at a pressure of 15 cm H2O is shown by the double-arrow solid line. In the right panel (B), P-V curves are particularly flattened, suggesting a marked decrease in aerated lung volume. Increasing PEEP results in minimal recruitment: P-V curves are almost totally superimposed and progressively shifted to right. In both panels, all P-V curves tend to converge at high lung volumes, suggesting that total lung capacity is approached. PEEP-induced recruitment is greater when the slope of the linear segment above the lower inflection point (LIP)—that is, linear compliance—of curve recorded from 0 cm H2O (the lowest curve) is high (A). When the curve at 0 cm H2O has a very low linear compliance, PEEP-induced recruitment is trivial (B). PEEP-induced recruitment also proceeds far above LIP (up to pressures greater than 40 cm H2O), suggesting that lung reopening is a pan-inspiratory phenomenon (A).
Measurement of PEEP-induced lung recruitment using pressure–volume curve stems from the pioneering observations of Katz et al14 on the time course of PEEP-mediated increase in lung volume. After applying PEEP, these authors observed that end-expiratory lung volume increased to approximately 66% of its total change within the first breath; it reached 90% in approximately five breaths, and was fully complete only after several minutes, suggesting time-dependence for lung recruitment.14 For a given pressure, lung volume measured during ventilation with 13 cm H2O PEEP was larger than the volume predicted for that pressure by the static compliance computed at 3 cm H2O PEEP. This observation suggests that some lung units were not ventilated at the lower PEEP, but were recruited by the higher PEEP.14 Quantification of lung recruitment induced by PEEP was not attempted until Ranieri et al200–202 proposed a simple method using pressure–volume curve analysis. This approach consists of aligning on the same pressure–volume diagram curves obtained at ZEEP and varying PEEP levels. With this technique, which assumes that FRC (i.e., the relaxation volume at ZEEP) remains constant,14,200,201 the ΔEELV induced by PEEP is added to the volume insufflated during the maneuver for recording pressure–volume curve at the corresponding PEEP level.197,247,297 Lung recruitment is then computed as the difference in volume between pressure–volume curves obtained at different PEEP levels at a given value of elastic recoil pressure (generally 20 cm H2O). Anatomic recruitment is reflected by an upward shift of the pressure–volume curve (see Fig. 10-7). If PEEP fails to recruit new regions, no volume gain at a given pressure will be observed; the resulting pressure–volume curve will be shifted rightward and superposed on the curve acquired at ZEEP.197,201
The CT scan is used to measure recruitment.215,307,308 PEEP-induced lung recruitment is computed as the decrease in volume of nonaerated lung parenchyma78,164,216,221,307 or, as recently proposed, the increase in volume of gas penetrating nonaerated and poorly aerated lung regions.18,308–311 With this technique, however, recruitment is assessed by comparing scans taken at different pressures (ZEEP and PEEP), and not at the same pressure as is done with the pressure–volume curve technique. Consequently, no matter how it is computed, recruitment at a given PEEP will include also a certain volume of gas corresponding to lung units that inflate normally from end-expiration at ZEEP up to the pressure corresponding to that PEEP. This helps explain some of the differences in the amounts of recruitment with the CT and pressure–volume techniques.312 Conversely, the CT scan offers a unique opportunity to estimate the amount of PEEP-induced hyperinflation (see Table 10-1),18,36,78,199,225,311,313 computed as the increase in gas volume within normally aerated lung regions; hyperinflation, however, does not necessarily mean overstretching.307
Bedside lung ultrasound has been proposed as a mean to estimate PEEP-induced recruitment. In forty patients with ARDS, Bouhemad et al compared the pressure–volume curve method and lung ultrasound to assess recruitment with PEEP 15 cm H2O.303 Significant correlations were found between an ultrasound re-aeration score and recruitment measured by pressure–volume curve, and between ultrasound score and oxygenation. This new technique did not, however, allow assessment of lung hyperinflation. Further studies are needed to evaluate the usefulness of this technique.
In patients with ARDS, PEEP increases end-expiratory lung volume and produces varying recruitment (see Table 10-1).18,25,76,78,169,197,199–204,208,216,221,225,275,282,296,311,313–317 Recent work197 shows that PEEP achieves progressive recruitment, from 84 mL at PEEP 5 cm H2O (range: 41 to 156 mL) to 304 mL at 15 cm H2O (range: 114 to 545 mL). The large interindividual differences suggest that, in certain patients, PEEP increases lung volume without achieving significant recruitment, likely reflecting overdistension of previously aerated lung regions. Indeed, PEEP-induced overdistension has been shown in several studies.200–202 18,36,199,225,311 In most cases, recruitment and overdistension occur concomitantly, rather than sequentially, in different regions of the lung following PEEP application.199,225,308,309,311,313 Vieira et al36 found that applying PEEP 10 and 15 cm H2O in patients with ARDS who did not have a clear LIP on the pressure–volume curve produced progressive recruitment of nonaerated lung areas and overdistension of aerated lung zones. The interplay between these two phenomena depends on several factors, such as lung and chest wall mechanics,266,318 stage of disease,319,320 lung morphology,199,225,309 and amount of PEEP.225
Recent studies highlight the importance of assessing lung recruitability for an individually tailored optimization of PEEP. By using CT scan in sixty-eight patients with ARDS, Gattinoni et al. assessed the percentage of potentially recruitable lung, as defined by the proportion of lung tissue in which re-aeration was restored at airway pressure between 5 and 45 cm H2O, and the effects of PEEP.33 Lung recruitability was 13% ± 11% of the lung weight, varied widely across the studied population (from 0% to more than 50%), and was highly correlated with lung recruitment induced by an increase in PEEP from 5 to 15 cm H2O. Patients with higher lung recruitability had more severe disease (lower oxygenation and respiratory system compliance) and a higher mortality rate.
Assessment of lung recruitability is important for setting PEEP: the use of high PEEP in patients with a low percentage of potentially recruitable lung provides little, if any, benefit and may actually be harmful if it produces overdistension.321 Lung recruitability may influence the effects of PEEP on alveolar strain (the ratio between the amount of gas delivered during a tidal breath and the amount of aerated lung available for ventilation) and alveolar opening and closing.
