Trauma is the leading cause of death for those younger than 40 years of age, with the majority of death secondary to bleeding within the first few hours of injury.1-3 Damage-control resuscitation has become the standard of care for battlefield resuscitation and now is becoming more common in civilian trauma.4 The goal is rapid hemorrhagic control with administration of balanced whole products in a plasma, platelet, and red blood cell at 1:1:1 ratio that mimic fresh whole blood, treat coagulopathy, and minimize colloid administration in patients requiring massive transfusion (≥ 10 units packed red blood cells [pRBC] in 24 hours).4
The European Guidelines on management of major bleeding and coagulopathy following trauma stratified their recommendation as such: Grade 1A for strong recommendation, high quality of evidence due to benefits clearly outweigh risks and quality of evidence is from randomized controlled trials (RCTs) without important limitations or from overwhelming evidence from observational studies; Grade 1B for strong recommendation moderate quality of evidence due to benefits clearly outweigh risks and quality of evidence is from randomized controlled trials with limitations; Grade 1C strong recommendation, low quality or very low quality of evidence due to benefits clearly outweigh risks and support is from observational studies; Grade 2A weak recommendation, high quality of evidence due to benefits balance risks and evidence is from randomized control trials without important limitations or from overwhelming evidence from observational studies; Grade 2B weak recommendation and moderate quality of evidence due to benefits balance risks with randomized control trials with important limitations; and Grade 2C weak recommendation and low quality of evidence due to uncertainty in estimates of benefits and risks and evidence is from observational studies or case series.5 Time between injury and control of bleeding needs to be minimized, since most fatalities occur within 24 hours of injury (Grade 1A).5 Management bundles have been created to expedite care (Table 29-1). Tourniquets need to be applied to presurgical open extremity injuries and can be left in place for up to 6 hours (Grade 1B).5 Major bleeding may not always be obvious and clinical presentation may correlate to the degree of blood loss (Table 29-2). Mechanisms of injury that suggest major bleeding include falling from a height of 20 feet or more, high-energy deceleration impact, and high-velocity gunshot wounds.5 Radiographic studies of the head, chest, abdominal cavity, and pelvis are often needed, and hemodynamic instability would warrant emergent surgery.5
Prehospital Bundle | Intrahospital Bundle | Coagulation Bundle |
---|---|---|
|
|
|
Class 1 | Class II | Class III | Class IV | |
---|---|---|---|---|
Blood loss (mL) | Up to 750 | 750–1500 | 1500–2000 | > 2000 |
Blood loss (% blood volume) | Up to 15% | 15–30% | 30–40% | > 40% |
Pulse rate (beats/min) | < 100 | 100–120 | 120–140 | > 140 |
Systolic blood pressure | Normal | Normal | Decreased | Decreased |
Pulse pressure (mmHg) | Normal or increased | Decreased | Decreased | Decreased |
Respiratory rate | 14–20 | 20–30 | 30–40 | > 35 |
Urine output (mL/h) | > 30 | 20–30 | 5–15 | Negligible |
Central nervous system/mental status | Slightly anxious | Mildly anxious | Anxious, confused | Confused, lethargic |
Initial fluid replacement | Crystalloid | Crystalloid | Crystalloid and blood | Crystalloid and blood |
Acute traumatic coagulopathy (ATC) consists of derangements in prothrombin time (PT), partial thromboplastin time (PTT), and thrombin time (TT); thrombocytopenia; low fibrinogen; and a disseminated intravascular coagulation (DIC) score of 4 or greater, associated with trauma.6 Although the true pathogenesis is unknown, ATC is believed to occur within 30 minutes of injury secondary to anticoagulation effects of protein C with inhibition of factors VIIIa and Va, profibrinolytic effects from inhibition of plasminogen activator inhibitor-1, and tissue factor expression with exposure of collagen, localized coagulation, platelet activation, and thrombin generation.6-8 Acute traumatic coagulopathy is an independent predictor of massive transfusion, protracted intensive care unit (ICU) stay, multiorgan failure, and death.7,9-14
Acute traumatic coagulopathy is worsened by the consumption of coagulation factors and platelets; the dilutional effect secondary to replacement of lost whole blood with replacement fluids and blood products; hormonal and cytokine changes that lead to endothelial cell activation; dysfunction of platelets and coagulation proteases secondary to hypothermia and acidosis; and continued bleeding causing lost axial flow (red cells flow in the middle while platelets and plasma are in proximity to endothelium) and little contact of platelets and plasma with endothelium.9
