Decompression sickness and arterial gas embolism, collectively known as decompression illness (DCI), are rare but serious afflictions that can result from compressed gas diving exposures. Risk is primarily determined by the pressure-time profile but is influenced by several factors. DCI can present idiosyncratically but with a wide range of neurologic symptoms. Examination is critical for assessment in the absence of diagnostic indicators. Many conditions must be considered in the differential diagnosis. High-fraction oxygen breathing provides first aid but definitive treatment of DCI is hyperbaric oxygen.
Key points
- •
Decompression sickness (DCS) is a disease resulting from an ascent profile not allowing the orderly elimination of excess gas that was accumulated in tissues during exposure to elevated pressure.
- •
Decompression sickness (DCS) can present idiosyncratically, affecting a wide range of systems with a variable degree of insult. Masking of important symptoms by chief complaint is possible.
- •
Arterial gas embolism (AGE) is a disease of frank gas in the arterial systemic circulation following a reduction of ambient pressure so rapid that expanding gases cause pulmonary tissue rupture.
- •
The first aid for decompression illness (collectively, DCS and AGE) is high fraction oxygen; the definitive treatment is hyperbaric oxygen therapy.
- •
There are currently no diagnostic tests to confirm decompression sickness.
Introduction
Diving is a popular recreational pastime, as well as an activity with numerous applications in the scientific, commercial, military, and exploration realms. Although diving can be done safely, the underwater environment is unforgiving. Problems may arise during a dive due to insufficient medical or physical fitness, improper use of equipment, or inadequate management of the high-pressure environment.
Decompression illness (DCI) is a term used to encompass injuries due to arterial gas embolism (AGE) and decompression sickness (DCS). AGE typically results from pulmonary barotrauma-induced damage to the alveolar wall and introduction of gas into the systemic arterial circulation. DCS, colloquially known as the bends, results from the uncontrolled release of gas from tissues during or after surfacing with inadequate time for equilibration (decompression).
Introduction
Diving is a popular recreational pastime, as well as an activity with numerous applications in the scientific, commercial, military, and exploration realms. Although diving can be done safely, the underwater environment is unforgiving. Problems may arise during a dive due to insufficient medical or physical fitness, improper use of equipment, or inadequate management of the high-pressure environment.
Decompression illness (DCI) is a term used to encompass injuries due to arterial gas embolism (AGE) and decompression sickness (DCS). AGE typically results from pulmonary barotrauma-induced damage to the alveolar wall and introduction of gas into the systemic arterial circulation. DCS, colloquially known as the bends, results from the uncontrolled release of gas from tissues during or after surfacing with inadequate time for equilibration (decompression).
Diving physics
The concentration, or tension, of dissolved inert gas within body tissues is a function of ambient pressure. Inert gases normally exist in equilibrium with the ambient environment, effectively a saturated state. When pressure differences (gradients) are created, molecules flow from the area of higher to lower concentration until equilibrium is re-established.
The pressure range of the diving environment is much greater than the pressure range of the air environment. The pressure exerted at sea level by the entire 100 km (62 mi) atmospheric column of gas is 1 atm absolute (ATA), equal to 101.3 kPa or 14.7 psi. In contrast, water pressure increases by 1 atm for every 10 m (33 ft) of seawater and for every 10.4 m (34 ft) of freshwater ( Fig. 1 ).
The lungs play a primary role in gas uptake and elimination and, ultimately, decompression stress. When exposed to increased pressure underwater, the gas in the lungs is compressed. This creates an inflow gradient from concentrated lung gas to the bloodstream and, subsequently, from the bloodstream into tissues as they are perfused. Tissues take up inert gas until saturation is achieved. At the point of saturation, staying longer does not further increase the subsequent decompression obligation.
Predicting gas uptake and elimination
Gas uptake and elimination generally follows roughly exponential patterns. The technology is not yet available to measure tissue status directly, so the norm is to rely on mathematical algorithms to predict gas exchange. A range of half-times are used to represent different tissue characteristics; not as exact referents but as mathematical constructs to collectively estimate what happens throughout the body.
