There is insufficient evidence to evaluate the effectiveness of protocol-directed sedation regimes as results from the two randomized controlled trials were conflicting [51, 52]. Patients who are not immediately weaned need further immediate assessment to identify reversible factors and then usually undergo either a strategy of progressive reduction of level of assistance, such as pressure support ventilation, or progressively longer periods of spontaneous breathing, with or without CPAP [26, 27]. There are now a wide variety of alternative modes and strategy with apparent equivalence in weaning [53–56]. Partial ventilatory support mode in patients with ALI/ARDS is associated with physiological benefits, but evidence of an impact on clinical outcomes in ARDS is lacking [56, 57]. These are outlined below under adaptive ventilation.
9.2.4 Movement to Spontaneous Breathing
In severe ARDS, the value of neuromuscular blockade and prone positioning mean that spontaneous breathing cannot be recommended in early severe ARDS [58, 59]. After this initial period, spontaneous breathing supported by modes such as ASV, APRV, BIPAP, or pressure support has appealed [30]. In less severe ARDS and as lungs recover, spontaneous ventilation has potential to improve lung ventilation at lower levels of airway pressure and traditionally has been encouraged as it requires less sedation and improves cardiopulmonary function, presumably by recruiting non-ventilated lung units, requiring a shorter duration of ventilatory support [57, 30]. In animals with severe lung injury, spontaneous breathing could worsen lung injury, and muscle paralysis might be more protective for injured lungs by preventing injuriously high trans-pulmonary pressure and high driving pressure [60]. Inspiratory pressure support provided to match the demand for a higher minute ventilation can lead to large trans-pulmonary pressure swings and TV that exceed the recommended maximums, both of which are major contributing factors to ventilator-induced lung injury and possibly to weaning failure [31, 61].
Strong spontaneous efforts in animals increase lung injury [31]. In injured lungs, the lungs’ normal fluidlike behavior is not universally present. With spontaneous breathing, the negative pleural pressures generated by diaphragmatic excursion are not uniformly transmitted across lung surfaces but concentrated in dependent regions. In the supine position, these dependent posterior regions are affected, but following prolonged prone positioning, this may also affect the ventral regions [62]. Locally increased pleural pressure variations cause underdiagnosed regional overstretching in dorsal (or dependent) lung regions, accompanying alveolar air shift from ventral to dorsal parts of the lung (i.e., pendelluft) [62]. Excessive spontaneous efforts may cause local overstretch because of a significant pendelluft effect [63].
Of concern is the cryptic damage due to high trans-pulmonary pressures, and regional overdistension will postpone successful weaning and increase both morbidity and mortality. Limiting the trans-alveolar pressure by strictly limiting driving pressures and tidal volumes generated is important [31].
9.3 Barriers to Weaning
The barriers to immediate easy weaning are shown on Diagram 2 [4]. It is noteworthy that only a few are reversible directly by manipulation of the ventilation support, the primary focus on many early “weaning” attempts.