Caironi et al reported that an increase in PEEP from 5 to 15 cm H2O in patients with a lower percentage of potentially recruitable lung did not significantly affect the amount of opening and closing lung tissue, which was already negligible at lower PEEP.27 Conversely, in patients with a higher recruitability, opening and closing was dramatically reduced (approximately equal to 50%) by increase in PEEP. Interestingly, increases in PEEP led to an identical increase in alveolar strain in the two groups of patients. In patients with higher recruitability, the lung volume available for ventilation conceivably increases during inspiration as recruitment of nonaerated lung regions at end-expiration progressively occurs. These findings further suggest a relationship between the amount of intratidal lung recruitment and the effective end-inspiratory alveolar strain, such that the larger the amount of potentially recruitable lung, the lower the risk of PEEP-induced overdistension. In addition, opening and closing lung tissue turned out to be an independent risk factor for death (odds ratio 1.10 for each 10 g increase), while alveolar strain did not.27 This suggests that in patients with higher lung recruitability, the beneficial impact of reducing intratidal opening and closing by increasing PEEP prevails over the effects of increasing alveolar strain. In this regard, lung imaging morphology (chest radiograph or CT scan) may help in assessing lung recruitability: patients with a lower percentage of potentially recruitable lung generally show a “lobar” pattern of lung consolidation, whereas patients with greater lung recruitability often present diffuse lung collapse.27,199,225,321
The integrated analysis of respiratory mechanics, lung volumes, and lung imaging enhances recognition of the effects of PEEP on recruitment and overdistension, and hence facilitates to set optimal PEEP at the bedside.
Effect of Positive End-Expiratory Pressure on Respiratory Mechanics in Acute Respiratory Distress Syndrome
Contrasted with normal subjects, the rate of change per unit of pressure is smaller, and linear compliance of the pressure–volume curve falls to very low values in patients with ARDS (because of the decrease in normally ventilated alveolar units); the whole curve is also flattened and shifted downward, and the inflection knees, normally seen at low (LIP) and high (UIP) lung volumes, may appear in the range of tidal ventilation247 (see Figs. 10-5B and 10-7). The presence of LIP and UIP in the VT range suggest considerable susceptibility of the ARDS lung to detrimental mechanical shear forces, generated by cyclic end-expiratory alveolar opening and collapse78,165,322 and end-inspiratory overstretching.201,323 These forces are considered the main mechanism of ventilator-induced lung injury.324,325 Recent evidence suggests that airspace reopening can occur along the entire pressure–volume curve.197,216,307,326–329 Moreover, the shape and characteristics of the pressure–volume curve can be greatly influenced by several factors including pathophysiologic mechanisms, stage of disease,282,322 chest wall mechanics,198,266 breathing pattern, and differences in measurement technique.330
As already mentioned, one typical feature of ARDS is the fall in respiratory system compliance (see Figs. 10-5B and 10-7), described both in experimental331–334 and clinical settings.42,76,176,322,335 Decreased compliance was initially attributed to increased lung stiffness.280 Subsequent studies have demonstrated that the decreased compliance in ARDS has different pathophysiologic mechanisms.333,334 In an animal model, Grossman et al333 showed that the loss of aerated lung units, secondary to alveolar flooding, accounted for virtually all of the decreased static lung compliance. With CT scan analysis, Gattinoni et al165 found that several parameters of the pressure–volume curve, including linear compliance, were correlated with the amount of aerated areas (mainly distributed in the nondependent, ventral lung regions) but not with nonaerated areas (preferentially distributed in the dependent, dorsal lung regions). Indeed, compliance was in the normal range when normalized to FRC (so-called specific compliance), suggesting that the aerated areas had normal intrinsic elasticity.165 According to this concept, respiratory system compliance provides an indirect estimate of the amount of aerated lung (see Figs. 10-5B and 10-7).
Through recruitment, PEEP prevents the fall in static compliance,5 and restores the normal pressure–volume pattern, by suppressing small airway closure and collapse of unstable lung units.281,336–339 Data suggest that the effects of PEEP on recruitment and overdistension can be predicted by the shape of the pressure–volume curve at ZEEP.197,200,201,225,326,328,340 Three distinct patterns of pressure–volume curve have been described in patients with ARDS.200,201,225 A concave shape, with a clear LIP, indicates that compliance is progressively increasing above this point, suggesting ongoing recruitment during inflation (see Fig. 10-7).200,201,340 A convex shape, showing an UIP, denotes progressive decrease in compliance, and likely overstretching (see Fig. 10-7).200,201 A linear pressure–volume curve, with absent or very low LIP, may be observed in localized lung injury.225
Rouby et al191,309,310 hypothesized that a linear pressure–volume curve results from two separate regional curves: a concave curve, related to normally aerated lung regions, and a convex curve, related to nonaerated areas. PEEP may have different effects on lung volumes and respiratory mechanics according to these patterns. When a concave shape is observed at ZEEP, PEEP results in lung recruitment, as documented by an upward shift of the pressure–volume curve and disappearance of LIP (see Fig. 10-7).197,200,201,225 With a convex profile and low linear compliance at ZEEP, PEEP-induced recruitment is minimal or zero: the pressure–volume curve is superimposed on that obtained at ZEEP, with a further decrease in compliance (see Fig. 10-7).197,200,201,225
A linear compliance on the pressure–volume curve at ZEEP may be a useful indicator of lung recruitability (see Fig. 10-7). A tight correlation between linear compliance at ZEEP and amount of PEEP-induced recruitment has been suggested.197 Because compliance, as assessed on the pressure–volume curve, is a function of normally aerated lung regions,165 two different situations can be imagined: (a) the lung is principally characterized by unstable lung regions, which are collapsed at the end expiration but progressively pop open during tidal inflation, and (b) the lung is predominantly consolidated, with no large areas expanding during inflation (compliance is very low). Application of PEEP results in significant recruitment in the former case; in the latter case, PEEP may overstretch already ventilated areas without producing significant recruitment.200,201 Independently of its effect on recruitment and overdistension, PEEP constantly and significantly decreases the linear compliance of the pressure–volume curve.25,197,201,326,328 This contrasts with the classical notion that compliance increases with recruitment and decreases with overdistension.42 Lung units that pop open at a certain pressure have an infinitely high compliance and contribute to the higher compliance observed at ZEEP, as opposed to that observed when the lung is fully recruited at PEEP. A corollary is that UIP may indicate the end of tidal recruitment and/or the beginning of alveolar overdistension, although both mechanisms may coexist at high pressure (see Fig. 10-7).25,326 Therefore, PEEP-related decrease in pressure–volume linear compliance may predominantly reflect recruitment below UIP; a decrease in compliance above this point may reflect overdistension.
