Transducers are defined as devices converting one form of energy into another. In the case of ultrasound, electrical energy is converted into mechanical (acoustic) energy. The most familiar transducer is a telephone receiver, with an earpiece that converts electrical impulses into sound waves and a mouthpiece that converts sound energy into electricity. Imaging transducers combine both functions by emitting and receiving ultrasound pulses and converting them into electrical impulses for further processing. Nonimaging continuous-wave (CW) Doppler transducers, just like the telephone receiver, have two elements: one is constantly emitting sound and the other is receiving sound. Figure 3-1 shows the anatomy of the imaging transducer.
Figure 3-1
An imaging transducer both emits and receives signals. The PZT (piezoelectric) crystal converts electrical impulses from the wire into ultrasound and vice versa. A matching layer reduces internal reflections within the probe by gradually decreasing acoustic impedance. Backing material reduces the length of the pulse by preventing after-ringing dampening effect. Acoustic lenses improve focus. The case prevents electrical shock exposure for the patient and the operator.

At the core of the ultrasound transducer (probe) is a sheet of piezoelectric material known as an active element, or simply the “crystal.” It is usually made of lead zirconate titanate, or PZT. This material will create electricity when mechanically deformed (direct piezoelectric effect) and it itself deforms when electrical voltage is applied to its surface (reverse piezoelectric effect). The ability of some natural and man-made materials to create electricity when physically deformed was discovered by the brothers Pierre and Jacques Curie in 1880 and first used to produce ultrasound in sonar to track German U-boats during World War I in France in 1917. The piezoelectric effect of PZT irreversibly disappears as temperatures rise above 360°C (Curie point), making it impossible to sterilize ultrasound transducers with heat. The PZT crystal is one-half–wavelength thick (for the speed of sound in the active element itself). Connected to the PZT crystal is a wire that transmits electrical impulses from a pulse generator to the crystal during a pulse-generation phase, and away from it to the processor, during the “listening” phase, when an electrical impulse is generated in the PZT crystal by the returning echo. The listening phase is 10 times longer than the pulse duration, so the duty factor in imaging ultrasound transducers is 0.1–1% (see Chapter 2). The transducer can be also set to emit sounds of a so-called fundamental frequency, but receive echoes with frequencies that are multiples of the fundamental one. This tissue harmonic imaging is usually performed with returning frequencies that are twice (first harmonic) or even four times (second harmonic) higher than the fundamental one. Because the harmonic frequencies are generated in the tissues themselves, the image is resistant to certain artifacts and tends to be of a better quality. Behind the active element (PZT) is a backing or damping material. Just as a guitar string continues to produce sound after being struck once by the player, the electrical impulse, once it exits the PZT, will keep on ringing, producing longer pulse durations and spatial pulse lengths with deteriorating axial resolution. The backing material works like a guitarist’s hand placed over the string, reducing the time that the PZT spends vibrating (ringing) after each electrical impulse and improving the image quality. The backing material is usually composed of tungsten-impregnated epoxy resin. CW Doppler transducers emit sound waves constantly and therefore do not require or contain any backing material (Figure 3-2).
Figure 3-2
Continuous-wave Doppler transducer has two PZT crystals. One constantly emits and the other receives signals. Element A transmits continuous ultrasound waves with frequency f1. Element B receives frequency f2 (f1 − f2 = Doppler shift). Backing material is not necessary because continuous-wave signals require no dampening.