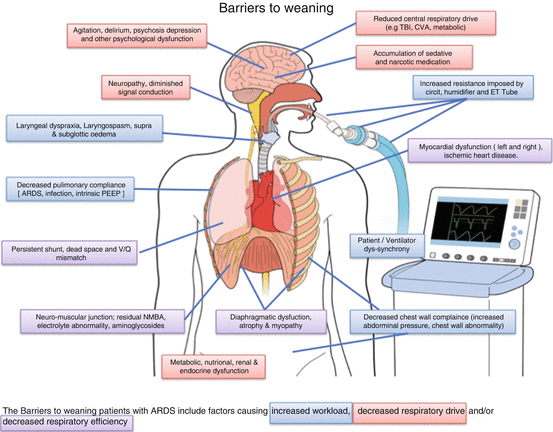
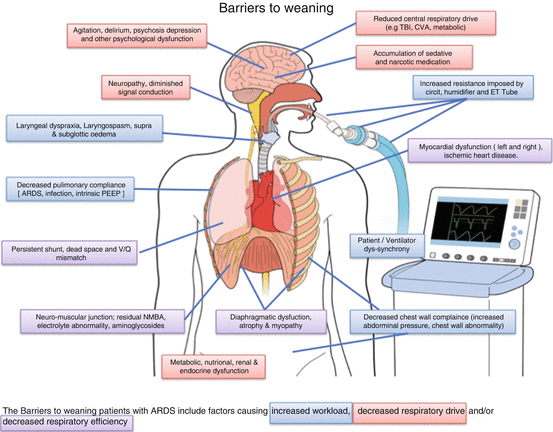
9.3.1 Increased Respiratory Load
With ARDS the residual shunt, increased dead space, and ongoing poor ventilation-perfusion matching does not resolve immediately [64]. Weaning patients have supranormal minute ventilation requirements, increased airway resistance, decreased pulmonary and/or chest wall compliance changes, and thus a greater work of breathing [65]. Decreased pulmonary compliance can arise from reversible causes like pleural effusions and pulmonary edema. Drainage or diuretics may improve compliance and oxygenation, decrease trans-pulmonary pressure, and shorten weaning time [66, 67].
9.3.2 Decreased Respiratory Drive
A central respiratory drive is necessary for ventilatory activity to continue once the ventilator support is removed. Commonly, there is a reduced respiratory drive with an inadequate response to either hypercarbia or hypoxemia. Carbon dioxide responsiveness is seldom tested formally, although ventilatory response to reduction in pressure support is used as part of the weaning algorithm. Responsiveness to carbon dioxide assessed from the 100 ms occlusion pressure has been shown to predict weaning [71].
9.3.3 Excess Sedation and Analgesia
The triad of adequate analgesia, control of delirium, and optimal sedation to facilitate tolerable MV is never more important than during weaning [72]. Early sedation depth predicts delayed extubation [73, 74]. This association is confounded by severity of illness, and with neuromuscular blockade and prone positioning in severe ARDS, both precipitating deeper sedation, being recommended therapy for severe ARDS, it is not surprising that ARDS patients are deeply sedated [58, 59]. Kress demonstrated that daily interruption of sedative infusions reduced the duration of MV, but less than one third of the studied group had ARDS or pulmonary edema [75]. Protocol-directed sedation is controversial as results from the two randomized controlled trials were conflicting [51, 52].
Prolonged weaning can be a complication of inappropriate dosing sedative/hypnotic medication, with delayed clearance in multi-organ failure, a common feature of ARDS patients [6, 76]. Increased understanding of sedation goals by attending staff principles may further reduce ventilation duration [77]. Change in sedation choice may contribute to shorter weaning periods. A fivefold increase in propofol use and a 70% reduction in midazolam use over 8 years were associated with a decline in MV duration, tracheostomy rate, and ICU and hospital length of stay [78]. Sedation techniques, particularly those that include the use of benzodiazepines, may increase delirium, agitation, withdrawal, and other neuropsychiatric conditions, which either directly or indirectly delay weaning [79, 80].
Withdrawal syndromes were associated with high doses of analgesic, and sedative medications with neuromuscular blockade and prolonged MV means that patients with ARDS are at increased risk of withdrawal syndrome [81].
9.3.4 Cardiac Load
Cardiac dysfunction is among the most common barriers to weaning [82].
The onset of ARDS respiratory failure may be precipitated by, exacerbate or importantly mask, congestive and right heart failure. Positive pressure reduces pulmonary edema, provides increased alveolar pressures, and reduces afterload. With weaning, spontaneous breathing is reestablished; the process reverses with a negative pleural pressure during inspiration [83]. This decrease in intrathoracic pressure increases the systemic venous return pressure gradient, the right ventricular (RV) preload, the central blood volume, and subsequently the LV preload [84]. A second effect is a decrease of the LV ejection pressure gradient, increasing the LV afterload [85]. As a consequence, respiratory and cardiac failure may occur, associated with increased work of breathing and hypoxemia.
