KEYWORDS
vaporizer types
vaporizer safety features
Vaporizers are one of our interfaces with the anesthesia machine. We continually adjust them throughout each case we do. But do we really know what we are doing each time we move the dial?
Vaporizers are very complicated on the inside, much more than they may look to us. Inside each one there are things going on that pertain to gas laws, specific heat, vapor pressure, and all that stuff from chemistry we thought we would never see again. They are also categorized by certain qualities that may not make sense to us at first.
In this chapter, we will discuss how a vaporizer works, what each phrase in its classification means, and the hazards of vaporizers.
CHEMISTRY AND PHYSICS
Think about a can of gasoline. When it is open, you can smell the fumes emanating from the can. Another name for the fumes would be vapor. The fumes we smell are not a “gas” (three-letter word, not the short word for gasoline). A true “gas” is a chemical that has already reached its boiling point in the setting in which it finds itself. Oxygen, carbon dioxide, nitrogen, and so on are true “gases” in our environment because they exist at a temperature above their boiling points. That is why it is incorrect (but nevertheless common) to refer to inhalational agents as “gas” because they are not true gases at the temperature of an operating room (OR); they exist in a state below their boiling points. (Desflurane at room temperature is almost at its boiling point, however). The only commonly used “gases” found in an anesthesia machine are oxygen, air, and nitrous oxide.
Now think about a can of gasoline on a hot day. Gas cans nowadays are made out of plastic, not metal like in the past. On a hot day, a full can of gasoline can actually expand and deform itself. This is because the gasoline fumes, or vapors, are exerting a pressure against the sides of the gas can. This is called, simply enough, vapor pressure. This is an example of how above every liquid in a closed container, there exists a vapor pressure. In this situation, the vapor pressure is enough to distend the sides of the plastic can.
The molecules of hydrocarbons that make up what we call gasoline are leaving the surface of the liquid and floating up into the above space while at the same time molecules of gasoline are settling back down into the liquid from the vapor in the space above. The liquid and vapor are in equilibrium with each other.
A liquid and a vapor in an enclosed space will always find its own equilibrium (Figure 6-1). The amount of substance that is in liquid versus vapor phase in an enclosed container varies with temperature. For instance, on a day when it’s 90°F, our can of gasoline has more molecules in a vapor phase than it would at 70°F (and therefore has a higher vapor pressure, thus distending the closed can). At 30°F, there would be less gasoline in a vapor phase still. There is the same amount of molecules of gasoline at all three temperatures, but the vapor pressure changes with changes in temperature because as the temperature increases, there is a shift to relatively more gasoline existing as a vapor than at lower temperatures.
Figure 6-1 Notice at 90°F, there are more molecules in vapor form than at 70°F, and at 30°F, there are less molecules in vapor form than at 70°F. Also notice how the gasoline can at 90°F has expanded its walls—because more molecules are in vapor form, the vapor pressure is higher.
So what does all this have to do with turning on the sevoflurane? There are things inside vaporizers that deal with the above concepts. Even the material that vaporizers are made of is important in ensuring an accurate delivery of what you dial in; more on all this to follow.
CLASSIFICATION OF VAPORIZERS
The modern vaporizer is classified as follows: variable bypass, agent specific, flow over, temperature compensated, and out of circuit. Each one of these phrases means something that you need to know about vaporizers (Figure 6-2).
Figure 6-2 Schematic of agent-specific variable-bypass vaporizers. (A) Draeger Vapor 19.n. (B) Datex-Ohmeda Tec 7. (Reproduced with permission from Morgan GE, Mikhail MS, Murray MJ. Clinical Anesthesiology. 4th ed. New York, NY: McGraw-Hill; 2006. Figure 4-19A, B.)
Variable Bypass
When you adjust the knob of a vaporizer, you are varying how much of your fresh gas flow (FGF) (that you have dialed in from your flowmeters) goes into the vapor chamber versus how much bypasses the vapor chamber, thereby controlling how much of your FGF is exposed to your inhalational agent (and picks up the anesthetic vapor) and how much of your FGF goes straight through the vaporizer without picking up any vapor.
Agent Specific
Vaporizers for the past several decades have been designed to be agent specific, meaning that a certain vaporizer is constructed and calibrated to deliver a certain anesthetic agent based on qualities such as its vapor pressure, at a range of ambient room temperatures found in ORs, throughout a wide range of FGF rates (from 250 cc/min to 15 L/min). We all know that vaporizers are labeled and colored a certain color for each agent. In fact, we probably rely more on the vaporizer color than reading the label.