The fastest tissues are the lungs, which achieve equilibrium almost instantly. Blood is another extremely fast tissue, followed by the brain. The slowest tissues are those that are relatively poorly perfused, such as ligaments and cartilage, or those that have a high capacity for inert gas uptake, such as fat in poorly perfused areas.
Consider a diver instantly displaced from the surface to a fixed depth as an example. A 5-minute half-time would represent a fast tissue, computed to take up sufficient inert gas uptake to eliminate half of the difference produced by the pressure gradient (50%) in the first 5 minutes. This would be the steepest portion of the uptake curve. The second 5-minute period would eliminate half of the remaining difference (25%). The third 5-minute period would eliminate half of the remaining difference (12.5%) and so on. At the other extreme, a 500-minute half-time could be computed to represent an extremely slow tissue. With no additional influences on the process, equilibration is expected to be nearly achieved after a period equal to about 6 half-times for any given tissue ( Fig. 2 ).
Most dives do not last long enough for the diver to reach saturation; these are known as bounce dives. During such exposures, the inflow gradient exists at least through the descent and bottom phase of the dive, which causes continued uptake of inert gases, certainly in slow compartments and probably in intermediate compartments. When the diver ascends, the ambient pressure starts to drop and the gradient begins to reverse, first in fast compartments and then in progressively slower compartments.
When the tissue tension of a gas exceeds the ambient partial pressure of that gas, the tissue is supersaturated. This will be the case of most tissues during and after surfacing. If the degree of supersaturation is modest, equilibration from peripheral tissues into the blood, then lungs, and then atmosphere, will be orderly. If the degree of supersaturation is too great, the elimination of inert gases becomes disorderly, gas phase (bubbles) can form, and DCS may develop.
Bubble formation does not always cause problems but the greater the supersaturation the greater the likelihood that symptomatic DCS will occur. It is a misconception that bubbles forming after a dive are of no importance; however, it is also a misconception that bubbles equate to DCS. The formation of gas bubbles during decompression represents a stress greater than is optimal and may lead to DCS.
Controlling dive exposures
Dive computers have supplanted printed dive tables as the primary means of regulating dive profiles over the last 20 years. Dive computers provide more flexible guidance because they continuously assess the pressure-time profile and compute the status of a variety of hypothetical tissue compartments. Exposure limits or decompression obligations are adjusted based on whatever compartment is deemed most critical (effectively the controlling half-time) at any point in the ascent. This is useful because modern divers frequently follow complex descent-ascent profiles, relying on the dive computer to keep track of their state.
Dive computers provide guidance based on the primary determinants of decompression risk: the pressure-time profile and breathing gas. However, gas exchange is also influenced by the timing and intensity of exercise and thermal stressors during a dive, as well as by individual predispositions, none of which are assessed by dive computers in a meaningful way.
Symptoms of DCS may develop after dives conducted within decompression algorithm limits. Decompression algorithms predict outcomes but they do not guarantee them. That a dive is conducted within the limits does not make a DCS hit undeserved.
Developing decompression sickness
Although DCS is commonly thought of as a bubble disease, bubbles are probably only the gateway to a complex array of consequences. Bubbles can form in or reach a wide variety of tissues, and initiate biochemical cascades to produce secondary insults and effects. Vascular obstruction or interactions may stimulate platelet aggregation, leukocytes activation and aggregation, and fibrin deposition. Intravascular bubbles may also damage the capillary endothelium, possibly leading to increased permeability and tissue edema exacerbating the ischemia process. An increased release of cytokines and/or complement activation has also been demonstrated as possible contributing agents. The inflammatory cascade could play an important role in the pathophysiology of DCI. The variability in activation threshold of this inflammatory cascade might explain the differences between individuals in susceptibility to DCS. The inflammatory and coagulation cascades might also explain the failure of recompression treatment in some cases because, once activated, the resolution of bubbles will not immediately stop the response.