Diagnosis and monitoring, augmented by echocardiography, allow titration of treatment by diuretics, vasodilators, inotropes, and coronary angioplasty when indicated. Using diuretics guided by brain natriuretic peptide (BNP) measurements shortens weaning suggesting that negative fluid balance using diuretics could hasten extubation [16]. The use of a conservative fluid strategy shortened MV in patients with acute lung injury although poor longer-term cognitive outcomes are concerning [66, 86].
9.3.5 Muscle Atrophy and Weakness
Early muscle changes are driven by inflammation and disuse, with atrophy from increased protein degradation [28, 87]. Late-phase muscle weakness persists in many despite resolution of lung injury and cessation of ongoing acute inflammation [87]. Late-phase muscle dysfunction may reflect a failure of the musculoskeletal system to regain homeostatic balance. These differences may require different therapies. Electrical muscle stimulation for those that cannot exercise has been suggested although this novel technique in the ICU setting is scarce [88]. Denervation injuries, for which there are no specific therapies, may persist and delay weaning.
9.3.6 Metabolic Electrolyte and Endocrine Considerations
Metabolic alkalosis, a common disorder in ICU, impedes respiratory drive and can be caused by diuretic use, hypernatremia, and hypokalemia, all classically associated with ARDS management [89]. Increased serum bicarbonate is associated with increased ICU LOS, more days on MV, and higher hospital mortality [90]. Chloride-restrictive buffered solutions theoretically could increase metabolic alkalosis, but in an interventional trial, chloride restriction did not increase in ventilation time [91, 92]. Studies of “contraction alkalosis” and effect medications such as acetazolamide on weaning are needed [93].
Serum calcium, phosphate, magnesium, and potassium deficiency all cause muscle weakness [94]. Hypothyroidism, hypoadrenalism, and relative or absolute cortisol efficiency also contribute to weaning difficulty. Endogenous hormones, and the exogenous corticosteroids that are often used in critical illness for septic shock, directly for ARDS, or for other indications may also contribute [95]. The corticosteroids and steroid-based neuromuscular blocking agents are associated with muscular weakness, but their overall effect on weaning remains unclear [96]. Avoidance and, when present, early correction of electrolyte abnormality as routine care are crucial to successful weaning [94].
9.3.7 Psychological Dysfunction
Forty-two percent of patients weaning from prolonged MV have depression, and weaning failure is twice as common in patients with depression [97, 98]. Fatigue, excessive daytime somnolence, and associated depression also delay weaning [99].
Second-generation antipsychotic and stimulants such as donepezil, modafinil, and methylphenidate have been used in difficult-to-wean patient depression [99–103]. Whether their effect is through counteracting depression or anxiety or as a direct respiratory stimulus is unclear.
Up to 80% of patients in ICU have delirium and are associated with delayed weaning and higher 6-month mortality [72, 104]. Prevention strategies include an early and exercise/mobility. Patients exposed to an “ABCDE” bundle spent 3 more days breathing without assistance and experienced nearly half the delirium, than patients treated with usual care [77]. Haloperidol is most commonly used for ICU delirium although evidence is entirely anecdotal [72]. Dexmedetomidine is also recommended [105]. Olanzapine, risperidone, and quetiapine are also used and may have some advantages in the critically ill [72].
9.3.8 Consent, Compliance, and Comfort
Prolonged MV could produce suffering. While deeply sedated, with adequate analgesia and ventilatory support, it is unlikely that a patient “suffers.” However, later, as patient “emerges,” and weaning begins, “suffering” may manifest. Long-term outcomes from prolonged MV are worse than generally expected by relatives and physicians [106]. In a recent systematic review of studies reporting outcomes of patients requiring MV for more than 14 days, only half were successfully weaned, one in five were able to return home, and half were dead in 1 year [107]. ARDS is associated with exercise limitation, physical and psychological sequelae, decreased physical quality of life, increased costs, and the use of health-care services although there is a similar functional recovery in survivors who did and did not develop ARDS [3, 64]. Overly optimistic expectations do not encourage meaningful discussion surrounding weaning outcomes or end-of-life decision-making. Initiation of weaning is a timely juncture when the objectives of future treatments are considered.
