The main function of the lung is gas exchange: the elimination of carbon dioxide (CO2) and the delivery of oxygen (O2) to the tissues. When either function is impaired, the result is respiratory failure. Hypercarbic respiratory failure is a consequence of and is in direct proportion to a reduction of alveolar ventilation. Since the third major alveolar gas, nitrogen (N), is inert, any increase in CO2 is accompanied by a reduction of O2, unless supplemental oxygen is provided. When there is an acute or rapid reduction of alveolar ventilation, the result is acute respiratory acidosis. At other times, chronic respiratory failure can result from chronic lung disease, chest wall disease, or an abnormal respiratory control of ventilation. With such patients, there is often compensation of the hypercarbic respiratory failure and the acidosis may be corrected. This chapter will discuss the physiology of hypercarbic respiratory failure and describe clinical scenarios associated with hypercarbia and their associated management.
Ventilation is the process of moving air from the atmosphere to the alveoli for the purpose of exchanging oxygen and carbon dioxide. Room air contains essentially no carbon dioxide. Mixed venous blood has a partial pressure of carbon dioxide (PCO2) of approximately 46 mmHg. Alveolar PCO2 (PaCO2) is approximately 40 mmHg, and there is no significant gradient between alveolar and arterial blood PCO2 (PaCO2). Thus, PaCO2 is a nearly perfect indicator of alveolar PCO2. An elevated PaCO2 indicates hypoventilation or hypercapnic respiratory failure.
Hypercapnic respiratory failure may exist in the presence of or independently of hypoxemia. As stated in Chapter 8, there are 5 mechanisms of hypoxemia: hypoventilation, ventilation/perfusion (V̇/Q̇) mismatch, shunt, diffusion abnormalities, and reduction in oxygen tension. As stated in Chapter 1, the alveolar–arterial (A-a) gradient may help determine which mechanism is involved.
Hypoventilation may be caused by a ventilatory drive problem (“won’t do”) or from a disease of the respiratory system (“can’t do”). The PaCO2 is normally very carefully regulated by the brain, with even small changes resulting in almost immediate changes in ventilation. The normal ventilatory response to elevated PaCO2 is 1.5 to 2 L/min/mmHg. Normal resting minute ventilation is approximately 6 L/min. This indicates that the normal response to an elevated PaCO2 of only 43 mmHg would be to nearly double resting minute ventilation. The increase in ventilatory drive is normally perceived as dyspnea. Clinically, any patient with an elevated PaCO2 who does not appear dyspneic has a blunted ventilatory drive, either from a central nervous system (CNS) problem or from habituation. Hypoxic respiratory drive is much weaker, and patients who are hypoxic may not have symptoms of dyspnea. Hypoventilation due to decreased drive may be caused by a number of primary insults to the central nervous system, the best example being acute drug intoxication such as opiate use.
Even when ventilatory drive is not a problem, hypoventilation can be caused by problems in the spinal cord, nerves, respiratory muscles, or chest wall, without intrinsic lung disease. In all of these conditions, the A-a gradient would be expected to be normal. Only in the presence of intrinsic lung disease will the A-a gradient be elevated (Table 9-1).
Ventilatory drive is reduced during normal sleep, and PaCO2 rises slightly. This can lead to some respiratory instability during the initial few minutes of sleep as the PaCO2 level is “reset” about 2 to 6 mmHg higher. During deep nondreaming sleep (slow wave sleep), breathing becomes very regular, as there is little cortical influence. During rapid eye movement (REM; dreaming) sleep, ventilatory drive is further blunted and breathing becomes erratic. Muscle tone is decreased in REM sleep, and there is a greater dependence on diaphragmatic function, which becomes most apparent in neuromuscular disease states.