Hypothermia is caused by heat loss at the scene and from treatments such as intravenous (IV) fluids.4 Coagulation protease activity and dysfunction with platelet activation, aggregation, and adhesion are seen when temperatures decrease from 37°C to 33°C.4 Acidosis secondary to injury, hypoxia, hyperperfusion, and subsequently lactic acidosis contributes to coagulopathy, with protease dysfunction as suggested by a 50% reduction of prothrombinase activity with a pH less than 7.2, decreased thrombin generation, and increased fibrinolysis.4,15-17
Dilutional effects are suggested by 1 study when coagulopathy is induced in 40% of trauma patients receiving 2 Liters of crystalloid and in 70% of trauma patients receiving 4 Liters of crystalloid.10 Starch-based colloids coat von Willebrand factor (vWF) and fibrinogen, causing them to be dysfunctional and to cause falsely high fibrinogen readings in assays.8,11 It has been suggested that dilutional effects prolonged PT secondary to a reduction of factor VII and starch colloids, leading to fibrinogen dysfunction and worsening clot stability.4,8,18
To control acidosis, hypothermia, hypoperfusion, and coagulopathy, damage control resuscitation (DCR) is recommended for severe hemorrhagic shock, particularly in abdominal trauma and orthopedic surgery (Grade 1B).4,5 Known triggers for DCR include hypothermia (≤ 34°C), pH less than or equal to 7.2, inaccessible major venous injury, extensive surgery, and coagulopathy.5 In abdominal and orthopedic trauma, DCR consists of 3 parts. The first part involves control of bleeding and decontamination. Packing for at least 48 hours and temporal closure are performed. The second part involves rewarming, correction of coagulopathy, improvement of acid–base status, and any other procedures.5 The third part involves definitive surgery.5
In patients with no traumatic brain injury (TBI) nor spinal injury, tissue oxygenation, prevention of dislodgement of clot, dilutional effects on coagulation factors by over-resuscitation, and hypothermia are achieved via permissive hypotension (systolic blood pressure [SBP] goal, 80–90 mmHg).5 The recommendation is grade 1C, since there are no good randomized trials, although this approach showed improved survival in penetrating trauma and penetrating and blunt trauma.19,20 Dutton et al randomized patients with hemorrhagic shock to target SBP greater than 100 mmHg or SBP of 70 mmHg until hemostasis is achieved.21 The study showed no difference in injury severity score (19.65±11.8 vs 23.64±13.8; P = 0.11), duration of active hemorrhage (2.97±1.75 hours vs 2.57±1.46 hours; P = 0.20), or overall survival.21
Despite the recommendation that crystalloids, particularly normal saline (NS), may be used as the initial form of resuscitation in trauma patients (Grade A1), it is limited to 1 to 1.5 Liters due to dilutional and hypothermia effects, and normal saline can induce acute kidney injury (AKI) with hyperchloremic metabolic acidosis.5,22,23 In addition to fibrinogen and von Willebrand factor dysfunction and dilutional effects of starch-based colloids, they can also induce renal failure and have not been shown to improve survival compared to crystalloids.5 With hypertonic saline (HS), a randomized controlled trial comparing it to dextran and Ringer lactate had improved acute respiratory distress syndrome (ARDS)-free survival in patients requiring 10 or more units of pRBC24, and another study showed a more effective reduction in elevated intracranial pressure.25 However, Cooper et al failed to show any improvement in neurologic function in 6 months in patients treated with hypertonic saline compared to normal saline, and in 2 large prospective trials, Bulger et al failed to show any advantage with HS compared to NS.26-28 The composition of crystalloids and colloids in comparison to the composition of blood is shown in Table 29-3.
pH | Na | K | Cl | Ca | Mg | Lactate | Acetate | Gluconate | Dextrose | Osmolarity | ||
---|---|---|---|---|---|---|---|---|---|---|---|---|
Normal in Blood | 7.35 | 140 | 4 | 104 | 2.3 | 1 | 285 | |||||
Crystalloid | ||||||||||||
Ringer lactate | 130 | 4 | 109 | 1.5 | 28 | 273 | ||||||
PlasmaLyte | 7.4 | 140 | 5 | 1.5 | 27 | 23 | 295 | |||||
Normal saline | 5.7 | 154 | 154 | 308 | ||||||||
3% Saline | 5 | 513 | 513 | 1025 | ||||||||
D5W | 4.3 | 278 | 272 | |||||||||
Colloid | ||||||||||||
Albumin | 6.9 130-160 | 310 | ||||||||||
Dextran | 154 | 154 | 310 | |||||||||
Hetastarch | 154 | 154 | 310 |
Resuscitation with blood products is preferred over crystalloids and colloids with a goal hemoglobin of 7 to 9 g/dL (Grade 1C), platelets of 50 × 109/L (Grade 1C) or 100 × 109/L with ongoing bleeding or traumatic brain injury (Grade 2B), PT and aPTT less than 1.5 times normal (Grade 1C), and fibrinogen of 1.5 to 2 g/L (Grade 1C).5 Blood work may not be available during the initial phase of resuscitation and should not preclude the use of blood products. These transfusion goals become more important during the goal-directed phase of resuscitation.
