Ventilation Strategies in COPD, Asthma, and Pulmonary Arterial Hypertension
BACKGROUND
Chronic obstructive pulmonary disease (COPD), asthma, and pulmonary arterial hypertension (PAH) are diseases that are commonly encountered in an emergency department (ED) setting. It is estimated that 1.5 million patients visit the ED in the United States each year for COPD exacerbations, 1.75 million for acute asthma, and 200,000 for PAH.1–3 The appropriate initiation and management of emergent ventilatory support in these patients requires a nuanced understanding of the pathophysiology of these diseases.
DISEASE PATHOPHYSIOLOGY
Severe airflow obstruction is typically encountered in asthma and COPD, the latter of which includes both chronic bronchitis and emphysema. In both asthma and chronic bronchitis, the central pathologic events include inflammation, increased mucus (due to both hypersecretion and inflammation), and airway caliber reduction due to the combination of these processes. Airways in asthma and chronic bronchitis also suffer from decreased compliance, or compromised airway caliber expansion during spontaneous inspiration or positive pressure ventilation. The consequence of these pathologic effects is a limitation of airflow during both inspiration and expiration, both of which are important considerations when initiating mechanical ventilation in these patients.
The pathology of emphysema is different, even while the resulting expiratory airflow limitation can be similarly extreme. Emphysema is characterized by a loss of parenchymal lung elastic tissue, and thus a loss of the normal elastic recoil forces that hold open the smaller conducting airways. The result is that conducting airways tend to collapse with normal expiration; in marked contrast to airways in asthma, conducting airways in emphysema have greater compliance, and this airflow obstruction can magnify with increased expiratory effort during spontaneous breathing. Appreciating these distinct disease processes is critically important when considering mechanical ventilation for these patients.
Another important pathologic feature present in all patients with acute airflow obstruction is an increase in the work of breathing because of the greater airway resistance in these diseases; left untreated, a sustained increase in work of breathing can quickly lead to respiratory muscle fatigue and failure.
PAH has diverse pathologic causes, but a number of characteristics are common to all etiologies of this disease. These include an already-increased pulmonary arterial resistance and an associated increase in right ventricular afterload. Increases in pulmonary arterial resistance are seen with hypoxemia, hypercapnia (with magnified response to the combination of both), as well as with significant increases or decreases in lung volume because of lung parenchymal compression and overdistension of the pulmonary vascular beds, respectively.
VENTILATION STRATEGIES IN COPD AND ASTHMA
Patients who present with exacerbations of obstructive airway disease have an acute or chronic increase in airway resistance and an associated increase in work of breathing. This process heightens the risk of respiratory muscle fatigue and decompensation, which can compound already inadequate ventilation and increase the risk of respiratory failure. The application of assisted ventilation, by either noninvasive or invasive modes, can help reduce a patient’s work of breathing. The underlying increased airway resistance persists, however, and can be challenging to manage. Comprehensive therapy therefore combines support of respiratory mechanics (assistance with ventilation as well as reduction of work of breathing) with administration of bronchodilator and anti-inflammatory medications.
Noninvasive Ventilation Support
Noninvasive positive pressure ventilation (NIPPV) can be an appropriate first-line adjunct to medical therapy in the management of obstructive airway disease. When properly applied, NIPPV can preclude the need for invasive mechanical ventilation and its associated risks of trauma (direct, mechanical trauma or barotrauma), infection, adverse effects of sedation, increased length of hospital stay, and difficulty or failure to wean from the ventilator.4
The benefits of NIPPV assistance in respiratory distress from COPD are significant and well documented. A 1995 multicenter randomized control trial (RCT) of 85 patients with COPD compared NIPPV with standard treatment and demonstrated a decreased rate of intubation (26% vs. 74%), frequency of complications (16% vs. 48%), length of hospital stay (23 vs. 35 days), and inpatient mortality rates (9% vs. 29%).5 A MEDLINE and EMBASE review of similarly designed RCTs from 1968 to 2006 confirmed a consistent significant reduction in the risk of intubation (65%), in-hospital mortality (55%), and length of hospitalization (1.9 days) when NIPPV was used for acute exacerbation of COPD.6 A 2003 Cochrane systemic review meta-analysis of eight RCTs evaluating the use of NIPPV as an adjunct to usual medical care in COPD exacerbations similarly demonstrated a lower mortality risk (RR 0.41), shorter hospital stays (−3.24 days), and greater improvements at 1 hour in pH (+0.03), PaCO2 (−0.04 kPa), and respiratory rate (−3.08 breaths per minute).7 While the benefits of noninvasive ventilation are well established in COPD, its efficacy is less well established, and its use more controversial, in the setting of status asthmaticus.8