LIP has long been considered the pressure at which collapsed lung units open during inflation, it is therefore considered the ideal pressure at which to set PEEP.322 No relationship between LIP and either opening or closing alveolar pressures, however, was subsequently found,197 indicating that LIP is not useful for optimizing PEEP setting. Other studies confirmed that recruitment and derecruitment are continuous processes unrelated to LIP (see Fig. 10-7).25,204,215,216,326,328,329 Nevertheless, the presence of a clear LIP followed by a high inflation compliance on the pressure–volume curve recorded from ZEEP may predict the effect of PEEP on recruitment (see Fig. 10-7).165,197,225,328 Vieira et al225 showed that patients with a diffuse loss of aeration had a marked LIP at ZEEP, whereas LIP was absent or blunted in patients with a focal loss of aeration. High PEEP induced progressive recruitment without overdistension in the former group, whereas it caused a significant overdistension of normally aerated regions in the latter group.225 Some think that LIP is caused by time-constant inequalities within the lung and/or reopening of compressed peripheral airway associated with expiratory flow limitation.183,184,341,342 Accordingly, the disappearance of LIP following application of low or moderate PEEP might indicate that PEEP can prevent small airway closure and make the distribution of tidal ventilation more homogeneous.184,342 This could also explain the difference between the reported values of LIP, 5 to 15 cm H2O, and the substantially higher pressures required to reverse atelectasis.216,245,343
Some studies suggest that the point of maximum curvature of the deflation limb of the pressure–volume curve may be used to set PEEP so as to optimize lung recruitment, but the feasibility of this approach is limited.215,344
The mechanical properties of the chest wall may influence the effects of PEEP.198,266,282 Mergoni et al198 found that in some patients the LIP on the pressure–volume curve of the respiratory system at ZEEP reflected the chest wall, rather than the lung. Application of PEEP abolished LIP in all instances, but oxygenation significantly improved, suggesting recruitment, only in patients exhibiting a LIP on the lung pressure–volume curve.198 Gattinoni et al282 found that increasing levels of PEEP decreased respiratory and lung static compliance, without any recruitment, in patients with primary pulmonary ARDS; in patients with secondary extrapulmonary ARDS, PEEP induced significant recruitment and increased respiratory, lung, and chest wall compliance.282 These findings have been confuted by other studies, even by the same group.25,33,197,199,225,345
The change in static compliance produced by PEEP has been proposed as a guide for optimizing PEEP42 and as a prognostic factor for predicting mortality in patients with ARDS.346 Because of nonlinearity of the pressure–volume relationship in ARDS, however, and the aforementioned complex effect of recruitment on the pressure–volume relationship,326–328 static compliance (calculated as the pressure-to-volume differences between two points) may not describe adequately the changes in respiratory mechanics produced by PEEP.247,297 Indeed, as previously shown347 and recently outlined,182,327,345 static compliance may vary for different VTs. A mathematical model suggests that the highest static compliance computed at a low VT during a decremental, but not incremental, PEEP trial may help to identify the level of PEEP needed to prevent end-expiratory alveolar collapse.327 These theoretical findings are supported by data in animals,348,349 but have not been sufficiently tested in humans.
Some authors recommend the monitoring of dynamic compliance as a means of assessing the mechanical properties of the respiratory system under dynamic condition (ongoing ventilation) and to identify the level of PEEP that prevents alveolar derecruitment.350,351 Data on this issue, however, are far from conclusive.
Several studies179,182,185,314,352 reveal that PEEP increases additional resistance through increased viscoelastic dissipation180 that is consequent to a PEEP-mediated increase in end-expiratory lung volume, as suggested by the correlation between changes in tissue resistance and end-expiratory lung volume.352 Other studies,275,353 although confirming the rise in tissue resistance, also report a decrease in airway resistance, especially at high PEEP. Normalizing resistances for lung volume (i.e., specific resistance) caused these changes to disappear.275 Partitioning resistance into lung and chest wall components, Pelosi et al275 found that the increase in total and tissue respiratory system resistance, consequent to PEEP, could be mainly ascribed to the lung rather than the chest wall. Gattinoni et al282 found that total respiratory resistance increased with PEEP in pulmonary ARDS, mainly because of a marked increase in lung additional resistance.282 In contrast, patients with extrapulmonary ARDS, characterized by higher chest wall tissue resistance and lower lung tissue resistance than pulmonary ARDS, did not show any change in total respiratory resistance with PEEP.282
Effect of Positive End-Expiratory Pressure on Respiratory Mechanics in Chronic Obstructive Pulmonary Disease
In COPD patients, lung and chest-wall static compliance are reported to be similar to those of normal subjects,354 unless expiratory flow limitation and dynamic hyperinflation causes lung overdistension. Depending on the level of PEEP, static compliance may decrease (if overdistension occurs),238,294,355,356 stay unchanged,357 or even slightly increase (if recruitment of new lung units occurs)252 (see Fig. 10-5C).
Total end-expiratory alveolar pressure (PEEPTOT) may markedly exceed preset PEEP when PEEPi is present (see Fig. 10-4). Rossi et al260 demonstrated that failure to take PEEPi into account caused static compliance to be underestimated by up to 48%. During volume-targeted controlled ventilation, an increase in inspiratory flow resulting in a prolonged expiratory time causes PEEPi to decrease. Thus, the value of static compliance calculated without correcting for PEEPi will falsely (and paradoxically) increase.260,252 The presence of a LIP on the pressure–volume curve at ZEEP may be partly caused by PEEPi, at least in patients with expiratory flow limitation.358
Patients with COPD have a high resistance to air flow.59,238,357,359,360 In patients with expiratory flow limitation, PEEP should have little influence on expiratory flow until PEEP exceeds PEEPi.260 Other studies, however, suggest that PEEP 5 to 10 cm H2O might partly decrease expiratory resistance, particularly at the end-expiration, and result in faster and more uniform lung emptying.294,357,361,362
Recent studies provide novel information about the effects produced by PEEP application on diaphragmatic electrical activity, assessed by transesophageal electromyography. Allo et al363 induced ARDS in rabbits by intratracheal instillation of hydrochloric acid: eleven animals, five of which had been previously vagotomized, underwent a short-term protocol where PEEP and inspiratory assistance delivered by neurally adjusted ventilatory assist (NAVA) were varied over a few minutes. After hydrochloric acid instillation in the nonvagotomized rabbits, tonic (i.e., continuous throughout the respiratory cycle) diaphragmatic electrical activity increased without PEEP, while phasic (i.e., timed with inspiration) diaphragmatic electrical activity became smaller and irregular. A stepwise increase in PEEP progressively attenuated tonic diaphragmatic electrical activity and increased phasic diaphragmatic electrical activity; when PEEP was subsequently reduced, tonic and phasic diaphragmatic electrical activity returned back to the initial values. In the group of vagotomized rabbits, conversely, tonic diaphragmatic electrical activity did not rise after lung injury and was consequently unaffected by PEEP, while phasic diaphragmatic electrical activity and VT were on average much higher than in the nonvagotomized animals. These data may suggest that tonic diaphragmatic electrical activity could result from vagally mediated reflexes acting to conserve end-expiratory lung volume and PEEP might decrease tonic diaphragmatic electrical activity by recruiting lung volume.
