TOXICOLOGIC EMERGENCIES
KATHERINE A. O’DONNELL, MD, KEVIN C. OSTERHOUDT, MD, MSCE, MICHELE M. BURNS, MD, MPH, DIANE P. CALELLO, MD, AND FRED M. HENRETIG, MD
PEDIATRIC POISONINGS
Poisoning represents one of the most common medical emergencies encountered by young children and accounts for a significant fraction of emergency department (ED) visits in the adolescent population.
Estimates of poisoning episodes annually in the United States range in the millions. Poisonings may be unintentional or intentional. Unintentional or exploratory exposures to poisons make up 80% to 85% or more of all reports, whereas intentional poisonings comprise the other 10% to 15%. Persons in this latter group have much higher rates of treatment in the ED, hospitalization, and intensive care. Among children 5 years and younger, most poisoning exposures are related to exploratory behavior. Although less common, the physician must also consider the possibility of environmental exposures, therapeutic errors, suicide attempts in children, and neonates exposed to toxicants in utero. Child abuse by poisoning, while rare, should be suspected in patients outside the typical age for self-poisoning (<1 year, 5 to 11 years of age), when multiple agents or illicit drugs are involved, when siblings present with similar syndromes, and in cases of massive ingestions in a young child.
The exploratory ingestion of a drug or chemical by a toddler represents a complex interplay of host, agent, and environmental factors and may be considered a subset of the modern traumatic injury model. In this model, each factor contributes, more or less, in a given context to the probability of the injury occurring. Some children are more at risk because of peak age of 1 to 4 years, male gender, temperament that leans toward hyperactivity, and increased finger–mouth activity and/or pica. Some agents are more culpable because of ease of access, attractiveness/palatability, and toxic potential. Two classic examples are iron tablets, which may look like candy, are widely available, and are toxic in significant overdose; and mouthwash, which has a bright color, as well as a pleasant taste and smell, is often packaged in large volumes without child-safety caps, and may have surprisingly high ethanol content (15% to 25%). Typical environmental factors include an acute stressor, such as a recent move or new baby in the household, or more chronic issues, such as parental illness/disability. The young child’s exploratory encounter with a poison should not be viewed as an “accident,” as the combination of child, agent, and environmental factors may lead predictably to the statistical likelihood of toddler ingestion. Pediatricians have led the way in poisoning prevention strategies by modifying these risk factors with traditional anticipatory guidance and by spearheading the lobby for child-safety caps on particularly dangerous medications and household products. These efforts resulted in a dramatic decrease in childhood poisoning morbidity and mortality since the 1970s, however recent data suggests a worrisome trend. As prescribing patterns in the adult population have created an increased availability of potentially hazardous medications, there has been a clear increase in serious pharmaceutical exposures in young children. As such, pediatric poisonings continue to occur and demand the emergency physician’s attention.
The scope of toxic substances involved in poisonings is broad, requiring a wide range of knowledge. Tables 110.1 and 110.2 review the categories of substances most commonly reported in human exposures in the United States for the year 2012. Table 110.3 presents the 10 most common toxic exposures involved in human deaths for the year 2012. The former listing much more closely approximates the profile of pediatric poisonings, whereas the latter is more typical of intentional adolescent and adult exposures. The most important difference between the pediatric and the adult profile by type of agent is in the higher percentage of cases in which psychopharmacologic drugs (sedatives, tranquilizers, and antidepressants) cause poisoning in adults and the much higher frequency of exposures to household and personal care products and plants in children.
There are seven basic routes of poison exposure: oral (ingestion), ocular, topical, inhalational, transplacental, parenteral, and by envenomation. Poisonings may result from acute or chronic exposures. Most poisonings treated in EDs are acute, and the patients are typified by the curious child who gains access to a medication or household cleaning product, or the adolescent who takes a massive number of pills in a fit of despair. Chronic poisoning refers to toxicity which develops over time as a substance accumulates in the body, and is best exemplified by environmental exposure to lead or other heavy metals. Chronic pharmaceutical toxicity also occurs. Examples of this pattern of poisoning include acetaminophen hepatotoxicity in infants and small children after repeated supratherapeutic dosing or aspirin poisoning in older adults with excessive dosing or renal impairment. Chronic toxicity can be a challenging diagnosis because the source is not always apparent, the toxicity is not always clear, and the toxic process is not often obvious until serious clinical derangements occur.