The mechanics of arterial gas embolism
Unlike DCS, which requires a period of gas uptake, AGE results from an acute decrease in ambient pressure in a tissue unable to accommodate. For example, a diver ascending rapidly from 10 m (2 ATA) to the surface (1 ATA) is faced with a 50% reduction from the bottom pressure. In accordance with Boyle’s law (P 1 V 1 = P 2 V 2 ; assuming constant temperature), an unventilated volume of gas would double during this excursion. Normally, the diver would breathe freely during a slow ascent and constantly re-equilibrate lung volume. If ventilation is not adequate, either due to breath-holding or a localized bronchial obstruction (eg, bronchospasm or mucus plug), an overexpansion injury (pulmonary barotrauma) can result. The maximum sustainable tissue elastic pressure in the alveoli is around 100 to 150 mm Hg (0.13–0.2 atm) over ambient. A pressure of 0.13 atm is found at a depth of 1.3 m (4 ft) in seawater. A rapid ascent with full lungs and an obstructed airway from this very shallow depth could result in pulmonary barotrauma. Tissue rupture will allow gas from the alveolar space to enter the pleural space (pneumothorax), the pulmonary interstitium, the mediastinum (pneumomediastinum), and/or the pulmonary capillaries. Bubbles entering the pulmonary capillaries can be transported into the systemic arterial circulation and may reach critical tissues, such as the brain or the spinal cord, to produce serious symptoms. The symptoms of AGE can be very similar to those of DCS, sometimes making them difficult to separate.
Incidence of decompression illness
The lowest DCI incidence rates have been reported in the scientific diving community at 0.324 per 10,000 person-dives. Divers Alert Network (DAN) estimates of DCI incidence rates in the recreational community range from 2.0 to 4.0 per 10,000 person-dives. The DCS incidence rate in commercial decompression diving has been reported to be as high as 35.3 per 10,000 person-dives. A more recent study of commercial diving DCS described incidence rates ranging from 1.4 to 10.3 per 10,000 person-dives, depending largely on the depth of dive operations.
Signs and symptoms
A variety of classification systems have been established for DCS. One common approach is to describe cases as type 1 or type 2.
Type 1 DCS is usually characterized by musculoskeletal pain and mild cutaneous symptoms. Common type 1 skin manifestations include itching and mild rashes (as distinct from a clear, mottled or marbled, and sometimes raised discoloration of the skin known as cutis marmorata [ Fig. 3 ] that may presage the development of the more serious type 2 symptoms). Less common but still associated with type 1 DCS is obstruction of the lymphatic system, which can result in swelling and localized pain in the tissues surrounding the lymph nodes.
Musculoskeletal manifestations of type 1 DCS are articular or periarticular pain. The joint pain usually has a gradual onset and presents as a deep dull ache. Pain intensity may range from mild to severe. The pain will typically be present at rest and may or may not be exacerbated by movement. Common locations include shoulders, elbows, wrists, hips, knees, and ankles.
Type 2 symptoms are considered more serious. They typically fall into 3 categories: neurologic, inner ear, and cardiopulmonary. Neurologic symptoms include numbness; paresthesia or tingling; muscle weakness; impaired gait, physical coordination or bladder control; paralysis; or change in mental status. Some neurologic symptoms are commonly described as constitutional, such as headache, lightheadedness, unexplained fatigue, malaise, nausea and/or vomiting, or anorexia. Inner ear symptoms include tinnitus, hearing loss, vertigo or dizziness, nausea, vomiting, and impaired balance. Cardiopulmonary symptoms, known as the chokes, include a dry cough, retrosternal pain, dyspnea, and sometimes pink-stained, frothy sputum. Cardiopulmonary involvement occurs when massive bubble loads obstruct a substantial proportion of the pulmonary vascular bed. Cardiopulmonary DCS usually follows highly provocative dive profiles with a significant omitted decompression. It is important to rule out immersion pulmonary edema, which can present like cardiopulmonary DCS.