Fresh whole blood has been split to its components for storage purposes. Packed RBCs can be stored for 42 days at 4°C, platelets can be stored for 5 days at 22°C, cryoprecipitate can be stored for 1 year at −20°C, and fresh frozen plasma (FFP) can be stored for 1 year at −20°C. Glycerol is used to protect cells. With component transfusion, some of the blood products are lost. There is a 20% reduction in RBCs. There is 70% diminished of factor function from FFP, with factor V and VII affected the most. Dried plasma has been used, and it can be stored for up to 15 months. Liquid plasma is the best, and it is not frozen. It is available for use within 28 days. Type O is the universal donor. The Prospective, Observational, Multicenter Major Trauma Transfusion (PROMMTT) prospective cohort study suggested that trauma patients who received less than a 1:2 ratio of pRBC to platelets or pRBC to FFP were 3 to 4 times more likely to die compared to a 1:1 ratio or higher within the first 24 hours.12 A decreased 6-hour mortality was found with increased ratios of plasma to RBCs (adjusted hazard ratio [aHR], 0.31; 95% confidence interval [CI], 0.16–0.58), and platelets to RBCs (aHR, 0.55; 95% CI, 0.31–0.98).12 The Pragmatic, Randomized Optimal Platelet and Plasma Ratios (PROPPR) study showed no significant 24-hour and 30-day mortality in trauma patients given plasma, platelets, and pRBC at a 1:1:1 ratio compared to a 1:1:2 ratio (12.7% vs 17%; 95% CI, −9.6% to 1.1%; P = 0.12; and 22.4% vs 26.1%; 95% CI, −10.2% to 2.7%; P = 0.26, respectively).29 However, exsanguination, which is the most common cause of death within the first 24 hours, was significantly decreased in the 1:1:1 ratio compared to the 1:1:2 ratio (9.2% vs 14.6%; 95% CI, −10.4% to −0.5%).29 A retrospective study of trauma patients who were transfused with warm frozen whole blood (FWB) versus component therapy at a 1:1:1 ratio showed improved 24-hour and 30-day survival in the warm FWB compared to component therapy (96% vs 88%, P = 0.018; and 95% to 82%, P = 0.002).30 The volume of warm FWB was less than component therapy because of a lack of additives and anticoagulants, and it was associated with improved 30-day survival.30 Virology may prohibit fresh whole blood use in civilian trauma.
It has been suggested that a platelet count less than 50 × 109/L or a fibrinogen level less than 0.5 g/L and a platelet count less than 100 × 109/L are predictors of microvascular bleeding, and those levels represent the threshold for transfusing and the predictor of progression of intracerebral hemorrhage, respectively.5,31,32 Initial thrombocytopenia may not be obvious in the initial phase due to release of platelets from bone marrow and the spleen.5,33,34 Thrombocytopenia as low as 50 × 109/L is found when 2 Liters of blood loss are replaced by crystalloids or red cells.5,35 Furthermore, there is growing evidence to support that qualitative dysfunction of platelets, particularly platelet aggregation, can have as much or more impact on mortality as quantitative function.5,35-39 There is evidence that qualitative dysfunction of platelets is evident in acute traumatic coagulopathy.5 Platelet transfusions also are recommended for patients who are receiving antiplatelet therapy with intracerebral hemorrhage, and platelet function should be monitored (Grade 2C).5 Five units of platelets are required to normalize platelet activity in patients taking aspirin, and 10 to 15 units of platelets are required to normalize platelet activity in patients taking aspirin and clopidogrel.5,40 This may be repeated. Desmopressin, at a dose 0.3 μg/kg diluted in 50 mL of normal saline and given over 30 minutes, enhances platelet aggregation and adherence and is recommended for trauma patients on antiplatelet agents or who have von Willebrand disease (Grade 2C).5 It has been suggested that administration of desmopressin with platelet transfusions improve platelet dysfunction, but it has not been shown to decrease radiographic progression of intracerebral hemorrhage or mortality.41-49 Recombinant factor VIIa and fibrinogen have been shown to improve hemostasis in patients receiving APA.5
Fibrinogen is the most essential substrate in clot formation and is the earliest and most affected factor in ATC.5 Recently, it has been recommended that transfusion of 2 g of fibrinogen to mimic the first 1:1 ratio of the first 4 units pRBC has improved survival with fibrinogen correction (Grade 1C).5,50 Cryoprecipitate that contains fibrinogen, factors VIII and XIII, vWF, and fibronectin is given when fibrinogen is 1 g/L to 1.5 g/L, since fibrinogen is activated by thrombin to form insoluble fibrin.9
Prothrombin complex concentrates (PCC) are produced from the cryoprecipitate supernatant after removal of antithrombin and factor XI. They are used to correct congenital or acquired vitamin K–dependent coagulation factor defects. There are 2 types: PCC 3 contains factor II, IX, and X, and PCC 4 contains factors II, VII, IX, and X, all at concentrations 25 times higher than in plasma. They also may contain heparin, protein C, and protein S. Half-lives differ, at 6 hours for factor VII and 60 to 72 hours for factor II.51 Prothrombin complex concentrates are in small volumes and can be administered rapidly.51 Therefore, the advantages of PCC over FFP in correcting vitamin K–factor coagulopathy include (1) faster correction of the international normalized ratio (INR); (2) smaller volumes: 15 mL/kg of FFP versus 1 to 2 mL/kg of PCC; and (3) faster preparation.51 Complications include heparin-induced thrombocytopenia and thrombogenic events.51 It has been recommended to administer PCC in patients who need early reversal of vitamin K–dependent oral anticoagulants (Grade 1A).5
Tranexamic acid (TXA) is an antifibrinolytic agent that is a competitive inhibitor of plasminogen with a plasma half-life of 120 minutes.5 The Clinical Randomisation of Antifibrinolytic Therapy in Significant Haemorrhage (CRASH-2) trial studied adult trauma patients who were given TXA (1 g loading dose followed by an 8-hour infusion dose) compared to placebo.52 Administration of TXA reduced all-cause mortality, risk of bleeding deaths, and exsanguination within the first 24 hours.52-58 There was no increased risk of venous or arterial thrombotic events, such as deep venous thrombosis and myocardial infarctions, respectively.52-58 Tranexamic acid should be given within 3 hours of injury, since there is a 1.3% increase of bleeding if given after 3 hours.5,52-59 One potential complication is seizure in cardiac pulmonary bypass patients.58 Aminocaproic acid is 10-fold weaker than TXA and is administered with a loading dose of 150 mg/kg followed by a continuous infusion of 15 mg/kg/h.