Using the same animal model, Beck et al364 applied the same level of inspiratory assistance by NAVA through an endotracheal tube in the presence of PEEP (8.5 ± 3.3 cm H2O) and in the absence of PEEP. An instantaneous increase in tonic diaphragmatic electrical activity was observed upon PEEP removal. Following extubation and initiation of NAVA without PEEP via a mask, however, tonic diaphragmatic electrical activity returned to the previous values. The authors considered the increase in tonic diaphragmatic electrical activity during invasive ventilation without PEEP as a mechanism through which the diaphragm assumes the role of conserving end-expiratory lung volume, replacing the activity of the glottal muscles that is lost when the endotracheal tube is in place.
In twenty adult patients with ARF of varied etiology undergoing NAVA, Passath et al365 evaluated the effects of different levels of PEEP. A stepwise reduction of PEEP from 20 to 1 cm H2O increased inspiratory diaphragmatic electrical activity by 34%, while VT and breathing rate did not significantly change. The ratio of VT to diaphragmatic electrical activity, an approximation of neuroventilatory efficiency, showed broad individual variability at different PEEP levels and may help in identifying the level of PEEP at which tidal breathing occurs at minimal diaphragmatic electrical activity cost.
Recent decades have brought the recognition that mechanical ventilation per se, in ARDS, may damage the lung and/or aggravate preexisting lung injury.325 This so-called ventilator-induced lung injury (VILI)325,366,367 is now widely recognized and supported by a large number of animal experimental studies220,368 and recent data in patients.6,369,370 Attempts to prevent VILI have modified the ventilator approach to patients with ARDS.324 Two main mechanisms for VILI are: (a) alveolar220,368 and bronchial overdistension371,372 occurring at high volume and transpulmonary pressure, and (b) repeated alveolar and small airways collapse and reopening at low end-expiratory volume.217,371,373 The common pathway for these two mechanisms is mechanical stress on the terminal units (including bronchiolar and alveolar walls).374–376 Other factors, including elevated FIO2, high blood flow, high inspiratory flow, and intensity of local inflammation, may play a role in aggravating or inducing lung injury.
PEEP may have opposing consequences, depending on its effects on the two aforementioned mechanisms of VILI (alveolar overdistension and cyclic alveolar collapse and reopening) (Fig. 10-8).225,313 In one study, PEEP (13 cm H2O) caused recruitment of nonaerated regions, and induced overdistension of already aerated lung areas in three patients.36
Since the 1970s, high ventilator pressures have been known to rupture alveoli and cause air leaks.377 By increasing airway pressure, PEEP may promote alveolar overstretching,39,201,202,225,311 ultrastructural damage,372 worsening of pulmonary edema,157 and triggering of local and systemic inflammation.378 Some early studies suggest that end-inspiratory lung volume, rather than high intrathoracic pressure, may be the major determinant of ventilator-induced lung edema (“volutrauma”), at least in normal lungs.220,379,380 PEEP, however, may also protect against edema accumulation during ventilation at high end-inspiratory pressure and end-inspiratory lung volume,217–220,381 although alteration in microvascular permeability may not be prevented.219,220 Several factors may explain these apparent contradictions: (a) differences in experimental setup (isolated lung vs. intact animals), (b) levels of PEEP and inspiratory airway pressure, and (c) driving pressure (difference between end-inspiratory and end-expiratory alveolar pressures, which, for a given pulmonary elastance, depends on VT size).325 In isolated lung with constant perfusion, PEEP augments edema formation probably because of increased filtration across extraalveolar vessels associated with lung overdistension.382,383 Conversely, in intact animals, PEEP does not affect edema formation, probably because of balancing between the PEEP-induced increase in end-inspiratory lung volume (which increases fluid filtration), and concomitant reduction in cardiac output and blood pressure (which reduce filtration pressure).384–386
The role of PEEP-induced changes in hemodynamics on edema accumulation has been suggested by several authors.219,387 In an animal model, Dreyfuss and Saumon219 showed that lung edema was reduced during ventilation with PEEP 10 cm H2O, as opposed to ZEEP, despite identical end-inspiratory pressures. When the drop in arterial pressure produced by PEEP was corrected by dopamine infusion, pulmonary edema increased in direct proportion to systemic blood pressure. The increase in permeability edema was, however, less than that observed during ventilation at ZEEP, suggesting that hemodynamic modification is not the only factor explaining the effect of PEEP on edema formation.219,388 Indeed, reduction in driving pressure while keeping end-inspiratory lung volume and pressure constant (as obtained by increasing PEEP while reducing VT) may reduce edema and the severity of cellular damage; these findings suggest that a decrease in tissue stress may explain this protective effect of PEEP.217–220,389 The greater amount of alveoli available to clear edema, consequent to PEEP-induced alveolar recruitment, might also contribute.388
PEEP may also exert beneficial effects in localized lung injury. De Prost et al assessed the dispersion of localized alveolar flooding and alveolar protein permeability in rats undergoing local instillation of albumin solution followed by different ventilator strategies with varying levels of VT and PEEP.390 High VT ventilation caused alveolar liquid dispersion, which PEEP had prevented; this occurred even when VT was kept constant, thus producing higher end-inspiratory pressures that did not exceed the safe limit of 30 cm H2O. PEEP also reduced the increase in alveolar protein permeability caused by high VT. In an animal model of unilateral lung injury, Schreiber et al reported that stepwise increases in PEEP, from zero up to a relatively moderate level of 13 cm H2O, reduced the inflammatory response in the injured lung, did not result in detrimental effects on inflammation in the healthy lung, and preserved oxygenation.391
PEEP-associated preservation of surfactant may further explain the benefit of PEEP on high-volume mediated VILI.217 Thus, severity of overinflation is one major determinant of VILI.321,378,392 When ventilator-induced lung edema is produced by high VT, the combination of PEEP and VT reduction decreases the severity of injury at the same end-inspiratory pressure. When PEEP, however, is not accompanied by VT reduction, excessive inspiratory pressures and additional overstretching occur, further increasing the rate of edema formation and tissue injury219 and triggering systemic inflammation.378
In a mechanically heterogeneous lung, noninflating collapsed tissue is surrounded by open airspaces. Mead et al374 suggested that recruitment of nonaerated lung units induces a local stress to alveoli and bronchioles, which is substantially higher than the average transpulmonary or transbronchial pressure, respectively, because of alveolar and small airways interdependence.371,375 If this process recurs cyclically, high shear forces may damage the alveolar and airway epithelium, overstretch fragile microvessels, deplete surfactant, and initiate or worsen inflammation. By recruiting nonaerated portions, and stabilizing airways and lung units prone to repetitive opening and collapse, PEEP plays a key role in protecting the lung from the mechanical shear stress and tissue injury produced by ventilation at low end-expiratory lung volume (“atelectrauma”).373,374,393–398