The upper airway maintains patency by a series of paired muscles, which are also respiratory muscles of inspiration. During sleep, muscle tone decreases in these muscles as well, leading to the potential for upper airway collapse (snoring and obstructive sleep apnea). Less common than obstructive sleep apnea is the obesity hypoventilation syndrome. These patients hypoventilate during sleep with little or no airway obstruction (“central” apneas). More severe cases will have evidence of hypoventilation during wakefulness and may benefit from respiratory support during sleep. A rare form of sleep related apnea called Ondine’s Curse occurs when automatic ventilatory control is reduced further during sleep, so that patients can profoundly hypoventilate during sleep. This is the congenital central hypoventilation syndrome, and is now known to be a specific defect in the PHOX2b gene.
Acute respiratory failure may be caused by a sudden catastrophic illness that overwhelms lung function and results in inadequate alveolar ventilation and hypercarbia. Extensive pneumonia, chest trauma, drowning, and drug overdose are common examples that can occur in patients with no underlying diseases. Other patients with acute respiratory failure may suffer from underlying conditions such as chronic obstructive pulmonary disease (COPD), asthma, or neuromuscular diseases such as kyphoscoliosis and muscular dystrophy. Early and mild stages of these diseases may not be advanced enough to cause chronic elevation of carbon dioxide. As the disease progresses, patients may develop and tolerate elevated levels of carbon dioxide for months to years. Renal compensation (retention of bicarbonate) will result in near-normal pH values, and the pulmonary symptoms of the underlying disease, such as dyspnea on exertion, predominate. However, because of limited respiratory reserves, an acute event such as infection can lead to an acceleration of the symptoms, with worsening shortness of breath and a struggle to maintain adequate ventilation. Those with an airway disease such as COPD, asthma, and bronchiectasis may also complain of increasing wheezing, cough, and sputum production. The sputum may change color from clear to yellow or green, and this reflects purulence from an acute bacterial bronchitis.
The pathophysiology of acute respiratory failure or an acute decompensation of chronic respiratory failure depends on the cause. Patients with underlying airway disease (COPD, asthma, bronchiectasis) may show worsening airway wall edema and inflammation, bronchospasm, and plugging of the airway lumen with thick secretions. This increases airway resistance and the work of breathing, which in turn increases CO2 production. Ventilation/perfusion mismatch occurs, causing further deficits in gas exchange and worsening hypercarbia and hypoxemia. Eventually, as the work of breathing continues to be excessive, respiratory muscle fatigue involving the diaphragm, chest wall, and accessory muscles occurs. This further reduces alveolar ventilation and causes the CO2 to rise. If the rise of the CO2 is rapid, renal compensation that takes many hours may not prevent a precipitous fall in the pH. In patients with chronic respiratory failure, preexisting buffering by renal compensation is somewhat protective and a less severe and precipitous fall in PCO2 results.
Other causes of acute respiratory failure include conditions that result in alveolar flooding with edema, blood, or water. Acute pulmonary edema, diffuse alveolar hemorrhage, and drowning are examples of these 3 mechanisms. In such patients, alveolar ventilation can be so compromised that gas exchange and elimination of CO2 is inadequate. In patients with chest wall trauma, pain, and splinting, minute ventilation and, hence, alveolar ventilation are compromised. Similarly, a large amount of blood (hemothorax) or air (pneumothorax) in the pleural space reduces the amount of functioning lung and can result in hypoventilation. One type of trauma-related severe chest wall deformity, with a high mortality rate of up to 40% when associated with lung contusion, is flail chest, defined as the fracture of 3 or more ribs in 2 or more places. In spontaneously breathing individuals, that diagnosis can be made by inspecting the chest wall. The flail segment is displaced inward rather than outward during inspiration. This paradoxical motion may not be seen in sedated, mechanically ventilated patients until positive pressure ventilation is discontinued.
