It is estimated 2.75 billion people travel aboard commercial airlines every year and 44,000 in-flight medical emergencies occur worldwide each year. Wilderness medicine requires a commonsense and improvisational approach to medical issues. A sudden call for assistance in the austere and unfamiliar surroundings of an airliner cabin may present the responding medical professional with a “wilderness medicine” experience. From resource management to equipment, this article sheds light on the unique conditions, challenges, and constraints of the flight environment.
Introduction
It is estimated 2.75 billion people travel aboard commercial airlines every year and 44,000 in-flight medical emergencies occur worldwide each year. Wilderness medicine requires a commonsense and improvisational approach to medical issues. A sudden call for assistance in the austere and unfamiliar surroundings of an airliner cabin may present the responding medical professional with a “wilderness medicine” experience. From resource management to equipment, this article sheds light on the unique conditions, challenges, and constraints of the flight environment.
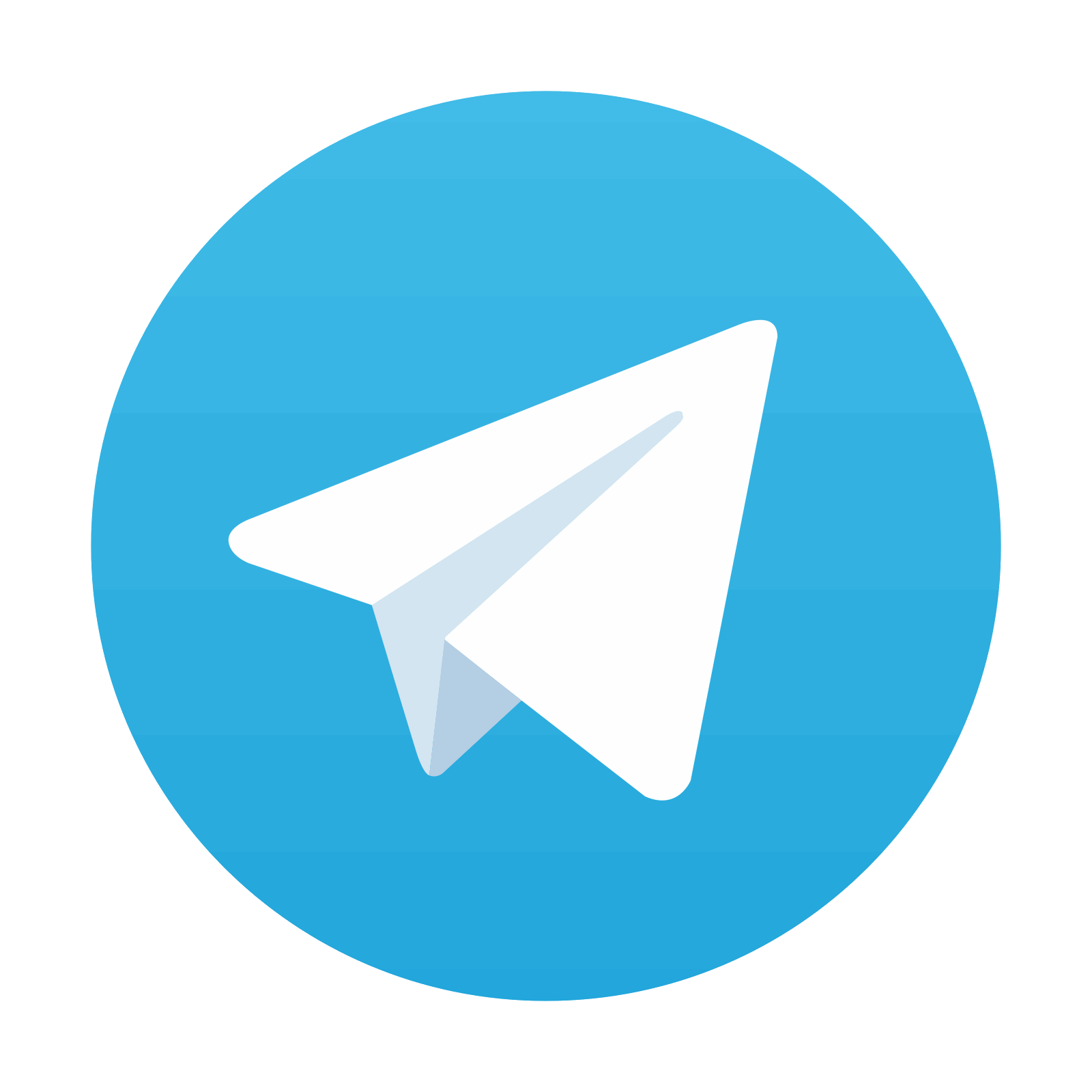
Stay updated, free articles. Join our Telegram channel

Full access? Get Clinical Tree
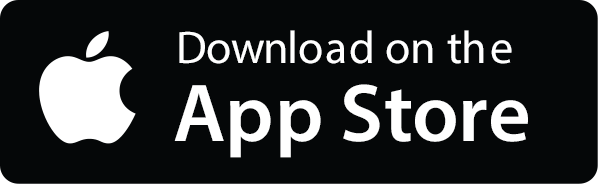
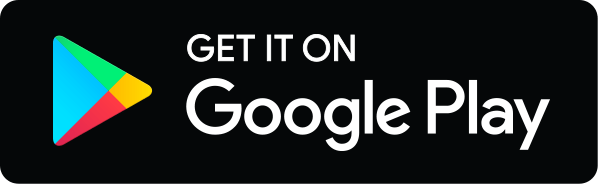