A large body of animal data supports the protective effect of PEEP on low-volume mediated VILI.217,218,338,373,389,393,395,398–402 By minimizing shear stress associated with cyclic opening and collapse, PEEP may attenuate inequalities in the regional distribution of tidal ventilation, thus avoiding or limiting overdistension in the less-injured lung zones.374,375 By increasing end-expiratory lung volume, PEEP can also prevent surfactant loss and preserve surfactant function.400,403–406 By minimizing mechanical stress, PEEP may also ease the intensity of ventilator-induced lung inflammation393,396,407 and remodeling,397 and reduce the decompartmentalization of a number of inflammatory mediators408 and bacteria from the lung into the circulation (“biotrauma”).409–411 These aforementioned mechanisms may play a role in initiating or propagating the systemic inflammatory response, which contributes to the multiple organ failure often observed in the terminal stage of ARDS.412–414 The local and systemic beneficial effects of PEEP are, however, dependent on the level of applied PEEP. Several authors, in fact, have suggested that higher-than-optimal levels of PEEP, defined as the PEEP required for maximum oxygenation and/or minimum elastance, may worsen lung mechanics, increase the amount of atelectasis and the lung-tissue expression of type III procollagen messenger RNA (an early marker of lung parenchyma remodeling), and also promote bacterial translocation.415,416
Recent clinical trials show that protective ventilator strategies, reduced VT (to limit the end-inspiratory stretch),6,369,370 and high PEEP (to avoid cyclic opening and closing)6,369 are associated with decreased pulmonary and systemic cytokine response,369,370 less organ dysfunction,417 and reduced mortality in patients with ARDS,6,370 compared to conventional, injurious mechanical ventilation. Although the mechanisms whereby VILI causes organ dysfunction are not completely understood, a recent study suggests that cell apoptosis may be involved.414 Rabbits ventilated with a low VT and high PEEP showed less epithelial apoptosis in the kidney and small intestine than did rabbits ventilated with a high VT and low PEEP.414 The former rabbits had more apoptotic cells in the lung, whereas the latter rabbits had less lung apoptosis and necrosis of alveolar epithelial type III cells.414 Analysis of plasma samples from a previous clinical trial369 revealed higher levels of soluble Fas ligand (a proapoptotic factor) in patients who received conventional ventilation than in patients who received protective ventilation; changes in soluble Fas ligand were correlated with changes in plasma creatinine.417
The lungs and heart are subject to variations in intrathoracic pressure. How alterations in intrathoracic pressure affect the heart and intrathoracic vessels varies substantially and depends on several factors, such as mechanical properties of the lung and chest wall, type of ventilation (spontaneous vs. mechanical), blood volume, and left-ventricular function. The complex interplay of these factors and the effects of PEEP on heart–lung interactions have been extensively reviewed.418–426
Positive airway pressure causes a drop in cardiac output secondary to a decrease in cardiac filling (preload); this was initially attributed to a reduction in the pressure gradient for venous return, determined by the rise in right-atrial pressure consequent to increased intrathoracic pressure.50,66,427–430 The PEEP-mediated decrease in the gradient for venous return, however, is less than expected because PEEP produces a concomitant rise in mean systemic pressure431 (the circulatory filling pressure representing the upstream pressure for venous return). In patients without lung disease undergoing implantation of defibrillator devices under general anesthesia, Jellinek et al432 measured right-atrial pressure and mean systemic pressure at airway pressure zero and 15 cm H2O during 15-second periods of apnea when ventricular fibrillation was induced to test the defibrillator. Rising airway pressure produced a drop in left-ventricular stroke volume.432 Right-atrial and mean systemic pressure, however, increased equally, showing that the reduction in venous return was not determined by a decrease in pressure gradient.432 The rise in mean systemic pressure may result from a reduction in vascular capacitance determined by neurovascular reflexes,433 displacement of blood from the pulmonary to the systemic circulation,434 and descent of the diaphragm, which increases the upstream pressure for venous return by augmenting intraabdominal pressure.435 These homeostatic adaptations, however, may be counteracted by a concomitant increase in venous resistance,431,436 suggesting that PEEP may alter venous return by affecting the peripheral venous circulation.
The effects of PEEP on hemodynamics strongly depend on intravascular volume. Cardiac output can be restored by increasing the ventricular filling through volume infusion.47,437–440 PEEP discontinuation produces a rise in cardiac filling, proportional to the pressure withdrawn and the circulating blood volume.47,441 In hypervolemic and hemodynamically stable patients who underwent cardiac surgery, van der Berg et al found that maintaining airway pressure up to 20 cm H2O for 25 seconds produced minimal variations in right ventricular output despite a concomitant rise in right atrial pressure.435
In summary, PEEP reduces cardiac output through a decrease in venous return that is not a primary consequence of a decrease in its pressure gradient. Regardless of the cause, the drop in cardiac output can be counteracted by blood volume expansion.
PEEP alters both left- and right-ventricular configurations and reduces left-ventricular diastolic compliance by augmenting right-ventricular afterload.49,442–444 An increase in lung volume causes a rise in pulmonary vascular resistance by directly compressing the alveolar vessels.445 The interconnections between lung volume and pulmonary blood flow are not straightforward, because blood expelled from the alveolar vessels can be retained in the extraalveolar vessels.446,447 When airway pressure is augmented, right-ventricular outflow impedance (i.e., afterload) is also increased.426,448 In patients with ARDS, high levels of PEEP can cause or worsen tricuspid regurgitation.449
The fall in cardiac output with PEEP has been also attributed to the stress exerted by the right ventricle on the interventricular septum,443 which is displaced leftward and restricts left-ventricular filling.49 Culver et al450 altered right-ventricular afterload, through a partial occlusion of the main pulmonary arteries, and found that this did not produce the same hemodynamic changes induced by increases in lung volume; these findings suggest that ventricular interdependence (caused by an increased right-ventricular afterload) was unlikely to be the main mechanism for PEEP-mediated fall in cardiac output. Wise et al69 evaluated the impact of PEEP 15 cm H2O on left-ventricular compliance in a study where they bypassed the right heart to exclude the effects of interventricular interdependence; they concluded that the elevation in left-ventricular filling pressure was mainly consequent to pericardial compression. In patients with ARDS, Dhainaut et al440 did not find any changes in ventricular diastolic compliance and ejection fraction when PEEP was increased up to 20 cm H2O during controlled ventilation; they attributed the reduction in cardiac output mainly to the preload effect.