GENERAL APPROACH TO THE POISONED CHILD
Following the analogy between unintentional poisoning and traumatic injury, a similar model may be used in formulating a management approach. The poisoned patient often represents an acute-onset emergency with a broad spectrum of multiorgan system pathophysiology that shares many features with the multiple trauma patient. In essence, poisoning might be viewed as a multiple chemical trauma. The concept of a brief window of opportunity to make critical diagnostic and management decisions is likewise analogous. One may conceptualize a management approach that attempts to prioritize critical assessment and, at times, simultaneous management interventions (Table 110.4). The initial phase (or primary survey) addresses the traditional airway, breathing, and circulation (ABCs) of airway securement and cardiorespiratory support, with a slight additional emphasis on emergent toxicologic considerations. The more specific evaluation and detoxification phase (or secondary survey) is aimed at simultaneously initiating generic treatment while assessing the actual extent of intoxication (in cases of known or presumed exposures) and/or identifying the actual toxicants involved (in unknown but highly suspected intoxications).
TABLE 110.1
SUBSTANCES MOST OFTEN REPORTED IN HUMAN EXPOSURES
Initial Life Support Phase
The general approach to recognition and support of vital airway and cardiorespiratory functions (or ABCDs) is well known to most readers and is covered in detail in Chapter 1 A General Approach to Ill and Injured Children. In the context of the poisoned child, a few points deserve special emphasis. In addition to the usual signs of airway obstruction, the physician must pay special attention to evidence of disturbed airway protective reflexes, or to signs of airway injury as with a caustic ingestion. Many poisoned patients will vomit, and some may be administered charcoal, which poses an aspiration risk. Elective endotracheal intubation (Chapter 3 Airway) may thus be indicated at a slightly lower threshold in this context than in another child with comparable central nervous system (CNS) depression.
TABLE 110.2
SUBSTANCES MOST OFTEN REPORTED IN PEDIATRIC EXPOSURES <5 YEARS OF AGE
TABLE 110.3
TOXIC EXPOSURES ASSOCIATED WITH THE MOST DEATHS
It is also particularly important to anticipate imminent respiratory failure in the deeply comatose poisoned child. Cyanosis and overt apnea are late findings with progressive drug-induced medullary depression. Thus, clinical or laboratory assessment of early ventilatory insufficiency is critical in such patients to avoid the chaos of a precipitous respiratory arrest. Likewise, it is far easier to establish intravenous (IV) access in a child with normal circulatory status than in a child in shock; early efforts to obtain a secure IV line in symptomatic overdose patients are thus well worth the time and effort.
After securing the airway, ensuring effective breathing, and supporting circulation, it is important to evaluate poisoned patients for neurologic “disability,” and the need for empiric “drug” treatment, and emergent “decontamination.” Level of consciousness may be assessed rapidly with a semiquantitative scale such as the Glasgow Coma Scale or the AVPU (spontaneously alert, response to verbal stimulation or pain, or unresponsive) scale. Pupillary size and reactivity may be quickly noted. Rapid changes in mental status are common in serious intoxications and may herald precipitous cardiorespiratory failure.
Empiric drug treatment is warranted for most symptomatic poisoned children with altered mental status. All such patients may initially be given humidified oxygen and their blood oxyhemoglobin saturation monitored, if possible, by pulse oximetry. If available, rapid bedside blood glucose testing may be used; if low, or not readily available, a trial dosage of 0.25 to 1 g per kg glucose as 10% to 25% solution should be infused. It should be noted that drug- or toxicant-induced hypoglycemia does not present uniformly with coma or seizures. Almost any neuropsychiatric sequelae of hypoglycemia may predominate, including aphasia; slurred, dysarthric speech; and focal neurologic signs. Adrenergic signs, such as diaphoresis and tachycardia, are not uniformly present. Hypoglycemia is a complication seen in ingestions of ethanol, oral hypoglycemics, β-blockers (BBs), salicylates, and, of course, insulin injection. As basic as this intervention seems, in our experience, it is still one of the most often missed (or more accurately, delayed) critical treatments in the management of the poisoned patient. Thiamine (100 mg IV), although routinely administered to adult overdose patients who receive hypertonic glucose to obviate precipitating Wernicke encephalopathy, is not generally necessary in the pediatric population. Perhaps it should be considered in adolescent patients who may be thiamine deficient secondary to eating disorders, chronic disease (e.g., inflammatory bowel disease), or alcoholism.