Additional interventions are necessary to achieve hemostasis in patients on direct oral anticoagulants. There are 2 groups: direct thrombin inhibitors such as dabigatran, and factor Xa inhibitors such as apixaban, rivaroxaban, and edoxaban60 (see Fig. 29-1). Dabigatran has been approved for treatment of nonvalvular atrial fibrillation, deep vein thrombosis (DVT), and pulmonary embolism (PE). It peaks 2 to 3 hours after administration, and 80% is eliminated through the kidneys and 20% through the liver.60 It binds to thrombin (factor IIa).61 Thrombin time and ecarin clotting time (ECT) are used to monitor levels.62-66 There are 3 available factor Xa inhibitors that directly inhibit free and clot-bound factor Xa. Rivaroxaban is approved for nonvalvular atrial fibrillation (AF) and DVT. It peaks at 2 to 4 hours after oral administration, and two-thirds undergoes metabolic degradation and one-third is unchanged and eliminated via the kidneys.60,67-70 Apixaban is approved for nonvalvular AF and DVT. It has a 15-hour half-life, and 35% is removed via the kidneys.60,71,72 Edoxaban and betrixaban are other factor Xa inhibitors. Generally, Factor Xa assays are needed to monitor levels and activity.60,73,74
Nonspecific reversal agents for dabigatran include charcoal, if administered within 1 to 2 hours of ingestion; desmopressin, by stimulating release of factor VIII and vWF, PCC; and hemodialysis, because of dabigatran’s low protein binding.60 Idarucizumab is a humanized monoclonal antibody fragment that binds to both free and thrombin-bound dabigatran with an affinity 350 times greater than for thrombin.60,75-79 Idarucizumab 5 g IV reverses dabigatran in a dose-dependent manner with normalization of ECT, TT, and aPPT values.75-79 Andexanet alfa is a recombinant modified human factor Xa decoy that binds to factor Xa inhibitors with a high affinity, allowing factor Xa activity. It is administered as a bolus followed by infusion.60,80-83 Reversal is assessed via decrease in antifactor Xa, unbound factor Xa inhibitors, and restoration of thrombin generation.60,80-83 Reversal was noted within 2 to 5 minutes after bolus and was maintained with continuous infusion.60,80-83 Reversal stopped after 1 to 3 hours after cessation of continuous infusion.60,83-86
The European Task Force for Advanced Bleeding Care from Major Trauma recommends routine monitoring of coagulopathy such as PT/INR, PT, and fibrinogen.5 These laboratory studies are limited by (1) an inability to monitor quantities of coagulation factor and thrombin potential; (2) a lack of monitoring interactions between coagulation proteases and phospholipids; and (3) the length of time (at least an hour) to obtain results.5,6 Therefore, viscoelastic studies such as thromboelastography (TEG) and rotational thromboelastometry (ROTEM) have been suggestive as valuable tools in understanding coagulopathy and guiding transfusions in trauma. (Grade 1C).5,6 If they are not available during the initial phase of resuscitation, they are still important during the goal-directed resuscitation phase. Serial lactic acid may also serve as surrogate markers for bleeding and shock (Grade 1B).5 Hypocalcemia can occur during massive transfusion secondary to citrate binding and should be monitored and maintained at normal levels (Grade 1C).5
Despite this discussion of controlling hemorrhage, trauma patients are at high risk for thromboembolism. It is recommended that pharmacologic thromboprophylaxis be initiated within 24 hours of control of hemorrhage (Grade 1B).5 Pneumatic compression (Grade 1C) and stockings (Grade 2C) are also recommended. Inferior vena cava filters as prophylaxis are not recommended. (Grade 1C)5
Every year in the United States, there are 40,000 hospitalizations and 3400 deaths from burns.84-89 Survival is 97% for patients admitted to burn centers.84-89 The etiology of burns includes thermal, electrical, and chemical burns, and depth of burns includes superficial, superficial dermal, and full thickness (Tables 29-4 and 29-5).84
Type | Causes | Description |
---|---|---|
Thermal burns | Scalds: 70% of burns in children and also in elderly | Superficial burns |
Flame: 50% of burns in adults, sometimes with inhalation injury | Deep dermal or full thickness | |
Contact | Deep dermal or full thickness | |
Electrical | 3–4% of burns Entry and exit points Voltage strength directly proportional to extent of tissue damage | |
Low voltage or domestic electricity | Small contact burns at entry and exit sites, can cause arrhythmias | |
True high-tension injuries if ≥ 1000 V | Tissue bone necrosis, rhabdomyolysis, fatal if > 70,000 V | |