In front of the PZT crystal is a matching layer that is a one-quarter wavelength thick. The difference in impedance results in an increase in reflection. The impedance of the matching layer is between that of the PZT crystal and the skin, in order to increase the transmission of the ultrasound from the active element into the tissues. To further reduce the impedance difference between PZT and skin, ultrasound gel is used. The impedance of the gel is less than that of the matching layer but more than that of the skin, making ultrasound transmission relatively smooth (Figure 3-1).
Wire, backing material, PZT crystal, and the matching layer are all housed in a case to protect them from the elements and to protect the patient and the operator from an electrical shock (Figure 3-3). One should never attempt to use the transducer with a cracked housing or frayed wire.
Single-crystal transducers can produce several forms of imaging, of which only M-mode and two-dimensional (2D) (with mechanical scanning) are presently in use. A and B modes are only mentioned for their historical relevance (Figure 3-4). Both A-mode and B-mode will relate the strength of the signal to distance to the boundary where that signal is produced. This strength is either represented by the height (A-mode) or the brightness (B-mode) (Figure 3-4). B-mode of the single active element transducer will produce a series of dots arranged in a line. With multiple crystal or array transducers, each line produced by the single active element will coalesce with the one formed by its neighboring element to form a 2D image (see below). Because of this relationship, 2D images are sometimes called “B-mode,” but this is technically incorrect. M-mode shows the position of the moving boundary over time, without reference to the signal’s strength (Figures 3-4 and 3-5). M-mode is presently used in echocardiography and occasionally in noncardiac chest ultrasound for the diagnosis of pneumothorax, but 2D images are the primary mode used today. Two-dimensional imaging displays are used in all portable ultrasound machines available for intensive-care unit (ICU) use and in almost all diagnostic ultrasound equipment on the market.
Figure 3-5
M-mode examination of the heart showing the position of different cardiac structures (intraventricular septum, mitral valve leaflets and inferior wall of left ventricle) plotted over time. Each 50 mm of the horizontal axis is 1 s. 1-Position of the anterior leaflet of the mitral valve at that time. Please note that brightness of the signal is of little relevance in an M-mode study.

Though 2D images can be produced by a single crystal transducer by mechanically moving the active element in a swinging motion across a scan plane (like moving a spotlight beam to see a deer in a night-time meadow), most of the modern transducers are composed of multiple active elements (Figures 3-3, 3-6, and 3-7). These so-called transducer arrays contain multiple PZT crystals with a separate wire attached to each element (Figures 3-3, 3-6, and 3-7). The electronic circuitry allows for each element to be activated separately in a specifically designed order. Arrays of active elements can be placed in a straight line (linear array), in an arc (convex or curved arrays), in concentric circles (annular arrays), or even in a checkerboard pattern (three-dimensional arrays) (Figures 3-3, 3-6, and 3-7). According to the sequence of element activation, transducers can also be divided into sequential arrays or phased arrays.
Figure 3-6
Mechanical scanning and phased-array probes offer a large acoustic footprint in the far field through a small window. They are common in cardiac ultrasound where the window is limited by intercostal spaces. In addition, phased-array probes offer electronic steering (sweeping) and focusing of the ultrasound beam. The operator is capable of selecting single or multiple focal points and the width of the sweep. A lack of moving parts also makes phased-array probes more reliable and durable.

Figure 3-7
Two-dimensional imaging. Linear sequential arrays consist of multiple PZT crystals (elements) arranged in a line. Each one is connected to a separate wire. Elements are activated in groups from one end of the transducer to the other. A similar arrangement is present in a convex array, but the elements are arranged in a curve, giving this type of the transducer a wider view (larger footprint) in the far field. Elements in curved-array probe can be activated individually or in small groups.