More recently, Gernoth et al reported that an open-lung strategy with high PEEP (20 cm H2O), which improved oxygenation without significantly affecting PaCO2, caused no major hemodynamic alteration, while right- and left-ventricular function, respectively, were improved and unchanged.451 An impairment in right-ventricular function and hemodynamics, however, has been reported when PEEP is increased at constant plateau pressure, with significant worsening of respiratory acidosis despite improvement in oxygenation.452
In summary, PEEP increases pulmonary vascular resistance and right-ventricular afterload and may therefore increase the stress exerted by the right ventricle, which has to maintain an adequate output to guarantee left-ventricular filling. Right-ventricular systolic overload may result in leftward displacement of the interventricular septum; the effect on left-ventricular function seems to be adjunctive, rather than a major determinant of the PEEP-mediated reduction in cardiac output.
A reduction in lung compliance reduces the transmission of airway pressure to the pericardial space,453,454 and to the pleural space. The transmission of PEEP to the pleural space increases when chest wall compliance is reduced455 and decreases when chest wall compliance is increased.454 Venus et al456 found that the fraction of PEEP transmitted to the pleura was reduced from 62% to 34% and to the pericardium from 54% to 36%, in intact and acutely injured lungs, respectively. VT was kept constant throughout the study and the reduction in cardiac output produced by PEEP did not differ before and after inducing lung injury, because absolute pericardial pressure at end-expiration did not differ.456
Left-ventricular afterload is the force opposing contraction. It corresponds to the tension developed by the contracting cardiac muscle. It is determined by both the systemic arterial resistance and the transmural pressure exerted on the left-ventricular wall; that is, the difference between the systolic pressure and the pressure surrounding the heart (i.e., intrathoracic pressure). A reduction in left-ventricular afterload is achieved either by decreasing systemic arterial resistance (through vasodilator administration) 457or increasing intrathoracic pressure.69–71 In healthy subjects with normal cardiac function the dominant action of increase in intrathoracic pressure is reduction in venous return; the consequences of the lowered transmural pressure are rather small (see Fig. 10-2A). In patients with poor left-ventricular function and congestive heart failure, cardiac output is relatively insensitive to reduction in venous return because left-ventricular filling pressure and diastolic volume are elevated.67 Thus, the net effect of a rise in intrathoracic pressure is a reduction in left-ventricular transmural pressure (see Fig. 10-2B).68,458,459 Conversely, a decrease in intrathoracic pressure raises afterload by augmenting left-ventricular transmural pressure (see Fig. 10-2B).332,460
When afterload exceeds the capacity of the left ventricle, pulmonary edema ensues, establishing a vicious circle (Fig. 10-9). Because of worsening pulmonary compliance332,460,461 and resistance,460,462–464 the inspiratory muscles must exert a stronger effort to achieve adequate alveolar ventilation. Thus, inspiratory intrathoracic pressure becomes more negative, and afterload increases. The combination of reduced O2 delivery465 and increased metabolic cost of breathing464–466 may precipitate respiratory muscle failure,466 which further worsens gas exchange.
Figure 10-9
Pathophysiologic mechanisms and pathways of cardiogenic pulmonary edema. Worsening in pulmonary mechanics increases negative swings in intrathoracic pressure and left-ventricular afterload. Worsening in gas exchange decreases oxygen delivery to the heart and the respiratory muscle. See text for further explanation. CO, Cardiac output; LV, left ventricle; O2, oxygen; PaO2, partial pressure of arterial oxygen.
The impact on left-ventricular transmural pressure of the increase in intrathoracic pressure produced by CPAP 10 cm H2O during expiration is rather small (see Fig. 10-2C). Conversely, the improvement in pulmonary mechanics466 generated by the same CPAP may minimize the swings in intrathoracic pressure466,467 and hence decrease afterload,467,468 thereby improving left-ventricular function (see Figs. 10-2C and 10-10).72,468 CPAP may simultaneously improve gas exchange72,73,146 by reducing venous admixture10,146 and avert failure of the respiratory muscles by improving their O2 balance10 (Fig. 10-10).
Figure 10-10
Cardiopulmonary effects of CPAP in cardiogenic pulmonary edema. By improving pulmonary mechanics, CPAP reduces negative swings in intrathoracic pressure and left-ventricular afterload. By improving gas exchange CPAP increases oxygen delivery to the heart and the respiratory muscle. See text for further explanation. LV, left ventricle; O2, oxygen; PaO2, partial pressure of arterial oxygen.
The effect of PEEP on O2 delivery depends on its relative effects on cardiac output and arterial oxygen content: in other words, on the balance between the pulmonary and hemodynamic consequences of PEEP. PEEP-induced increase in PaO2 and arterial oxygen content may be accompanied by a decrease in O2 delivery, because of concomitant decrease in cardiac output.46 The impact of PEEP on O2 delivery depends primarily on its hemodynamic effect. By decreasing cardiac output and consequently O2 delivery, PEEP may worsen O2 balance and promote pathologic supply-dependency of O2 delivery.430,469–472
The effect of protective ventilation on O2 delivery was evaluated in patients with ARDS.473–475 In forty-eight patients with severe ARDS, Carvalho et al474 found that PEEP up to 24 cm H2O (average: 16 cm H2O) produced an increase in cardiac output, heart rate, and O2 delivery, and decreased systemic vascular resistance and plasma lactate. The authors speculated that the good tolerance of high PEEP may have been a consequence of the acute hemodynamic effects of hypercapnia and the observed very low lung compliance.474 No correlation was found between changes in PEEP and cardiac output. A strong inverse correlation existed between changes in plateau pressure and cardiac output. These findings suggest, in line with other reports,202,318 that the negative hemodynamic effect classically attributed to PEEP may depend more on the associated high inspiratory pressure (if VT is not concomitantly reduced).474
PEEP decreases urinary output,47,54,55,476–500 sodium excretion,55,476–478,480–482,484–490,493,500 and creatinine clearance.476,480,481,484,490 Although there is general consensus about these effects of PEEP, studies investigating the mechanisms are conflicting. Discrepancies are related to differences in experimental design, such as diverse subjects, trial duration, type and severity of underlying disorder, volemic state, use of drugs and anesthetics (which commonly cause sympathetic depression), and level of PEEP. The decrease in urinary output caused by PEEP has been attributed to multiple factors, such as fall in cardiac output54,477,491 and renal blood flow,477,479,487,491,495 reduced intravascular volume,54 reflex sympathetic nerve activation,497 and altered release of hormones including catecholamines,476 renin–angiotensin–aldosterone system,476,488,498–500 antidiuretic hormone,476,488 and atrial natriuretic factor.485,486,488–490,493