Flash injury: arc current from high-tension voltage | Superficial burns, although clothes can be set on fire; no current passing through body | |
Chemical | Alkalis are worse than acids | Deep burns; can continue to cause necrosis even after agent is removed |
Chromic acids | ||
Dichromatic acid | ||
Hydrofluoric acid |
Burns | Depth | Characteristic |
---|---|---|
First Degree | Superficial epidermis | Red, good capillary refill, painful, healing with no scar after 5-10 days |
Second Degree | Partial dermis | Red with blisters, edema, good capillary refill, painful, healing with minimal scarring after 2-3 weeks |
Second Degree | Deep dermis | Pinkish to white, thick skin texture, poor or no capillary refill, healing with dense scar after 1-2 months |
Third Degree | Full thickness | White, black or brown, leathery with no capillary, painless, no spontaneous healing |
Fourth Degree | Involvement of subcutaneous tissue, tendon, and / or bone | Variable, no capillary refill, painless, no spontaneous healing |
There are 3 zones of burns. The zone of coagulation is exposed to the greatest amount of heat and has irreversible tissue damage.84-86 The tissue must be exposed to temperatures greater than 41.1°C (106°F), which is needed for protein to be denatured.85 The zone of stasis surrounds the zone of coagulation. It is associated with decreased tissue perfusion and is potentially salvageable. However, continued hypoxia and ischemia can lead to tissue necrosis if no intervention is done within 48 hours.85 The mechanism is secondary to autophagy within the first 24 hours and followed by apoptosis in the next 24 hours.85 The zone of hyperemia is the outermost area where there is increased perfusion; tissue in the zone of hyperemia will likely recover.84-86 Burn healing is a dynamic process with overlapping stages of inflammation, proliferation, and remodeling.85
Severe burns affecting 20% total body surface area (TBSA) can result in burn shock, which is a combination of hypovolemic, distributive, and cardiogenic shock.84,90,91 Intravascular volume is depleted secondary to increased capillary permeability, and only 30% of the body surface area can be resuscitated due to reduced sodium ATPase activity, disruption of the cellular transmembrane ionic gradient, and protein loss and secondary to microvascular injury from inflammatory mediators.91 There is increased systemic vascular resistance and decreased cardiac output.89
Mortality is increased if resuscitation is delayed by 2 hours, and hypovolemic shock occurs if resuscitation is delayed in patients with burns of greater than 15% to 20% TBSA. The goal is to provide fast resuscitation while avoiding the sequela of over-resuscitation, as suggested by the phenomenon called fluid creep, which can lead to pulmonary edema and compartment syndrome. This has led to exploration of other fluid resuscitation methods, such as albumin and hypertonic saline.
In burn patients, the primary survey starts with airway evaluation followed by vascular access and monitoring devices.90,91 Primary injury occurs from direct thermal injury and secondary injury results from systemic inflammatory response. The secondary survey includes determination of mechanism of injury; evaluation for inhalation injury and carbon monoxide intoxication, corneal burns, and abuse; and then a detailed assessment of burns.90,91
Resuscitation with crystalloid fluids within the first 24 hours is key, using the Parkland formula or modified Brooke formula generally is reserved for second- or third-degree burns. The Parkland formula calculates the amount of crystalloid fluids as 4 mL/kg multiplied by the percentage of TBSA burned. The first half is given over the first 8 hours and the second half is given over the next 16 hours. Fluid resuscitation is crystalloid, usually lactated Ringer over isotonic saline because its more physiologic, and after 18 to 24 h when capillary integrity is restored, colloids can be used (5% albumin with lactated Ringer).
The rule of nines or the palm rule can be used to calculate body surface area. Burns less than 15% BSA are not associated with extensive capillary leak. Pulse, blood pressure (BP), and urine output (0.5-1 mL/kg) are monitored to measure adequate perfusion. Urine output goals are 30 to 50 mL/h, and higher goals should be avoided in order to prevent over-resuscitation. Gradual increases in maintenance fluids instead of boluses prevent worsening edema. Pigmented urine, such as in high voltage or deep thermal injury, should be cleared. Glycosuria also can elevate urine output falsely, and some patients might require diuresis. Urinalysis should be obtained at least 8 hours after resuscitation.