Up until the early 1990s, there was no safeguard to ensure that the correct liquid was poured into the correct vaporizer. Vaporizers back then had a simple screw-on lid that you opened and filled the vaporizer through. If you weren’t paying attention or were in a room with the lights dimmed, it would have been easy to mistakenly pour the incorrect agent into the incorrect vaporizer (especially with halothane and enflurane—one was colored orange-red and the other reddish orange!). We will discuss the implications of misfilling a vaporizer later on in the chapter.
Of course, now there are various fail-safes to guard against misfilling a vaporizer. We are all familiar with the collars that are on bottles of liquid agent. The collars have tabs that will only allow the correct attachment that will fit the refilling inlet of the vaporizer in question. Draeger uses a funnel-type system, and Ohmeda uses a “key” siphon system; both systems are agent specific and manufacturer specific.
Flow Over
When you turn the vaporizer control knob and vary the amount of FGF that bypasses the vapor chamber, a portion of the FGF is directed into the vapor chamber. There, it flows over the top of the liquid agent, picking up the vapor that is above the liquid.
Older vaporizers were called “bubble through” because the FGF would actually bubble through the liquid agent from the bottom of the chamber, similar to blowing bubbles in a glass with a drinking straw. The bubbles with their great amount of surface area would pick up molecules of the anesthetic agent in that manner.
It is easy to see by the size of a vaporizer that there is not much surface area for the liquid anesthetics in their chamber. There might be enough surface area of the liquid agent for enough vapor to be picked up at low FGF, but how about when you have sevoflurane at 3% and 6 L/min FGF? There is definitely not enough surface area for accurate delivery at such a rate. Enter the wick.
Inside vaporizers there are wicks, analogous to lantern wicks, along the sides of the vaporizing chamber that absorb the liquid agent. By capillary action, the liquid is spread out all over the wick. This greatly increases the surface area of the liquid, so more vapor is picked up by our FGF. The wicks make the sides of the vaporizing chamber a surface area for the liquid in addition to merely the top of the liquid in the bottom of the vaporizer.
Temperature Compensation
As we saw earlier by observing our gasoline can at various temperatures, vapor pressure is dependent on temperature. The higher the ambient temperature, the more the vapor a liquid will have above it in a closed container and vice versa.
So what happens in the OR when the room temperature may be high in a pediatric room and cold in the heart room? Even in the range of temperatures seen in ORs, the amount of vapor above our liquid anesthetic agent in the vaporizer will differ from room to room because of temperature differences. There will be more agent in the vapor phase in the pediatric room versus the heart room. This would influence accurate delivery of how much agent we have dialed in if there were no compensation for temperature differences.
In addition to differences in room temperature, the vaporizer needs to compensate for changes in temperature inside the vaporizing chamber as a direct result of the vaporizing process. Vaporization of a liquid takes energy. That energy comes from the heat of the liquid that is vaporizing. This is called the latent heat of vaporization. So as we provide an anesthetic, the temperature of our liquid agent is decreasing because it is losing heat in the process of vapor formation.
Therefore, we have two potential causes of temperature problems in vaporizers: ambient temperature changes and loss of heat from vaporization. Now let’s say that our vaporizers were designed to work at 25°C. If the room was warmer, the output of anesthetic from the vaporizer would be more than we have dialed in; conversely, if the room was colder, our agent output would be less than what we want. On top of that, we are also losing heat from the process of vaporization of our liquid agent.
Fortunately, there are means of temperature compensation. There is a device inside a vaporizer that changes shape or length with changes in ambient temperature. Think about junior high school science class. You may have studied about how a thermostat on the wall has a bimetallic strip inside it that changes shape or length in response to changes in room temperature. That is how a thermostat knows when to turn on or shut off.
Some vaporizers have a rod that changes length in response to temperature changes and is precisely calibrated so that it will allow more or less FGF into the vapor chamber, automatically as needed. Other manufacturers have a precisely calibrated bimetallic strip that either blocks FGF into the vaporizing chamber or allows more FGF into the chamber based on ambient temperature. This keeps the output of the vaporizer the same whether you are using it in the pediatric room or the heart room (Figure 6-3).
Figure 6-3 The bimetallic strip at 68°F lets more of the fresh gas flow enter the vapor chamber compared with the bimetallic strip at 75°F, where more agent is in the vapor phase. This calibrated strip keeps the vaporizer output the same for different room temperatures.