With sequential-array probes, groups of PZT crystals, usually arranged in linear or curved arrays, are fired in a sequence starting from one end to the other, 5–10 elements at a time, with each group firing immediately after its neighboring group. This is similar to a “wave” in a baseball stadium. When the activation sequence reaches the opposite end of the transducer, the process starts again. Linear sequential-array probes produce images only of the size of the transducer with a fixed focus, since each crystal in the array has its own focal zone and there is an inability to steer the beam. The image produced has a uniquely characteristic square shape (Figures 3-7 and 3-8). The linear-array probes are more common in vascular ultrasound. Convex sequential-array probes tend to be larger, with a fixed focus (for the same reason), but the image has a sector shape with a blunted top. Convex arrays have the advantage of a large near field and even larger far field and are used extensively in abdominal ultrasound, where large images are necessary (Figures 3-7 and 3-9).
Most transducers that critical care physicians will encounter will be linear, convex, or phased arrays. In phased-array probes, both beam steering and focusing is achieved electronically by sequencing the PZT crystal activation. Each active element is activated with an approximately 10-ns delay in a pattern created by a beam former in the ultrasound machine. Each PZT crystal in the array receives a signal in that predetermined pattern. There is also a similarly spaced delay in signal reception by the ultrasound machine. If the delay pattern is from the left to the right of the array, the beam is steered to the left. If the delay pattern is from right to left, the beam will be steered to the right. This will create the sweeping necessary to form 2D images without moving the active element (Figures 3-6 and 3-10).
The entire sweep from one side of the probe to the other will produce one imaging sector or frame. If the activation pattern is parabolic, the beam will be focused on a particular depth and the combination of patterns will produce both focusing and steering. Phased-array probes, regardless of how the active element patterns are arranged, are now the predominant probes used in echocardiography and are being used more frequently in vascular and general ultrasound.
The quality of the detail of the 2D image (spatial resolution) produced by an array probe depends on the number of separate ultrasound beams (lines) generated by the probe and the width of the sector necessary to produce the image. The line density, therefore, will depend on the number of the PZT crystals in the array probe and the sector width. To visualize the entire heart, for example, one will need a wider sector than the one necessary to visualize just the mitral valve. A cross section of the aorta will need a wider sector than a cross section of the carotid artery. The more lines per sector, the higher the line density, and the better the image quality and spatial resolution will be. Shallow images allow higher ultrasound frequency with better axial resolution per line and improved overall image quality (spatial resolution) (Figure 3-11 and Table 3-1). One complete sweep of either a mechanically or electronically steered beam will produce one frame of the 2D image. The sequence of the frames will create a video clip that can be observed or recorded by the operator. The number of frames per unit time is the temporal resolution of the exam. For continuous motion to be perceived as continuous, a minimal frame rate of 15 frames per second is necessary to provide a minimally acceptable temporal resolution. The more frames per second, the higher the temporal resolution. For a moving structure, like the heart, the higher the temporal resolution, the more real time is the image. The number of frames is limited by the time necessary to create a single frame. In any imaging, the previous pulse has to be received by the transducer and processed before the next one can be generated (Figure 3-11).
Figure 3-11
Probe A produces more ultrasound beams (lines) than probe B, with a resulting improvement in image quality (better spatial resolution). Probe C produces as many lines as probe A, but a wider sector decreases line density and degrades spatial resolution. Multifocusing requires more pulses per line and improves spatial resolution.