9.4 Adjunctive Care
In addition to standard ventilation care, during weaning, some interventions need further consideration.
9.4.1 Monitoring
A venous CO2 threshold of below 45 mmHg is sensitive enough to reliable enough to exclude hypercarbia in lieu of sequential arterial samples [108]. If hypercarbia is detected, venous samples are insufficient to determine the degree of hypercarbia. End-tidal monitoring is less reliable. For end-tidal CO2 to accurately reflect arterial CO2, lung perfusion levels and alveoli CO2 must be consistent throughout the lung, and TV must be at least three times the 2.2 mL/kg anatomic dead space [109]. As TV of 6 mL/kg is recommended, and CO2 is unevenly distributed, the utility of end-tidal CO2 monitoring is limited.
Standard cardiac monitoring of vital signs and rhythm remains minimum requirements. More extensive cardiac monitoring is variable; many invasive processes to estimate cardiac performance have been withdrawn prior to weaning. Repeated echocardiograms may detect cardiac dysfunction during dys-synchrony, during weaning failure, or during the transition from controlled to spontaneous modes. Ultrasound may also detect undetected diaphragmatic dysfunction that contributes to delayed weaning [110].
9.4.2 Therapy and Mobilization
9.4.3 Tracheostomy
In some situations, tracheostomy may reduce the work of breathing, lower in-hospital mortality, and improve weaning [112, 113]. Systemic analysis of randomized controlled trials suggests that early tracheostomy generally achieved better outcomes, including more ventilator-free days, shorter ICU stays, shorter sedation, and reduced long-term mortality [114, 115].
While early tracheostomy may be desirable for weaning, timing is dependent upon having safe operating conditions, including low enough FiO2 and PEEP settings to allow safe airway manipulation. With ARDS, both the decision to wean and the identification of a “difficult-to-wean” patient are important decision points, at which the longer-term prognosis and treatment strategy, including tracheostomy, should be considered [113, 116].
9.4.4 Extubation to Noninvasive Ventilation
Noninvasive ventilation (NIV) has been used both in an attempt to prospectively reduce “invasive ventilation” time, through “early extubation,” and as a rescue therapy for patients on the threshold of failing an extubation attempt.
Early trials of NIV in post-extubation respiratory failure produced conflicting outcomes. Subsequent trials of “prophylactic” use of immediate post-extubation in patients who “fail” a SBT have been found to be beneficial [17, 117–125]. Systematic reviews have evaluated this technique and are support its clinical use in appropriate clinical circumstances [126, 127].
Extrapolating this data to ARDS requires considerable caution [128, 129]. Firstly, patients studied were predominantly suffering from chronic lung disease (CLD). Noninvasive ventilation has the capacity to reduce intubation rates and lower the length of stay and mortality, in patients with CLD when used before invasive MV. In contrast on patients with moderate to severe ARDS, NIV used prior to invasive ventilation had high intubation rates, had delivered high tidal volumes, and had a high mortality with NIV failure [61, 129].
With NIV, oxygenation is increased by a raised mean alveolar pressure, a reduction in tidal de-recruitment of alveoli, and/or a provision of high oxygen concentrations through a semi-closed system [130]. After extubation, a less than perfect NIV mask seal may produce air entrainment and airway pressures below airway “closing” pressure more frequently than during invasive ventilation [131].
While entrainment can be managed by mask adjustment, or increasing oxygen, the decreased airway pressure may result in small airway collapse with de-recruitment.