The decision to undertake a trial of noninvasive ventilation rather than proceed directly to intubation and mechanical ventilation is largely based on clinical judgment. Studies show that the majority of patients with asthma, even those with some degree of hypercapnia, can be successfully managed noninvasively.9,10 Similarly, in an RCT of 49 patients who failed standard ED medical therapy for COPD exacerbations, the use of NIPPV as a rescue therapy averted the need for intubation in 48%. Factors supporting the decision to initiate invasive ventilation in both COPD and asthma include refractory hypoxemia, severe hypercapnia (PaCO2 > 60 mm Hg), significant acidosis (pH < 7.25), and the inability to tolerate noninvasive ventilation, whether due to unsustainable dyspnea with increased respiratory frequency or altered mental status. Certainly, failure of a noninvasive ventilation trial or cardiorespiratory arrest calls for immediate intubation.11,12
Mechanical Ventilation Support
If the decision is made to initiate invasive ventilatory support, either assist–control ventilation or synchronized intermittent mandatory ventilation, using volume or pressure cycling, may be used; no one mode of ventilation has been found consistently superior in the treatment of these conditions. There are, however, a number of ventilator parameters that should be carefully selected and continuously evaluated when managing these patients.
The initiation of mechanical ventilation involves a substantial shift in the physiology of ventilation, with multiple, immediately relevant effects. The principal physiologic differences for the clinician to remember are that:
- Positive pressure (with or without patient assistance) is now being used to achieve lung inflation.
- Inspiratory peak pressures from positive pressure ventilation will necessarily be increased in the context of the high airflow resistance caused by airway inflammation and narrowing and/or dynamic collapse.
- The time needed for complete exhalation will be substantially increased due to the marked airflow obstruction, and if adequate (prolonged) expiratory times are not allowed by the ventilator parameters, lung gas volumes will increase (“air trapping” and “hyperinflation”) and intrapulmonary and intrathoracic pressures will rise (“induced, or intrinsic, positive end-expiratory pressure [PEEP]”).
By far, the most potentially harmful of these adverse physiologic effects is the production of higher intrathoracic pressures and induced PEEP, which can result in both pulmonary and cardiac compromise. Pulmonary effects include barotrauma (i.e., pneumothorax, interstitial emphysema with pneumomediastinum) and parenchymal ventilator–induced lung injury. In addition, increased intrathoracic pressure increases thoracic volume, which results in chest wall distension (and a less compliant chest wall) and a greater ventilator workload for any patient assisting with mechanical ventilation (i.e., taking spontaneous breaths in addition to the set mechanical respiratory rate). This increased work of breathing can raise metabolic demand, further destabilizing an already compromised patient. Cardiac effects include reduced biventricular preload and increased pulmonary artery resistance (increased RV afterload), with resulting diminished cardiac output. Given this array of potential issues, the goal when initiating mechanical ventilation in patients with severe airflow obstruction is to employ techniques and parameters that avoid or at least limit pulmonary and cardiac compromise.
Minimization of induced intrathoracic air trapping and intrinsic PEEP is achieved by using either a shorter inspiratory time (which, for a fixed respiratory rate, will produce a lengthened I:E ratio) or a lower respiratory rate (which, with a fixed inspiratory time, will also produce a lengthened I:E ratio). As inspiratory time is shortened, ventilator inspiratory flow rate will necessarily be increased in volume-cycle ventilation modes to achieve the specified tidal volume, often into the range of 80–100 L/min.
As a working example of the adjustment of ventilator parameters to achieve a lengthened I:E ratio and lessened barotrauma risk, consider the following example in volume-cycled ventilation:
A.Initial ventilator settings:
- Ventilator mode: volume cycle assist control ventilation
- Ventilator rate: 20 (i.e., a 3-second breath cycle)
- Inspiratory time: 1 second (with resulting I:E ratio of 1:2)
B.Adjusted ventilator settings to avoid air trapping:
- Ventilator mode: volume cycle assist control ventilation
- Ventilator rate: 20
- Inspiratory time: 0.5 second (with resulting I:E ratio now increased to 1:5)
While different ventilator manufacturers use different default parameters to adjust the I:E ratio (i.e., either adjustment of the I:E ratio directly, or conversely, adjustment of inspiratory time to alter the dependent I:E ratio), the important points are the following: a) it is imperative to recognize the need for a lengthened I:E ratio for optimal control of ventilation in severe obstructive diseases, and b) careful monitoring and appropriate adjustment of the I:E ratio will help to avoid increments in intrinsic PEEP and resulting cardiopulmonary function compromise. Peak inspiratory pressures will necessarily increase with a shorter inspiration but will not contribute to patient injury.