Improved 2D Resolution | Depth | Sector Width | Line Density | Focusing |
Spatial | Shallow | Narrow if the line density increases (zooming in) | High | Multiple |
Temporal | Shallow | Narrow if the line density is unchanged | Low | Single |
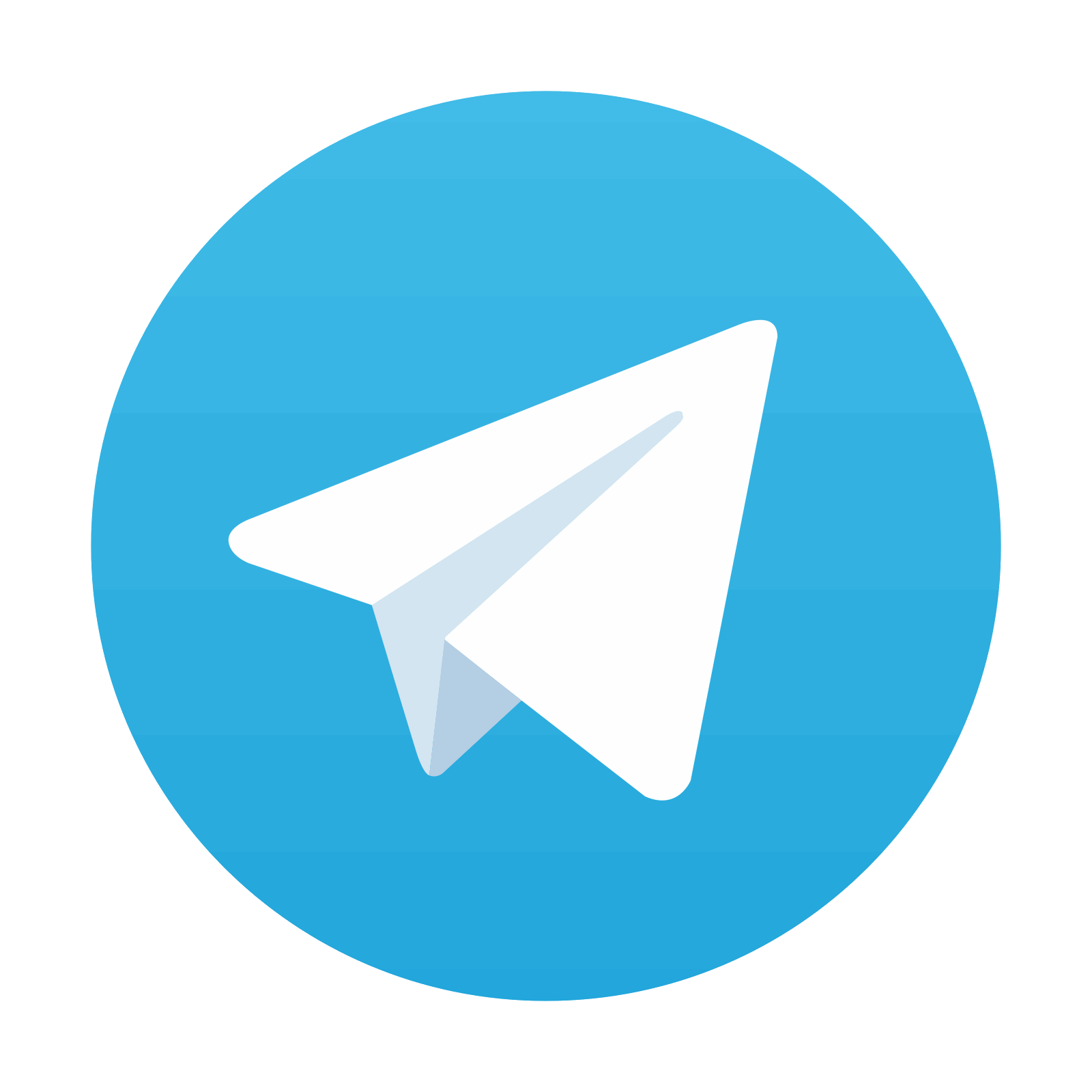
Stay updated, free articles. Join our Telegram channel

Full access? Get Clinical Tree
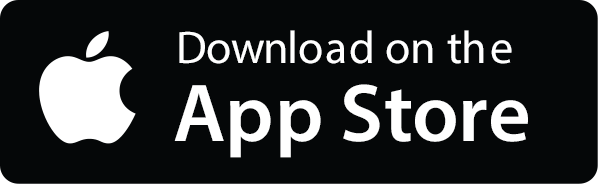
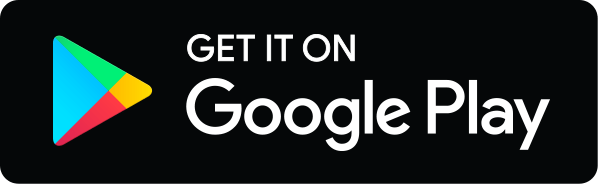