Although it has been hypothesized that capillary leak is improved at 18 to 24 hours, this is not certain. One test involves administering equal portions of albumin and Lactated Ringer, and if urine output improves, this could suggest that capillary leak has resolved. An infusion of 5% albumin is given over 24 hours at a dose of 0.5 mL/kg/% TBSA burned; free water in mL/h is determined by the following equation: (25 + % TBSA burned) × BSA (mm2). Sodium must be monitored since the albumin is rich in protein. Unlike crystalloids, of which only 20% to 30% remain in the intravascular space, 50% of colloids remain in the intravascular space. Other centers prefer FFP at 0.5 to 1 mL/kg for the first 24 hours. Dextran has twice the oncotic pressure of albumin and reduces erythrocyte aggregation. This reduces edema formation in nonburned areas, although removal of dextran leads to edema if capillary leak still present. Hypertonic saline (180–300 mEq/L Na), with a serum goal of 160 mEq/L or less, theoretically mobilizes extravascular fluid into the vascular space by increasing the osmotic gradient. A compromise is to use lactated Ringer with 50 mEq sodium bicarbonate. The greatest benefit is seen in inhalation injury and in burns involving 40% TBSA or more.
Burn depth can be underestimated, since tissue may initially appear viable, but appearance changes over time. The Lund and Browder chart or the rule of nines is employed for adults (9% TBSA for the head, neck, and each arm; 18% TBSA for the anterior thorax, posterior thorax, and each leg; 1% TBSA for the perineum).
First-degree burns are limited to the epidermis and are similar to a sunburn without blisters. They are red, dry, and painful. There are 2 types of second-degree burns. Superficial partial-thickness burns involve papillary dermal elements and are pink, moist, and painful. There is blister formation and heal well without skin graft. Deep partial-thickness burns involve deeper reticular dermis and have a variable appearance from pink to white, with a dry surface, diminished sensation, and sluggish or absent capillary refill. Third-degree burns are leathery, dry, and waxy. They will contract but not heal. Blisters occur in second- and third-degree burns. Fourth-degree burns involve subcutaneous tissue, tendon, or bone. Circumferential or near-circumferential burns should be noted because they can progress to ischemia or interfere with ventilation.
High doses of ascorbic acid (vitamin C) during the initial 24 hours post-burn reduced fluid requirements by 40%, tissue water by 50%, and ventilator days.91
Early nutritional support is important and is associated with shorter hospital stays, increased wound healing, and decreased infections.91 Carbohydrates and fat supplementation might be minimized, since hyperglycemia leads to increase in systemic inflammatory response syndrome (SIRS), and fat can cause further immunosuppression.85 Amino acids and vitamins are key, and it is hypothesized that insulin administration delays the catabolic state and increases protein synthesis.85,92-96
Virtually every patient will meet criteria for SIRS. Early excision and skin graft is now the accepted practice. In adults, there is no role for prophylactic antibiotics. Human tetanus immunoglobulin (250–500 IU) is administered regardless of immune status to prevent tetanus.86 Tetanus toxoid 0.5 mL is also given intramuscularly (IM) if the patient has not received complete primary immunization or has not received a booster in the last 10 years.86 Patients partially immunized or never immunized should also receive the vaccine.86