In dogs receiving a constant fluid infusion, Berry et al55 observed that the time needed for urinary output to approximate the rate of fluid infusion was 20, 27, and 46 hours during spontaneous breathing, positive-pressure ventilation and positive-pressure ventilation plus PEEP 10 cm H2O, respectively. Priebe et al54 found that renal impairment caused by PEEP 10 cm H2O in anesthetized dogs primarily resulted from a reduction in intravascular volume. In swine, Venus et al476 found that the addition of PEEP to positive-pressure ventilation caused a fall in cardiac and urinary output, associated with increase in plasma antidiuretic hormone, renin, epinephrine, and norepinephrine in normovolemic animals, but not in animals that received volume expansion through crystalloids. In six neurologic patients with intact cardiopulmonary and renal function who were receiving positive-pressure ventilation plus PEEP 15 cm H2O, an increase in lower body pressure via military antishock trousers improved systemic and renal hemodynamics and decreased plasma norepinephrine, with no change in total blood volume.499 Urinary output and sodium excretion did not improve. The authors concluded that increased plasma renin and sympathetic activation were the main determinants of renal impairment with PEEP.499
Annat et al477 evaluated the short-term effects of PEEP 10 cm H2O in normovolemic patients without cardiac and renal abnormalities and little or no respiratory failure who were receiving positive-pressure ventilation. PEEP decreased urinary output by 34%, renal blood flow by 32%, and sodium excretion by 33%; the associated drop in cardiac output was 15%.477 PEEP also increased urinary antidiuretic hormone and plasma renin and aldosterone.477 In a similar setting, Payen et al491 found that PEEP 15 cm H2O reduced urinary output (55%) and fractional sodium excretion (39%); cardiac index fell by 21%. Plasma antidiuretic hormone did not vary, and norepinephrine increased.491
PEEP increases sympathetic activity.501 Nevertheless, the observation by Fewell et al494 that the effects of PEEP on renal function are mediated by sympathetic activity has not been confirmed.481,492 PEEP decreases plasma atrial natriuretic factor.478,485,486,488,493 This may contribute to the reduction in urinary output and sodium excretion, probably as a result of the reduced atrial transmural pressure consequent to the raised intrathoracic pressure.485
In summary, PEEP may alter renal function by reducing cardiac output and renal blood flow to an extent that depends on the volemic state and on the amount of applied pressure, although other mechanisms, including several hormones, may play a role.
PEEP decreases splanchnic blood flow43,57,58,502–504 and causes hepatic congestion.503 The reduction in splanchnic blood flow is consequent to a fall in cardiac output secondary to impaired central hemodynamic43,57,58,502–504; it is less pronounced when cardiac output is maintained either by expanding blood volume57,58 or inotropic drugs.504 In hemodynamically stable patients with ARDS, Kiefer et al505 found that PEEP 13 cm H2O (5 cm H2O increment above the preset values), did not decrease cardiac index or splanchnic blood flow and metabolism, suggesting that PEEP does not affect splanchnic blood flow. Other factors, however, such as hepatic outflow resistance, may play a role.58,506 In swine, Brienza et al506 found that PEEP 15 cm H2O reduced portal vein flow through an increase in liver venous resistance; the authors postulated a direct compressive effect caused by diaphragmatic descent on the liver.
Sha et al502 applied increasing PEEP (up to 20 cm H2O) and found proportional decreases in hepatic blood flow. Because the rate of decrease in hepatic O2 delivery exceeded that of cardiac output and hepatic blood flow, the authors concluded that hepatic O2 delivery was reduced primarily because of the drop in portal venous O2 content.502 Aneman et al507 evaluated the impact of PEEP 10 cm H2O on mesenteric and hepatic blood flow in patients under general anesthesia (during elective surgery for gastric or pancreatic neoplasm). Neither arterial pressure nor arterial, portal, and hepatic venous norepinephrine were significantly affected by PEEP.507 A decrease in portal blood flow was associated with a rise in mesenteric vascular resistance; conversely, an increase in hepatic arterial flow was associated with a drop in hepatic arterial resistance.507 Mesenteric O2 delivery was reduced and hepatic O2 delivery remained unchanged.507 Mesenteric and hepatic O2 did not significantly vary with PEEP.507 In similar patients, Berendes et al43 found that hepatic venous lactate did not change with PEEP up to 15 cm H2O, and concluded that a critical reduction in splanchnic oxygenation did not occur.
In spontaneously breathing normal subjects, Fournell et al508 reported that CPAP levels up to 10 cm H2O progressively altered gastric mucosal microvascular O2 saturation, as assessed by reflectance spectrophotometry. Lehtipalo et al44 found that PEEP 10 cm H2O had little effect on intestinal perfusion pressure; mesenteric blood flow and oxygenation were maintained until the intestinal perfusion pressure exceeded 33 mm Hg. In patients with septic shock, Träger et al509 increased PEEP up to 15 cm H2O. PEEP decreased cardiac index and hepatic venous O2 saturation.509 Hepatic metabolic performance, assessed by glucose production, fell at each PEEP level in patients who did not survive, whereas it decreased only at the highest PEEP among survivors.509 In 141 unselected critically ill patients, PEEP greater than 5 cm H2O during mechanical ventilation was found to be a risk factor for liver dysfunction, as defined by serum bilirubin equal to or greater than 2 mg/dL for at least 48 hours.510
In sixty-five patients who underwent liver transplantation, Saner et al found in the immediate postoperative period that application of PEEP up to 10 cm H2O did not affect venous liver outflow, portal vein and hepatic artery flow, and systemic hemodynamics.511 In a retrospective observation study, Kocabayoglu et al reviewed the records of 50 patients who underwent mechanical ventilation for more than 7 days because of pulmonary complications following liver transplantation and found that PEEP equal to or greater than 10 cm H2O did not harm liver-graft function.512
In summary, PEEP reduces hepatic and splanchnic blood flow when determining a drop in cardiac output, although other factors, such as regional outflow resistance or derangements in microcirculation consequent to sepsis, are likely to play a role.