In patients with acute life-threatening respiratory acidosis due to lung or chest wall causes, patients will complain of severe dyspnea, and the physical examination will show signs of respiratory distress. The patient may be diaphoretic and show nasal flaring, intercostal retractions, and the use of accessory and/or abdominal muscles of respiration. Tachypnea, tachycardia, hypertension, or hypotension may be present. Pursed lip breathing may be present, especially in patients with COPD. Patients may also show respiratory paradox, a sign of excessive respiratory load. This occurs when the chest wall and diaphragm are moving in the opposite directions. Inspection will show the abdominal wall moving in rather than out as the chest wall expands.
As a result of hypercarbia and acidosis, patients may develop CO2 narcosis with mental status changes that range from confusion to frank coma. Terms that have been used to describe patients with CO2 narcosis include disorientation, confusion, incoherence, somnolence, obstreperousness, combativeness, and bewilderment. Initially, patients show agitation and anxiety, and as respiratory distress increases, the level of alertness decreases. Apathy leads to drowsiness and then coma. Focal neurologic signs may also be present, and miosis, myoclonic jerks, sustained myoclonus, seizures, papilledema, and a flapping tremor of the hands (asterixis) can occur.
Patients with acute respiratory failure that results from excessive opioid and sedative use in the hospital setting, illicit drug use, and suicide attempts will not likely show signs of respiratory distress. Central nervous system respiratory center depression and slowing of the respiratory rate can result in hypercarbic respiratory failure and depressed mentation. This can lead to frank coma either from the drug or from respiratory acidosis. In patients who become apneic as a result of catastrophic CNS damage, the respiratory arrest and hypercarbic acidosis will demand immediate attention with respiratory support, and signs of respiratory failure are not relevant. Other causes of respiratory arrest that occur secondary to shock from sepsis, volume loss, or cardiac events are similar in this respect.
Chronic obstructive pulmonary disease, characterized by progressive airflow obstruction due mainly to cigarette smoking and cooking with fossil fuels, is the third leading cause of death in the United States. It is projected to reach a similar level worldwide by 2020. An exacerbation of COPD is defined as an acute event characterized by worsening of the patient’s symptoms (usually dyspnea, cough, and sputum production) that is beyond the normal day-to-day variation.1 Mild exacerbation can be treated with short-acting bronchodilators; moderate exacerbation can be treated with short-acting bronchodilators, antibiotics, and/or oral corticosteroids; and severe exacerbation may require hospitalization or emergency visits, and may be associated with acute respiratory failure.2 Exacerbation of COPD is the most common reason for admission to the hospital for hypercarbic respiratory failure. Often, the sputum becomes purulent, suggesting underlying bacterial infection. Approximately 70% to 80% of exacerbations can be attributed to respiratory infections, and coinfection with multiple organisms can worsen the outcomes. Viruses such as rhinovirus account for approximately 60% of acute exacerbations. Common bacteria that have been implicated include Haemophilus influenzae, Streptococcus pneumoniae, and Moraxella catarrhalis. The remaining causes are likely due to environmental exposures, but in many instances, the inciting event is unknown. The underlying pathophysiology of COPD is V̇/Q̇ mismatch, and during an exacerbation, this abnormality worsens. As a result, hypoxemia ensues, and with severe degrees of mismatch, hypercarbia results because of increased dead space ventilation. The respiratory failure of COPD is associated with a high risk for mortality with reported rates from 4% to 30%. Exacerbations are associated with poor long-term outcomes and a high risk of hospital readmission for those who survive.