Type 2 symptoms can develop slowly or quickly. A slow build can obscure the seriousness of the situation, allowing denial to persist. For example, fatigue and weakness may initially be easy to ignore. Less common symptoms, such as difficulty walking, urinating, hearing, or seeing (especially with quick onset) are more difficult to deny.
Other types of classification exist for DCS. For the first-line physician, differentiation between type 1 or type 2 DCS is somewhat unnecessary because DCS is a systemic disease that often involves multiple organs and, in any case, the final treatment approach will not differ that much.
The presentation of DCS is frequently idiosyncratic; that is, its typical pattern can be atypical. In some cases, an affected diver’s chief complaint may draw attention away from subtler but potentially more important symptoms. The following list ranks the initial manifestations of DCS, with frequency estimates for each :
- •
Pain, particularly near the joints (68%)
- •
Numbness or paresthesia (63%)
- •
Constitutional symptoms (41%)
- •
Dizziness or vertigo (19%)
- •
Motor weakness (19%)
- •
Cutaneous changes (10%)
- •
Impaired mental status (8%)
- •
Impaired coordination (8%)
- •
Muscle discomfort (7%)
- •
Pulmonary symptoms (6%)
- •
Bladder or bowel dysfunction (3%)
- •
Auditory symptoms (2%)
- •
Impaired consciousness (2%)
- •
Lymphatic involvement (2%)
- •
Compromised cardiovascular function (<1%).
Clinical manifestations are described by system in Table 1 .
System involved | Symptoms | Signs |
---|---|---|
Skin | Itching | Nonspecific skin rash, urticarial rash, well-organized mottling (cutis marmorata) |
Lymphatics | Localized pain in the region of lymph nodes | Localized skin and soft tissue swelling (lymphedema) |
Musculoskeletal | Articular or periarticular pain, muscular pain | Usually no joint swelling, no redness |
Venous blood (pulmonary circulation) | Dyspnea, cough, respiratory distress, retrosternal pain worsened on inspiration | Tachypnea, tachycardia, hypotension, frothy bloody sputum, low oxygen saturation |
Central nervous system | Headache, unexplained fatigue, malaise, dizziness, impaired cognitive processes, paresthesias, limb weakness, speech difficulty, visual loss, ataxia, nausea, vomiting, convulsions | Altered state of consciousness, confusion, visual field deficit, unusual distribution of sensory deficits, motor deficits, coordination deficit, gait and walking disturbance, ataxia, positive Romberg sign |
Spinal cord | Back pain, girdling abdominal pain, numbness, paresthesias, limb weakness, urinary or fecal dysfunction (with urinary issues being more common) | Motor and/or sensory deficits, anal sphincter weakness, urinary retention, loss of bulbocavernosus reflex, loss of deep tendon reflexes |
Peripheral nervous system | Numbness or paresthesias in a peripheral nerve distribution | Patchy sensory deficits |
Inner ear | Deafness, vertigo, nausea, vomiting, ataxia, tinnitus | Acute sensorineural hearing loss, nystagmus |
Symptoms might present with some delay. Severe neurologic DCS symptoms usually appear within 10 minutes of surfacing and in 90% of cases symptoms will be present within the first 3 hours. In some cases, it can take up to 24 hours for symptoms to be noticed by the diver.