Early excision, 24 to 48 hours from injury, is associated with decreased blood loss, length of stay, infection, graft take, and mortality.85,96-100 Full-thickness burns are treated with an autograft, which is a split-thickness skin graft from the patient’s uninjured skin.85 With an autograft, risk of rejection is low, although pain and the wound healing burden on donor sites are high.87 Temporary coverage can also be achieved with allografts, tissue from other living or dead donors; xenografts, tissue from different species; skin substitutes; and dermal analogs.85 The traditional dressing approach is mafenide acetate in the morning and a silver sulfadiazine cream at night covered with gauze.85 There are some dressings impregnated with silver, although it has been suggested that silver delays wound healing. Topical antibiotics have been used to prevent the development of infection, to reduce microbial load, and to decrease conversion from a partial to a full-thickness burn (Table 29-6).86,101-103 It is an adjunct to early debridment.86,101-103
Topical Agent | Preparation | Eschar Penetration | Antibacterial Activity | Major Toxicity |
---|---|---|---|---|
Silver nitrate (AgNO3) | 0.5% solution | None | Bacteriostatic against aerobic Gram-negative bacilli, Pseudomonas aeruginosa, limited antifungal | Electrolyte imbalance |
Silver sulfadiazine (Silvadene, Flamazine, Thermazine, Burnazine) | 1% water-soluble cream (oil-in-water emulsion) | None | Bactericidal against aerobic Gram-negative bacilli, P aeruginosa, some Candida albicans | Leukopenia |
Mafenide acetate (Sulfamylon) | 10% water-soluble cream (oil-in-water emulsion), 5% solution | Limited | Broad spectrum against aerobic Gram-negative bacilli, P aeruginosa, anaerobes | Metabolic acidosis |
Nanocrystalline silver dressings (ACTICOAT A.B. dressing, Silverlon) | Dressing consisting of 2 sheets of high- density polyethylene mesh coated with nanocrystalline silver | Moderate | Potent activity against aerobic Gram-negative bacilli, P aeruginosa, aerobic Gram-positive bacilli, MRSA, VRE, multidrug-resistant Enterobacteriaceae | Limited toxicity |
Infections are serious complications in burns, and it might be difficult to distinguish colonization from infection. Increasing inflammation (edema, redness, pain), purulent discharge, and fever and leukocytosis can determine true infection.86 Wound swabs may show purulence but true infection will have elevated polymorphonuclear neutrophil (PMN) count with moderate to heavy growth from pathogenic organisms.86 Tissue quantitative cultures for infection would have 104 or more CFU/g or 105 or more colony-forming units (CFU)/g of tissue, and predicted potential of sepsis, and can have 75% mortality.86,104 A polymerase chain reaction (PCR) may be able to identify the organism, but the infection will still need culture to help determine antibiotic sensitivities.86
There are 4 types of burn-related infections: (1) burn impetigo with loss of epithelium from previously epithelized surface not related to trauma, which is treated with cleaning of debris and exudates, topical antistaphylococcal antibiotics, and grafting of unstable areas; (2) burn-related surgical wound infection that is surgically created in nonepithelized skin with loss of overlying graft or membrane, which is treated in a similar way to burn impetigo; (3) burn wound cellulitis, which is an infection of uninjured skin surrounding the wound with local progression, is treated with antibiotics against Streptococcus pyogenes; and (4) invasive burn wound infection with infection in unexcised burn and invasion into underlying tissue that is diagnosed by histology or cultures and that is treated with systemic antibiotics and wound excision.86