Patients with COPD often have one or more comorbidities, such as congestive heart failure, coronary artery disease, diabetes mellitus, and gastrointestinal bleeding.3 Exacerbations of COPD can be precipitated or caused by one of the comorbidities of COPD. In addition, COPD patients have a higher rate of bacterial pneumonia, especially those who are chronically using inhaled corticosteroids, one of the mainstays of treatments for severe COPD. Also, a high degree of suspicion for pulmonary embolic disease is necessary, especially when the clinical presentation lacks the typical infectious features of a COPD exacerbation, such as a viral prodrome or increased sputum production.4 A reduction in previously elevated levels of PaCO2 could suggest pulmonary embolus rather than a COPD exacerbation.5 A prior history of a COPD exacerbation within the last year is the best predictor of new exacerbation and the odds ratio of another exacerbation6 grows rapidly with each subsequent attack.7
All patients with a COPD exacerbation should have a with admission chest radiograph.8 New infiltrates may signify underlying pneumonia and findings of congestive heart failure may be present. The use of brain natriuretic peptide (BNP) or N-terminal pro BNP (NT-proBNP) can be helpful in identifying left ventricular dysfunction, and some studies have suggested it is a predictor of subsequent mortality,9,10 and levels can help guide therapy.11 Sputum Gram stain and culture are not recommended for routine use. However, for those who have had a recent hospitalization or who have failed outpatient antibiotic treatment, this study is indicated as Gram-negative organisms such as Pseudomonas are reported. Arterial blood gases (ABGs) are almost always needed, but in some hospitals, this test has been replaced by venous blood gas (VBG) analysis coupled with pulse oximetry.12,13 Atrial and ventricular tachycardias should be anticipated, and atrial fibrillation and atrial flutter are also observed. Treatment of an acute exacerbation of COPD should target relief of symptoms, improvement in oxygenation, and reversal of