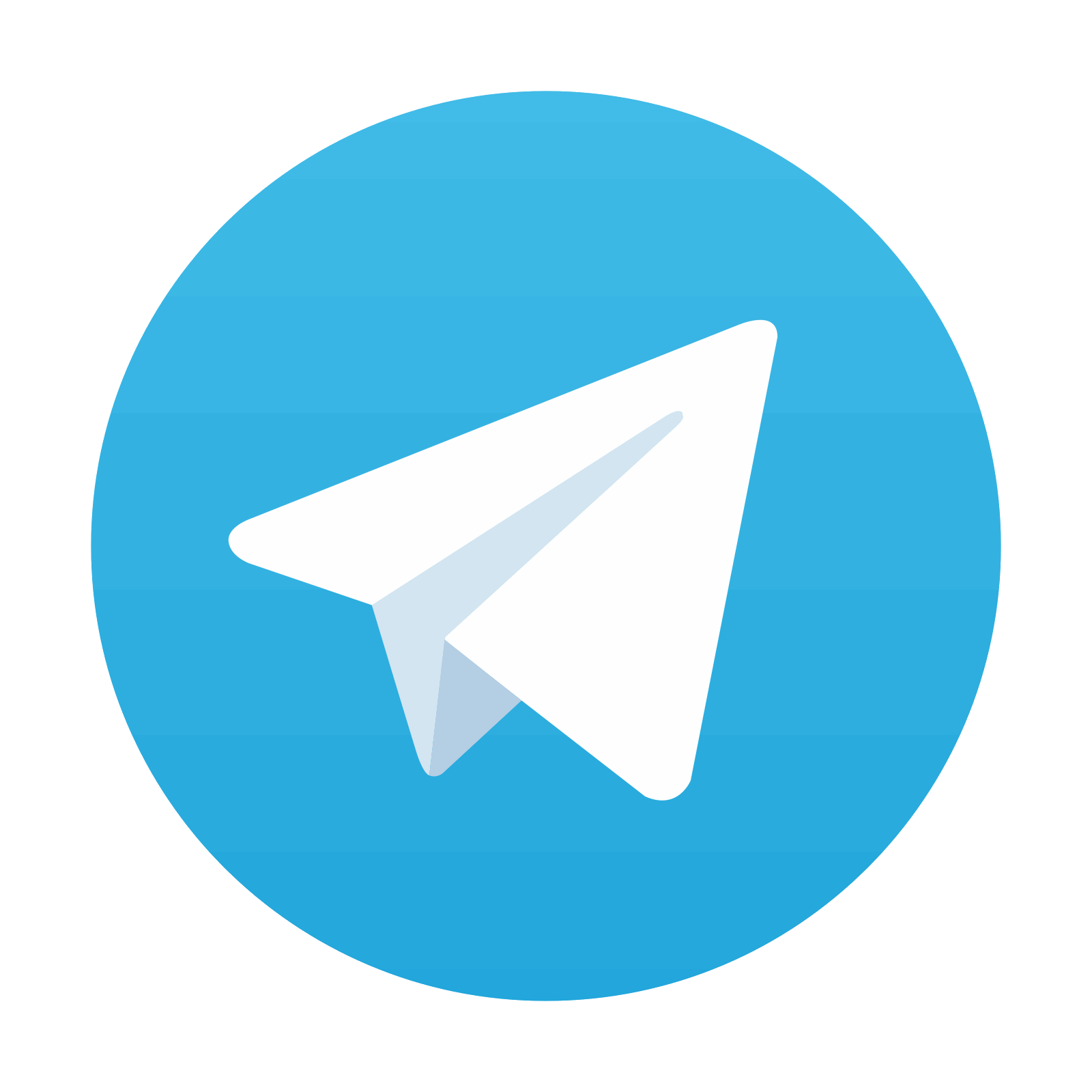
Stay updated, free articles. Join our Telegram channel

Full access? Get Clinical Tree
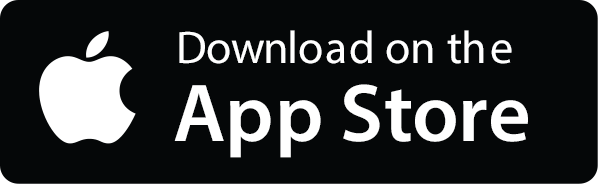
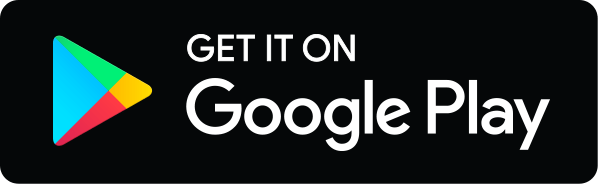