It is important to understand the role of increased peak inspiratory pressures seen in severe airflow obstruction. While high inspiratory peak pressures have historically been thought to produce an increased risk for barotrauma, this has been proven false.13 To avoid air trapping and hyperinflation—by far the more harmful consequences of mechanical ventilation in these patients—ventilator settings that are invariably associated with higher peak inspiratory pressures (e.g., shorter inspiratory times with higher ventilator inspiratory flow rates) are reasonable and should be tolerated by the treating physician. In addition, these same physiological considerations also underlie the strong recommendation to not use pressure-control ventilation modes in acute severe airflow obstruction. Using pressure-limited ventilation modes in severe obstruction will produce very small, and likely much more variable tidal volumes and resulting minute ventilation, and thus should be avoided, particularly when higher peak pressures of volume-cycle modes are known to be tolerated well.
In contrast to peak pressures, high plateau pressures are known to be harmful and to be associated with barotrauma. While few studies have looked specifically at patients with COPD or asthma, lower plateau pressures (<30 cm H2O) have been shown to decrease the incidence of ALI and acute respiratory distress syndrome (ARDS) in mechanically ventilated patients.14,15 As in circumstances of acute lung injury, the delivery of smaller tidal volumes may also be necessary to achieve target plateau pressures and avoid traumatic complications in patients with COPD or asthma.16 A tidal volume of 6 to 8 mL/kg of ideal body weight, with further adjustments as needed, is a reasonable initial strategy. Lower tidal volumes, however, produce lower minute ventilation, which can be a physiologic confounder in the already hypercapnic patient. Increasing set ventilatory rates can counter the effect on MV of lower tidal volumes but will, in turn, accelerate air trapping. To minimize air trapping, a set respiratory rate of 10 to 14 breaths per minute is preferred. Significant air trapping is indicated by increasing levels of intrinsic PEEP and should be closely monitored and minimized where possible.17 Careful consideration of physiology and available ventilator support options is necessary to balance acceptable ventilation with the associated risks of mechanical ventilation in these patients; some degree of “permissive hypercapnia” —that is, allowing for an abnormal rise in PCO2 to enable minimization of delivered tidal volumes and breaths per minute—is often required to avoid exacerbation of lung injury and barotrauma.
While the addition of PEEP is known to contribute to lung hyperinflation in patients with obstructive airway disease, there are certain circumstances in which added PEEP can help relieve airflow obstruction. This is particularly true in patients in whom airflow obstruction and respiratory failure are associated with increased airway compliance (e.g., emphysema) as opposed to decreased compliance (e.g., acute asthma flare or bronchiolitis). When intrinsic PEEP is present in these patients, the application of added PEEP can provide a “stenting” effect to maintain airway caliber during exhalation, which can improve overall airflow obstruction, assist the patient’s ability to trigger subsequent breaths, and minimize the associated hypercapnia, increased work of breathing, and hemodynamic compromise. If this technique is used, additional extrinsic PEEP should be set to a level that is approximately 75% of the intrinsic PEEP.18–20
The optimal fraction of inspired oxygen (FiO2) provided to the mechanically ventilated patient with COPD or asthma has not been clearly determined. While low pO2 (>60) and Hgb saturations (>90%) may be acceptable in ARDS, the avoidance of additional cardiopulmonary compromise from hypoxemia may require a higher FiO2 in this setting.
Helium can be mixed with oxygen to form a gas with a lower density than ambient air or than ambient air mixed with oxygen. Termed “heliox,” and generally available in helium:oxygen ratios of 80:20 or 70:30, this gas can decrease airway resistance by increasing the proportion of laminar to turbulent flow in the airways, thereby improving airflow and decreasing a patient’s work of breathing. Heliox can be delivered either noninvasively or with mechanical ventilation. It should be noted, however, that there are limited data supporting the routine use of heliox in obstructive airway diseases.21 While there are minimal adverse effects associated with the use of heliox, the physician should be aware that ventilator adjustments might be necessary to correct for changes in flow of this less dense gas. The use of heliox mixtures can produce erroneous volume delivery measurements in some mechanical ventilators, with a resulting need to use pressure-cycle rather than volume-cycle ventilation modes. It is important to determine the specific needs of the ventilators used and to make any necessary adjustments, before using heliox mixtures for ventilator support.