respiratory acidosis, if present.14 Supplemental oxygen can be offered with nasal prongs or a 24% or 28% venturi mask to assure controlled oxygen delivery to maintain the oxygen saturation (SpO2) above 92% and the PO2 above 55 mmHg. Limiting oxygen delivery to achieve these targets provides adequate tissue oxygenation and avoids the risk of worsening hypercarbia and respiratory acidosis, as high-flow oxygen therapy can induce hypercapnia in susceptible patients during exacerbations of COPD.15 This can occur as a result of worsening V̇/Q̇ mismatch. Medical management should include a short-acting β agonist coupled with a short-acting anticholinergic, as some patients may achieve more benefit when both are offered together8 (Level of Evidence [LOE] C).2 Methylxanthines such as aminophylline and theophylline, once popular, are not helpful and potentially dangerous (LOE B).2 Corticosteroids are indicated, as they improve lung function and may reduce length of stay and early treatment failure. Oral corticosteroids can be as effective as intravenous corticosteroids, if tolerated, and most patients can be treated with a short course of low-dose systemic corticosteroids, such as 40 mg of prednisone daily for 5 days16,17 (LOE A).2 High doses of corticosteroids (> 240 mg of methylprednisolone/day) in such critically ill patients have been associated with worse outcomes and more frequent adverse effects, such as diabetes and fungal infection, than lower doses (average daily dose of 96 mg/d).18 Based on 1 study of ventilated patients in the intensive care unit (ICU), the use of antibiotics is important18 (LOE B).2 In that study, ofloxacin, 400 mg daily for 10 days, reduced the time on the ventilator, decreased the length of hospital stay, and improved overall mortality (number needed to treat [NNT] = 6; absolute risk reduction [ARR] 17.5%; 95% confidence interval [CI], 4.3–30.7; P = 0.01).19
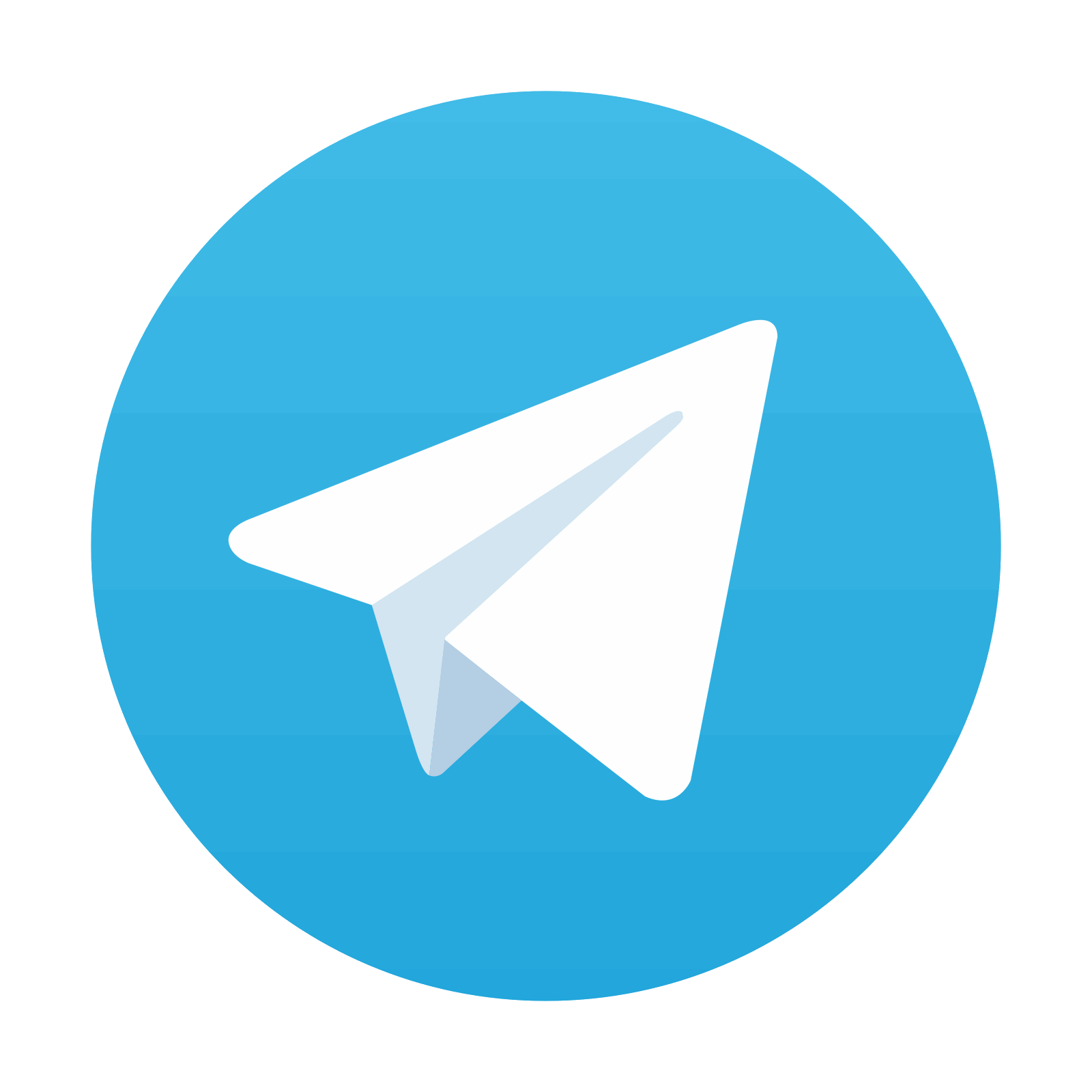
Stay updated, free articles. Join our Telegram channel

Full access? Get Clinical Tree
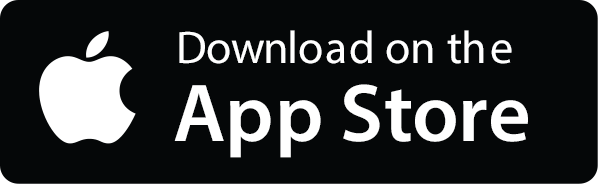
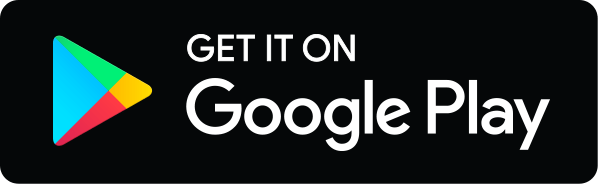