TABLE 110.4
GENERAL APPROACH TO THE KNOWN OR SUSPECTED INTOXICATION
Lastly, empiric naloxone therapy is just as important in potentially poisoned toddlers with altered mental status as it is in adults. As opioid prescriptions in the adult population have increased in recent years, so too has the availability of these agents to young children. Many households contain a variety of oral opioid analgesic agents, as well as cough medicines (codeine, dextromethorphan), antidiarrheal agents (paregoric, diphenoxylate), and partially naloxone-responsive antihypertensive agents such as clonidine. In addition, the possibility of exploratory ingestion of a “stash” of illicit opioids does exist. Thus, naloxone should be used as a therapeutic/diagnostic trial when there is a reasonable possibility that altered mental status is drug induced. Previous recommendations have based dosing on weight (e.g., 0.01 to 0.1 mg per kg); however, many authorities now prefer a unified pediatric dose of 0.4 to 2 mg for acute overdose patients of all ages (outside the neonatal period). Such an approach conceptualizes naloxone dosing as based on total narcotic load and number of opioid receptors that require competition for binding sites. In general, this latter approach is easier to remember and has not been associated with complication in the ED. Adolescent patients with a strong clinical picture for opioid intoxication (without habituation) may receive 2-mg bolus doses every 2 minutes, up to a total dose of 8 to 10 mg, before abandoning hope of benefit because several congeners of morphine (e.g., propoxyphene, illicit fentanyl derivatives, pentazocine, oxycodone, methadone) may require such large doses. If chronic abuse is suspected, lower initial doses (0.2 mg or less) are warranted. Administration of flumazenil to adolescents exhibiting depressed consciousness after an unknown drug overdose is not recommended as it may precipitate seizures and life-threatening benzodiazepine withdrawal (see “Central Nervous System Sedative-Hypnotics” section).
The rationale for decontamination (Decontamination) of the poisoned child is discussed in the next section. This treatment phase may begin urgently, after or in concert with attention to the ABCDs. At times, a decision to perform gastric decontamination through the preferred technique can be made almost immediately upon presentation and, if so, should be instituted as soon as possible, taking into account the patient’s clinical status and the number of hands available to assist in management. For example, a toddler with coma, shock, and massive hematochezia who is rushed into the ED by the rescue squad—and for whom there is witnessed or strong circumstantial evidence of massive iron overdose—requires a concerted team effort directed toward resuscitation, stabilization, and urgent gastric decontamination. However, an asymptomatic adolescent who ingests 10 g of acetaminophen 30 minutes before arrival at the ED may be fully evaluated in a timely but orderly manner (as outlined in the next section) and considered for less emergent gastric decontamination—in this case, possibly an oral dose of activated charcoal. Significant dermal or ocular exposures require immediate copious lavage, and precautions should be taken to protect the healthcare providers tending to the patient from exposure.
At the completion of this initial life support phase, the poisoned patient should have been assessed for compromise of vital airway and cardiorespiratory function and for global neurologic status and should have had resuscitative measures instituted. Patients with significant altered mental status have been critically evaluated for respiratory status, have had IV access secured, and have had therapeutic trials of oxygen, glucose, and naloxone. Other advanced life support interventions such as anticonvulsants or antiarrhythmics have been instituted as necessary. Consideration of decontamination options has begun.
Evaluation and Detoxification Phase
History
A brief and focused historical evaluation should be addressed as soon as the life support phase has been completed. The primary goal is to determine the potential severity of the exposure. This assessment requires poison and patient-related data alike.