VENTILATION STRATEGIES IN PULMONARY ARTERIAL HYPERTENSION
PAH is a significant contributor to right ventricular dysfunction.22–24 The challenges to mechanical ventilation in patients with PAH are significant, as positive pressure ventilation can exert a destabilizing hemodynamic impact on an already stressed right heart. Positive pressure ventilation increases intrathoracic pressures, including right atrial pressures, which in turn decreases venous return to the right ventricle. This drop in right ventricular preload decreases right ventricular output, contributing to ventricular dysfunction. Compounding this process, as lung volumes increase with positive pressure ventilation and larger tidal volumes, alveolar distention leads to compression of the associated vessels, including the inferior vena cava. The resultant increase in pulmonary vascular resistance (PVR) is reflected as an increase in right ventricular afterload, which exacerbates existing PAH and tricuspid regurgitation, and contributes to further right ventricular dysfunction.25–27
Both hypoxemia and hypercapnia/acidemia independently increase PVR, and the combination of the two has a greater effect on PVR than either alone. The choice of ventilation parameters thus needs to optimize oxygenation and ventilation as much as can be safely achieved without adding greater stress on RV function.
Initial ventilation management strategies for patients with PAH should focus on limiting intrathoracic pressures. High applied PEEP and large tidal volumes should be avoided if possible. Lower tidal volumes (4 to 6 mL/kg ideal body weight) will help limit plateau pressures while still allowing for adequate oxygenation and ventilation. Similarly, lower levels of applied PEEP, typically to remain <15 cm H2O, will limit the detrimental effects of high plateau and intrathoracic pressures. Careful monitoring is required to avoid the problem of mechanical ventilation-induced increased intrinsic PEEP, as can be produced in severe airflow obstruction (e.g., asthma and COPD). Oxygen saturation should be maintained at or above 92%. When employing a low tidal volume ventilation approach, a higher set respiratory rate can help minimize hypercapnia and acidemia; this is unlikely to create physiologic problems so long as there is no concurrent severe airflow obstruction present. If airflow obstruction is present concurrently with PAH, then the ventilator management guidelines outlined earlier in this chapter will also need to be employed.28
CONCLUSION
Optimal support of severe COPD and asthma requires recognition of the central and critically important role of increased airway resistance in these diseases. NIPPV can assist significantly in reducing the work of breathing and helping to alleviate respiratory failure. Intubation and mechanical ventilation are typically necessary when progressive respiratory muscle fatigue and/or worsening airflow obstruction produce hypercapnic ventilatory decompensation. Critical parameters to consider for achieving optimal mechanical ventilation support in these patients include
- Shorter inspiratory times and prolonged expiratory times, with resulting longer I:E ratios
- Acceptance of higher peak inspiratory airway pressures to achieve shortened inspiratory times
- Reduced tidal volumes to avoid higher inspiratory plateau pressures
- Avoidance of increases in trapped gas, hyperinflation, and the induction of increased intrinsic PEEP
- Application of PEEP in select patients with emphysema to assist in the relief of airflow obstruction resulting from dynamic expiratory airway compression
Mechanical ventilator support of patients with significant PAH requires taking steps to minimize physiologic stressors that can increase pulmonary arterial resistance. These include
- Avoidance of hypercapnia and hypoxemia
- Avoidance of high tidal volumes, as these can contribute to increased PVR
While these diseases can be associated with complex clinical conditions, their optimal ventilatory management is based on well-understood pathophysiology. The considerations and approaches outlined in this chapter provide a basis for the initial aspects of ventilatory care, with the goal of improving outcomes in ED and ICU care (Table 9.1).