NIV may assist patients suffering from respiratory failure where work of breathing rather than hypoxia predominates. These patients are less sensitive to short periods of mask removal or leaks. In patient recovering from ARDS although NIV supports the work of breathing, there is a risk of tidal de-recruitment [130]. At lower levels of support requirement, high-flow nasal cannula (HFNC) is likely to provide better oxygenation than venturi mask and is as least effective as, and better tolerated than, NIV for treatment of post-extubation respiratory failure [19, 20]. HFNC role in ARDS is as yet undefined [21]. NIV is not recommended and if used for weaning must be used with caution [61, 128].
9.4.5 Adaptive and Automated Weaning Systems (AWS)
While transition to pressure support or T-piece has traditionally been used, more recently “adaptive modes” have been advocated for weaning. Available “adaptive modes” are listed in Table 9.1.
Table 9.1
Available advanced “adaptive” modes used in weaning
Name | Company | Principles | Ref. |
---|---|---|---|
Mandatory minute ventilation (MMV) | Dräger Medical, Lübeck, Germany | Closed loop control of the mandatory breath rate while considering the patient’s spontaneous breath rate based on a prescribed minute ventilation | [132] |
SmartCare/PSTM | Dräger Medical, Lübeck, Germany | Closed loop control of pressure support based on monitoring of respiratory rate, tidal volume, and end-tidal carbon dioxide. Tests for extubation readiness using a 1-h spontaneous breath trial adaptive | [12] |
Adaptive support ventilation (ASV) | Hamilton Medical, Bonaduz, Switzerland | Closed loop control to maintain preset minimum minute ventilation by adjusting inspiratory pressure and mandatory breath rate on a breath-by-breath basis. Pressure support ventilation based on respiratory rate and tidal volume. Adjusts I:E ratio based on RC | [133] |
IntelliVent-ASV® | Hamilton Medical, Rhäzüns, Switzerland | Extension of ASV that uses closed loop control to adjust minute ventilation using end-tidal carbon dioxide and oxygenation by adjusting PEEP and inspired O2 concentration. Conducts automated SBT | [134] |
Automode | Siemens, Solna, Sweden | PC, PRVC, or VC switches to a support mode such as (either VS or PS ventilation) on detection of patient-initiated ventilation of two consecutive breaths | [135] |
Neurally adjusted ventilatory assist | Maquet, Solna, Sweden | Partial ventilatory support proportional to inspiratory diaphragmatic electrical activity measured via an esophageal catheter | [136] |
Proportional assist ventilation (PAV) | The University of Manitoba, Canada | Adjusts airway pressure based on measurement of compliance and resistance throughout the inspiratory cycle to maintain an clinician selected % degree of support | [137] |
Proportional pressure support (PPS) | Dräger Medical, Lübeck, Germany | Germany pressure support is provided proportionately to changes in airway resistance and lung compliance. PPS is based on the PAV algorithm | [137] |
Mandatory rate ventilation (MRV) | Taema-Horus Ventilator® Air Liquide, France | Closed loop control to titrate pressure support based on a set respiratory rate target | [138] |
The first adaptive modes with feedback were volume-targeted pressure control modes such as “pressure-regulated volume control” which titrated pressures to achieve a TV. Subsequent iterations targeted MV. Adaptive support ventilation (ASV) is a partially automated mode available from Hamilton Medical. ASV targets minute ventilation with an algorithm using the “Otis equation,” to target the least work of breathing. Studies comparing ASV weaning to standard care found that ASV could shorten weaning in post-cardiac surgery or chronic lung disease patient. When ASV was compared to assist control in patients with ARDS, no differences were found [133]. Of concern is that ASV allows TV of up to 22 mL/kg and in ARDS delivered median tidal volumes greater than the recommended 8 mL/kg [55, 133–135, 139, 140].
The most recent fully AWS incorporates feedback loops that titrate to ventilation and oxygenation targets and provides interesting opportunities. Two AWS are currently available the SmartCare® from Dräger Medical and IntelliVent from Hamilton Medical [141, 142].