Traumatic brain injury encompasses many forms, which are not mutually exclusive. In addition to intracerebral hemorrhage, there are 4 types of hemorrhage associated with traumatic brain injury. An epidural hematoma is an arterial bleed, usually from the middle meningeal artery at the level of the foramen spinosum, from rupture of the anterior meningeal artery, or from a dural arteriovenous fistula. Bleeding occurs between the dura and the skull and radiographically appears as concave. A subdural hematoma is usually secondary to torn bridging veins between the surface of the brain and the dural sinuses, but it may occur from the small cortical arteries. Bleeding occurs between the dura and arachnoid spaces radiographically appears as a crescent. A subarachnoid hemorrhage has many etiologies that may directly or indirectly lead to trauma, such as arteriovenous (AV) malformation, cocaine use, cavernous sinus thrombosis, cerebral amyloid angiopathy, sickle cell disease, pituitary apoplexy, arterial dissection, or moyamoya. An associated skull fracture and contusion may suggest that the subarachnoid hemorrhage is a direct consequence of trauma. A diffuse axonal injury is the result of deceleration and acceleration and/or rotational injury and radiographically appears as areas of focal 5- to 15-mm intraparenchymal hemorrhage.
Linear skull fractures extend through the thickness of the calvarium and have no clinical significance unless they cross the middle meningeal groove or dural sinuses.105 Depressed skull fractures occur below the adjacent skull and are usually associated with traumatic brain injury.106 A basilar skull fracture involves the plate of the ethmoid bone, the orbital plate of the frontal bone, the petrous and squamous portions of the temporal bone, and the sphenoid and occipital bones.107,108 It can lead to epidural hematomas, dural tears leading to cerebrospinal fluid (CSF) leaks, and cranial nerve palsies. A Battle sign or retroauricular or mastoid ecchymosis, periorbital ecchymosis or raccoon eyes, clear rhinorrhea or otorrhea, and hemotympanum may appear 1 to 3 days after the event and are clinical signs of a basilar skull fracture. Penetrating fractures usually occur from bullets, knives, and explosives and are usually associated with traumatic brain injury.109
The central nervous system is encompassed in a fixed, nearly incompressible space. The skull has a total volume of 1450 mL, with the brain comprising 1300 mL (85%), CSF comprising 65 mL (5%), and blood comprising 110 mL (10%).110,111 Cerebrospinal fluid is continuously produced at the rate of 20 mL/h (total of 500 mL/24 h) in the choroid plexus and is absorbed in the arachnoid granules in venous circulation. According to the Monro-Kellie hypothesis, these components are constant and an increase in one means a decrease in another.111 Otherwise, pressure increases and herniation can occur. Intracranial pressure (ICP) varies with age, but generally, in adults is 5 to 15 mmHg (7.5–20 cm H2O) and positional, with lower values obtained if the head is elevated.111 Intracranial pressure also is important in that it influences cerebral perfusion pressure (CPP) and oxygen delivery to the brain, using the following formula:
where MAP is the mean arterial pressure, SBP is the systolic blood pressure, and DBP is the diastolic blood pressure. Normally, there is pressure autoregulation that maintains CPP at 50 to 150 mmHg. Arterioles dilate in response to elevated pressure or constrict in response to low pressure. In pathologic states, this autoregulation is impaired and CPP passively follows cerebral blood flow (CBF), a process called pressure passive flow.111
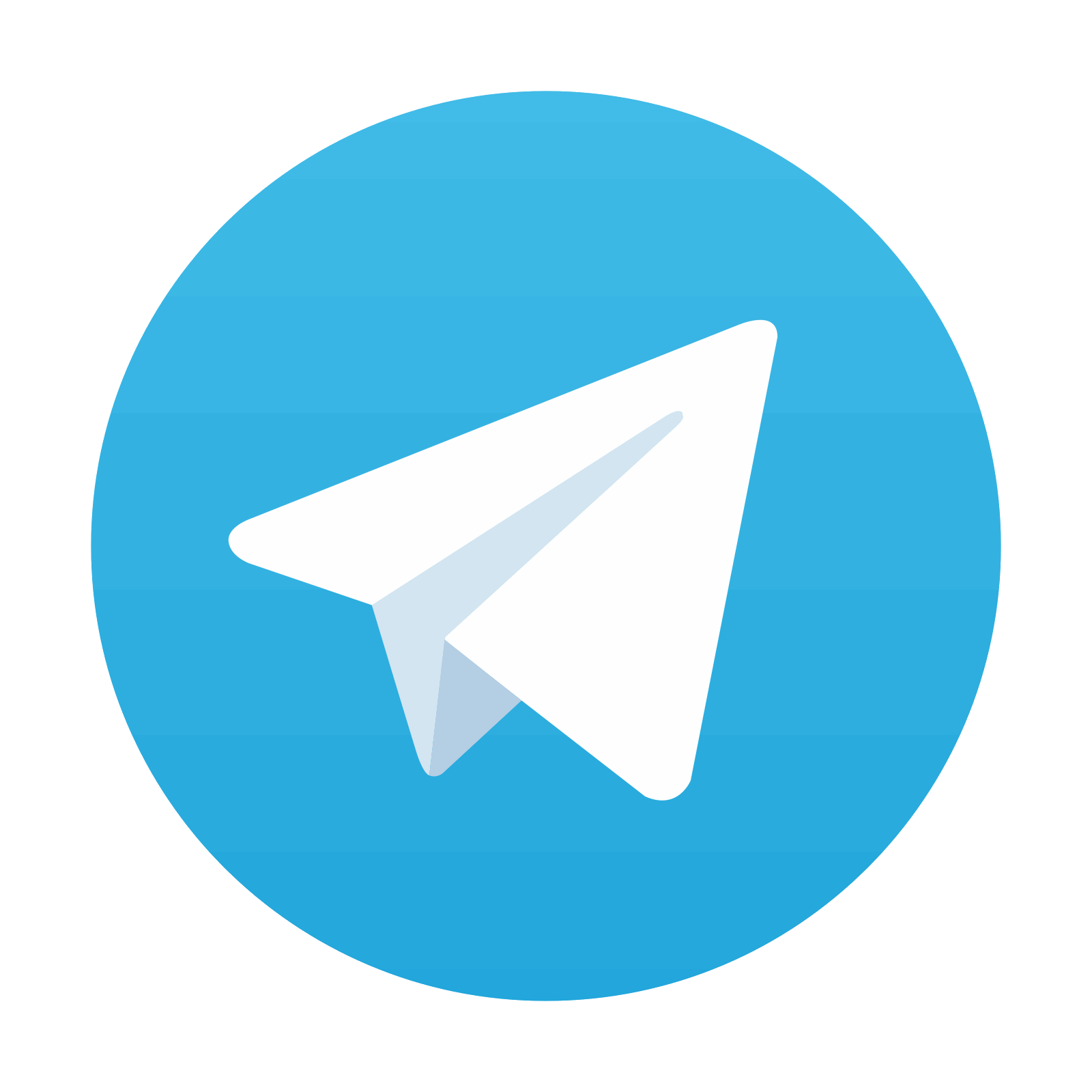
Stay updated, free articles. Join our Telegram channel

Full access? Get Clinical Tree
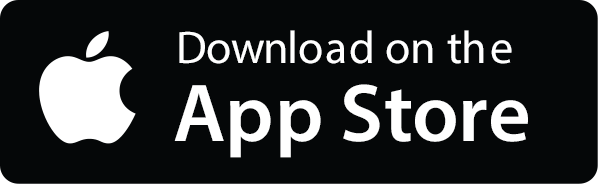
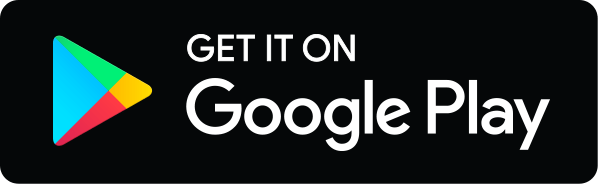