For a known or highly suspected toxic exposure, an attempt is made to estimate the total amount ingested (number of pills missing, ounces left in the bottle, dosage of pills, concentration of alcohol, and so forth). The best estimate of time elapsed since ingestion is also sought. Parents should be questioned regarding early symptoms noted at home or en route to the ED and any treatments administered before arrival. Certain underlying medical conditions may be relevant (e.g., glucose-6-phosphate dehydrogenase [G6PD] deficiency for mothball ingestions); thus, any significant medical history should be noted.
Often, children who are poisoned do not come to the ED with a clear history of exposure followed by onset of symptoms. Rather, they develop signs and symptoms that mimic other diseases and give no history of toxic exposure. Thus, the ED staff must always consider the possibility of ingestion when treating young children.
General historical features that suggest the possibility of poisoning include (i) acute onset; (ii) age range of 1 to 5 years or adolescence; (iii) history of pica or known exposure to a potential toxicant; (iv) substantial environmental stress, either acute (e.g., arrival of a new baby, serious illness in a parent) or chronic (e.g., marital conflict, parental disability); (v) multiple organ system involvement; (vi) significant alteration in level of consciousness; and (vii) a clinical picture that seems especially puzzling.
Certain family and social history variables are also important. Medications used by other household members, particularly new medications introduced into the home environment by virtue of recent illnesses or visits from grandparents and other relatives, are a common source of ingested drugs. Changes in routine and large family gatherings (e.g., holiday parties, moving to a new home) are particularly risky occasions for decreased parental supervision and new (or less carefully guarded) potentially toxic medications or household products. Although often difficult to obtain, the history of illicit drug use, manufacture, or distribution in the child’s environment (the “drug-endangered child”) significantly increases the risk of serious outcomes from a poison exposure as well.
Physical Examination
The focused physical examination should begin with a reassessment of vital functions and complete recording of vital signs, including core temperature. With secure airway and cardiorespiratory function confirmed, the examination should then focus on the central and autonomic nervous systems, eye findings, changes in the skin and/or oral and gastrointestinal (GI) mucous membranes, and odors (see Chapter 45 Odor: Unusual) on the breath or clothing of the patient. These features represent those areas most likely affected in toxic syndromes and, when taken together, often form a constellation of signs and symptoms referred to as toxidromes (Tables 110.5 and 110.6). Such toxidromes may be so characteristic as to provide guidance for early therapeutic management before precise historical or laboratory confirmation of a specific exposure is available.
Laboratory Evaluation
Laboratory studies may be helpful in confirming diagnostic impressions or in demonstrating toxicant-induced metabolic aberrations. However, there is no “tox panel” that is uniformly helpful or necessary. Most poisonings can be managed appropriately without extensive laboratory studies, and in particular, the reflex ordering of rapid overdose toxicology “screens” has rarely been found to be helpful in acute patient management. They have important, nonemergent roles (e.g., in resolving medicolegal issues or considering drug-induced causes of behavioral changes in a psychiatric patient). In toddlers with a known or strongly suspected specific ingestion, rapid drug screens are rarely indicated. In the adolescent intentional overdose patient who is not critically ill or who does not have a particularly puzzling clinical picture, the drug screen again is rarely helpful, although the finding of an unexpected toxic level of acetaminophen (which may have been omitted in the history) may impact management, and some authors recommend that quantitative acetaminophen levels (in lieu of “tox screens”) be sought in all such patients.