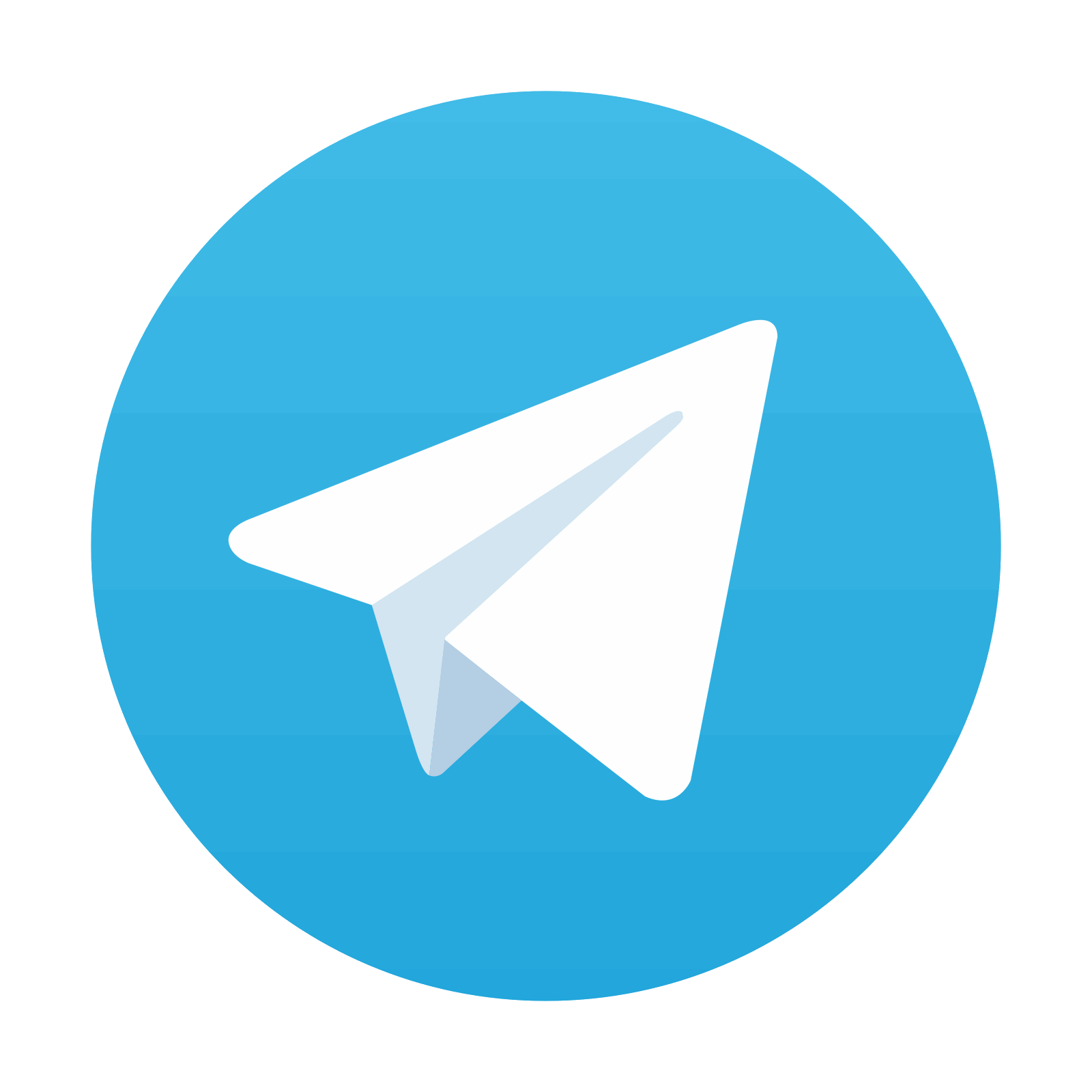
Stay updated, free articles. Join our Telegram channel

Full access? Get Clinical Tree
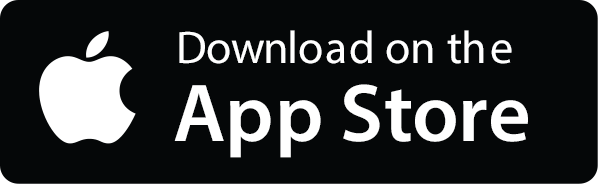
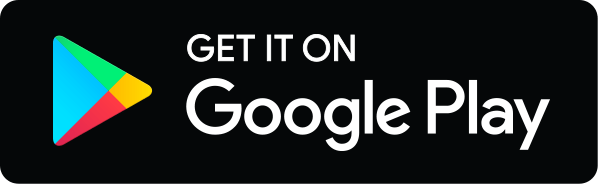