While they differ slightly, the principles of the AWS are to establish ventilation through titration of adaptive ventilation: when stable, the sequential application of a SBT and then if successful conversion to titrated pressure support weaning. An early study of SmartCare ® found that closed-loop weaning resulted in more rapid weaning, whereas a similar study carried out in an extremely well-staffed unit demonstrated no benefit [12, 142]. Differences in the nursing intensity and clinical governance may be explain this discrepancy. This development highlights two possibilities:
Firstly, a more consistent weaning system, which if calibrated correctly, could provide rapid weaning.
Compared to nonautomated weaning methods, these systems demonstrated positive advantages in weaning time, time to successful extubation, and possibly assisted in reducing ICU length of stay and the number of patients requiring prolonged MV [54, 143] However, most patients studied received relatively short-term ventilation after surgery or ventilation for exacerbation of COPD. Clinical evidence from patients who have suffered ARDS is scarce [144, 145].
Secondly, when skilled personnel are scarce, equivalent outcomes may be achieved with less clinician input. Adaptive weaning systems follow a computerized protocol and as such could be viewed as being identical to a “manual” weaning protocols, with more assured compliance. If “weaning protocols” are non-inferior to standard care, an automated form of these should also demonstrate at least equivalent outcomes.
While the automated weaning systems show promise and some evidence of efficacy, proprietary systems are generally available only on a single manufacturer’s ventilator, and for multiple reasons, these systems have not been widely adopted [54].
9.5 Summary
Weaning patients with ARDS from ventilatory support present a challenge, with patient presenting disease, comorbidity, and severity of the ARDS all influencing the success rate. Most knowledge in the area is derived from trials in mixed populations or excluded ARDS patient; the evidence supporting the use of specific techniques is very rare. General attention to nutrition, physiotherapy, mobilization, and psychological support is pragmatically useful. Automated systems offer some promise, but clinical evidence of benefit in patients who have suffered from ARDS remains scarce.
References
1.
Bellani G, Laffey JG, Pham T, Fan E, Brochard L, Esteban A et al (2016) Epidemiology, patterns of care, and mortality for patients with acute respiratory distress syndrome in intensive care units in 50 countries. JAMA 315(8):788–800PubMed
2.
Cox CE, Carson SS, Govert JA, Chelluri L, Sanders GD (2007) An economic evaluation of prolonged mechanical ventilation. Crit Care Med 35(8):1918–1927PubMedPubMedCentral
3.
Herridge MS, Tansey CM, Matté A, Tomlinson G, Diaz-Granados N, Cooper A et al (2011) Functional disability 5 years after acute respiratory distress syndrome. N Engl J Med 364(14):1293–1304PubMed
4.
Boles JM, Bion J, Connors A, Herridge M, Marsh B, Melot C et al (2007) Weaning from mechanical ventilation. Eur Respir J 29(5):1033–1056PubMed
5.
Ambrosino N, Gabbrielli L (2010) The difficult-to-wean patient. Exp Rev Respir Med 4(5):685–692
6.