TABLE 9.1 Ventilation Strategies for COPD, Asthma, and Pulmonary Hypertension
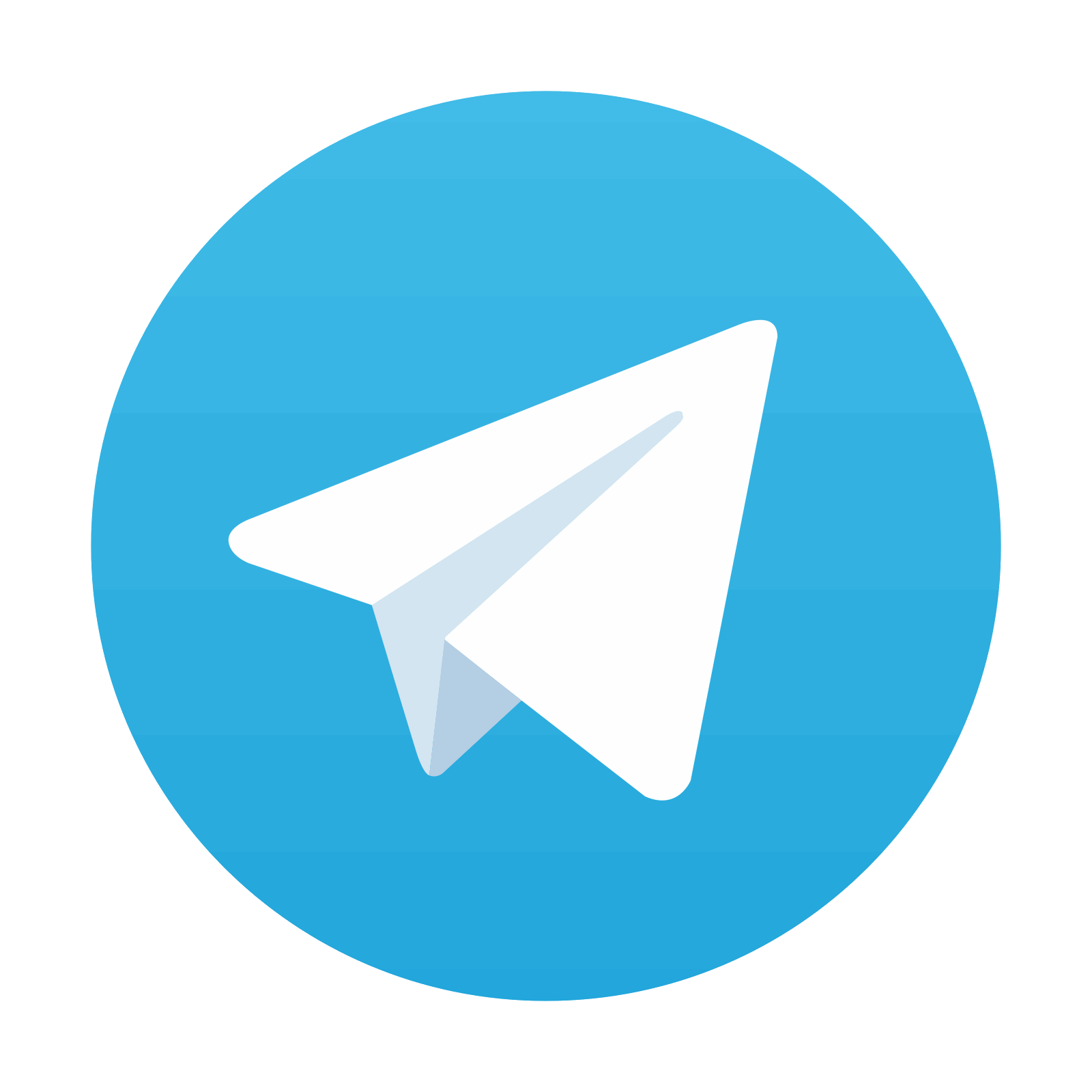
Stay updated, free articles. Join our Telegram channel

Full access? Get Clinical Tree
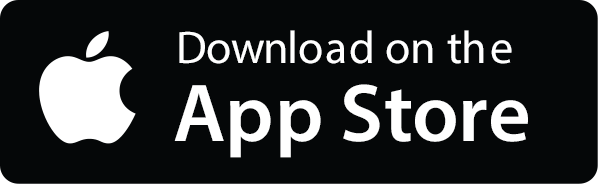
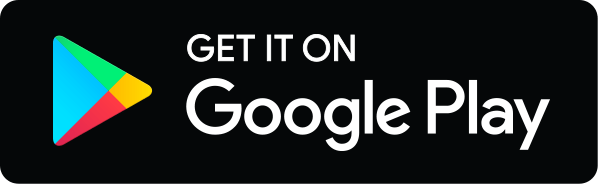