High intracranial pressure (ICP) and reduced cerebral perfusion pressure (the difference between mean arterial pressure and ICP) are common in several acute neurologic and neurosurgical intensive care unit patients, particularly those who have focal or generalized cerebral edema. Hypoxemic respiratory failure secondary to ARDS is a fearful complication in these patients leading to use of PEEP.513
ICP may be altered by PEEP through a rise in right-atrial pressure, which increases superior vena cava pressure and hence reduces cerebral venous return.53,514,515 The effects of PEEP on ICP depend on the amount of intracranial compression, and are of little importance when cerebral compliance is not altered.52 In a study evaluating twenty-five head-injured patients, Apuzzo et al52 found that PEEP caused an increase in ICP in only twelve patients who had low cerebral compliance, and reduced cerebral perfusion pressure below 60 mm Hg in only six of the twelve patients.52 In normal healthy volunteers, Hormann et al516 found that alterations in ICP and cerebral perfusion pressure produced by CPAP 12 cm H2O were slight and not of clinical relevance. Body position also affects the impact of PEEP on ICP. PEEP raises ICP through an increase in the downstream venous pressure; thus, elevating the head above the chest should lessen the transmission of intrathoracic pressure to the venous sinuses and mitigate the effects of PEEP on ICP. In supine anesthetized patients undergoing posterior fossa surgery, Lodrini et al517 found that PEEP up to15 cm H2O caused proportional increases in central venous pressure and ICP; in the sitting position, PEEP also increased central venous pressure, but ICP changed little in most of the patients.
Muench et al found that increases in PEEP up to 25 cm H2O in healthy pigs did not produce any adverse effect on ICP, cerebral blood flow, or tissue oxygenation. On the contrary, in patients with subarachnoid hemorrhage, reduction in mean arterial pressure consequent to application of PEEP up to 20 cm H2O caused a significant decrease in cerebral blood flow attributable to the altered cerebrovascular autoregulation.518 Caricato et al studied the effects of increasing PEEP up to 12 cm H2O in twenty-one patients with severe head injury or subarachnoid hemorrhage and who were receiving mechanical ventilation; static compliance of the respiratory system was normal in thirteen patients and reduced in the other eight.519 The authors found that PEEP caused a decrease in mean arterial pressure and cerebral perfusion pressure in the first subgroup of thirteen patients, whereas it did not produce any significant change in the eight patients with reduced respiratory compliance.519 In twelve severely brain-injured patients with ARDS and high ICP, Mascia et al randomly applied 5 and 10 cm H2O of PEEP; recruited volume and elastance were used to classify patients as recruiters (n = 6) and nonrecruiters (n
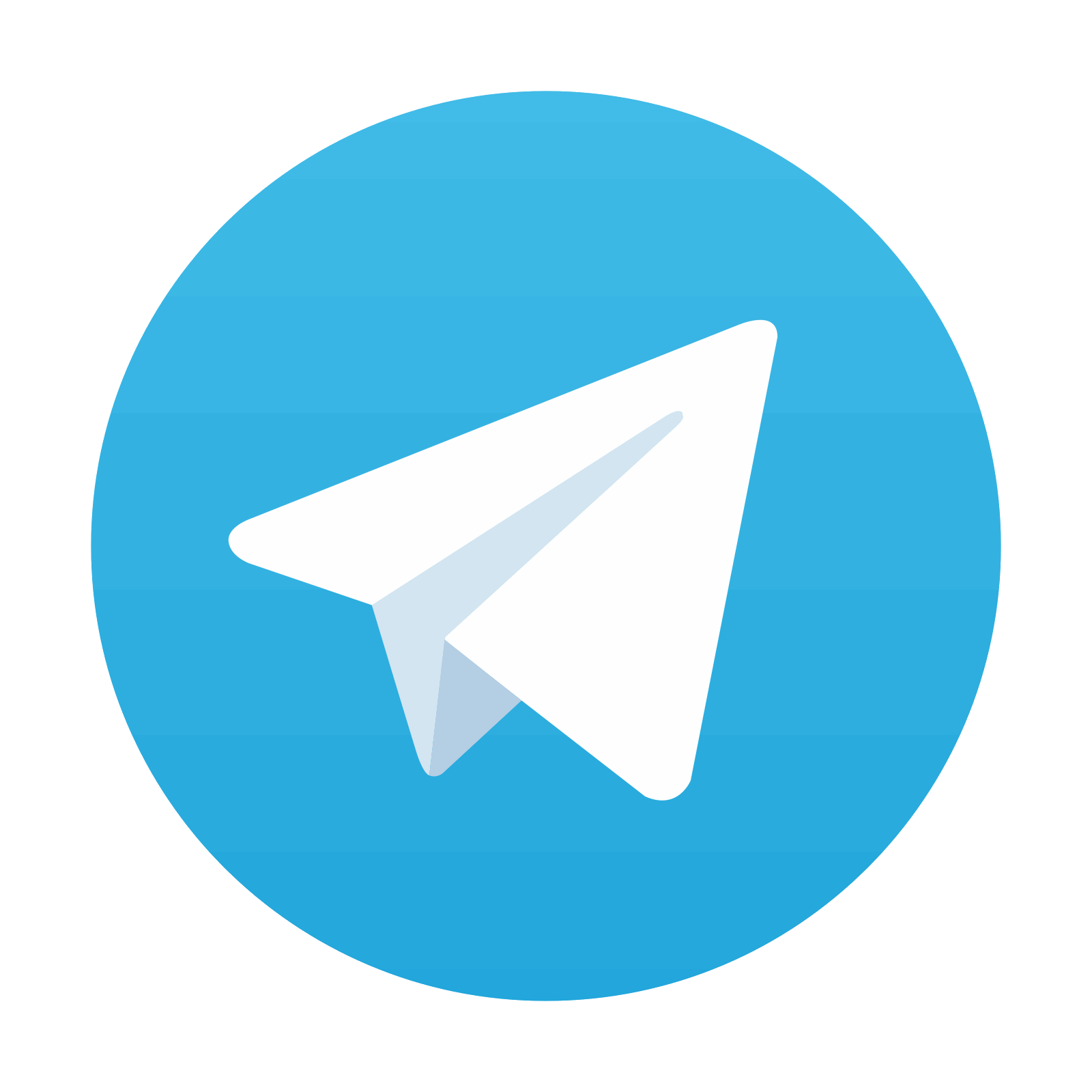
Stay updated, free articles. Join our Telegram channel

Full access? Get Clinical Tree
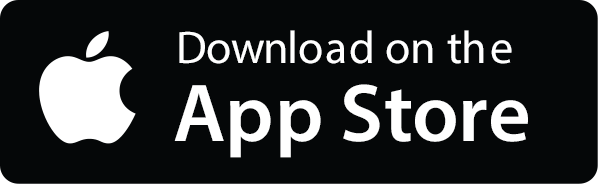
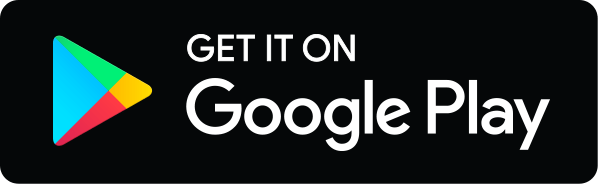