Dhanireddy S, Altemeier WA, Matute-Bello G, O’Mahony DS, Glenny RW, Martin TR et al (2006) Mechanical ventilation induces inflammation, lung injury, and extra-pulmonary organ dysfunction in experimental pneumonia. Lab Investig 86(8):790–799PubMed
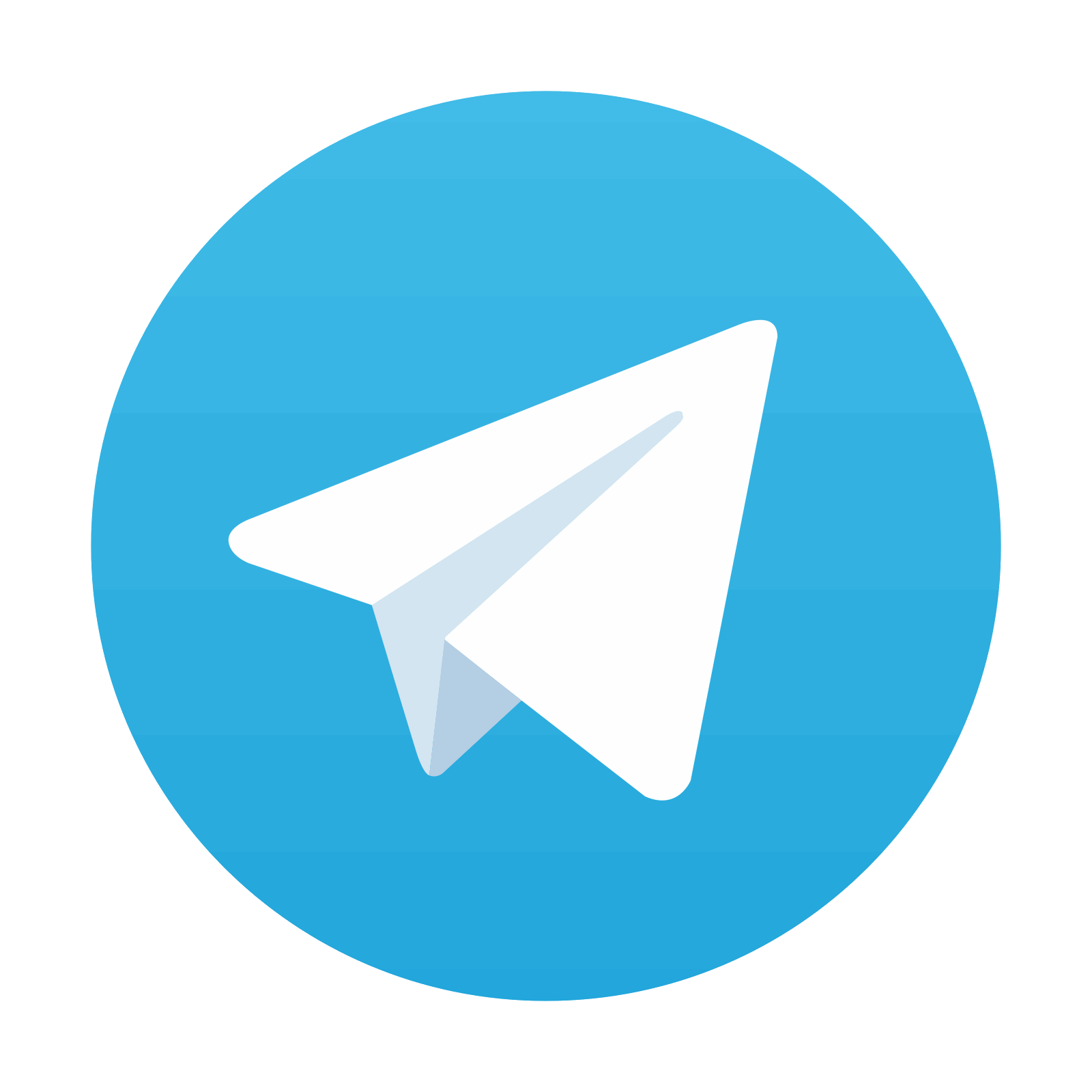
Stay updated, free articles. Join our Telegram channel

Full access? Get Clinical Tree
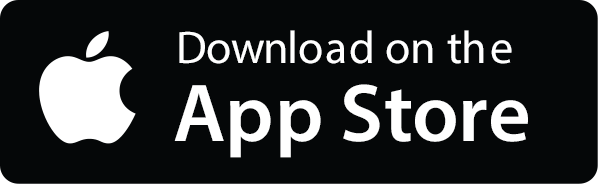
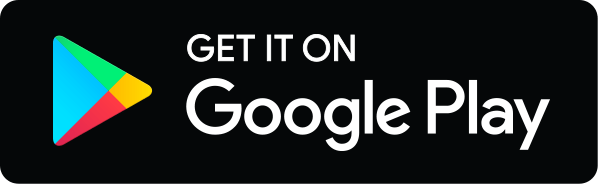