TABLE 110.5
CLINICAL MANIFESTATIONS OF POISONING
The labor-intensive comprehensive urine drug screen may be useful for patients who are seriously ill with an occult ingestion or for the occasional intentional overdose adolescent patient whose clinical picture does not fit with the stated history. Often of greater help is the critical interpretation of routine measurements of serum chemistries, blood gas analysis, and osmolality in patients with altered mental status. The presence of hypoglycemia or aberrations of serum electrolytes may provide crucial information about the poisoned patient. In certain circumstances, tests of liver or renal function, urinalysis, creatine phosphokinase levels, and other select tests may be useful. Metabolic acidosis with a high anion gap is found in many clinical syndromes and toxidromes, reflected by the often-cited mnemonic MUDPILES, for methanol and metformin; uremia; diabetic and other ketoacidoses; paraldehyde; Isoniazid (INH), iron, inborn errors of metabolism and massive ibuprofen; lactic acidosis (seen with hypoxia, shock, carbon monoxide, cyanide, and many drugs that cause compromised cardiorespiratory status or prolonged seizures); ethylene glycol; and salicylates or seizures. Differences between calculated and measured serum osmolarity (calculated = 2 [serum Na mEq per L] + blood urea nitrogen [BUN] mg per dL ÷ 2.8 + glucose mg per dL ÷ 18; with normal osmolarity ∼290 mOsm per kg) may suggest intoxication with ethanol, isopropanol, or more rarely in pediatric patients, methanol or ethylene glycol. Blood collection tubes containing ethylenediaminetetraacetic acid (EDTA) should not be used to send samples to the laboratory because the osmolal gap will be falsely elevated.
TABLE 110.6
TOXIDROMES
TABLE 110.7
FREQUENTLY USEFUL QUANTITATIVE TOXICOLOGY TESTS IN PEDIATRIC PATIENTS
An immediate determination of quantitative levels is helpful in making management decisions for some drugs, and these are outlined in Table 110.7. Furthermore, many important causes of coma and altered vital signs are not detected on even the most sophisticated “comprehensive” toxicology panels (which are usually biased toward psychoactive medications and illicit drugs). An overview of such agents is presented in Table 110.8. An electrocardiogram (EKG) should be performed in all seriously ill patients in whom poisoning is being considered. Detectable conduction delays (prolongation of the PR, QRS, and/or QT intervals) may provide diagnostic direction and impact management by predicting imminent life-threatening cardiac rhythm disturbances.
TABLE 110.8
IMPORTANT DRUGS AND TOXICANTS NOT DETECTED BY MOST DRUG SCREENS
Assessment of Severity and Diagnosis
At this juncture, most intoxicated patients may be readily stratified by specific toxicant or category of drug(s) ingested and some judgment made as to the potential or current severity of the exposure. For some children, clinical features of a complex illness of acute onset may suggest intoxication without a specific history of such ingestion. In a few cases, some laboratory confirmation of clinical suspicion will be available on an immediate basis. Using all the clinical clues available and with some familiarity of the “toxidrome” approach to differential diagnosis as detailed previously (Tables 110.5 and 110.6) and, at times, with help from the laboratory, the emergency physician must now establish a working diagnosis and proceed with consideration of options for specific detoxification.
Specific Detoxification
Again, the proviso that the patient be continually reassessed and managed for impaired vital function is addressed. All decisions about further decontamination and/or specific antidotal therapy involve a complex interplay between the toxicant(s) ingested and the patient’s condition.
GI Decontamination. The effort to “get the poison out” has long been a mainstay of the traditional discussion of toxicologic management. However, gastric emptying measures have fallen out of favor in recent years, and the routine use of activated charcoal as a poison adsorbent has been subjected to increased academic scrutiny. Unfortunately, young children have been underrepresented in clinical studies of GI decontamination. It is likely that as further research is conducted, particularly as directed toward the pediatric population, current dogma regarding optimal GI decontamination will evolve. For the sections that follow, several appropriate techniques for gastric decontamination are reviewed, all of which may be useful under certain circumstances. An approach to the overall decision process in a given patient is then offered.
Simple Dilution. Dilution may be indicated only when the toxicant produces local irritation or corrosion. Water or milk is an acceptable diluent. Dilution for caustic agents is controversial; dilution may be used in the first few minutes after an exposure but only if there is no evidence of airway compromise or significant abdominal pain/vomiting. For drug ingestion, however, dilution alone should not be used because it may increase absorption by increasing dissolution rates of the tablets or capsules or it may promote more rapid transit into the lower GI tract.
Gastric Emptying. The goal of gastric emptying is to rid the stomach of remaining poison to prevent further local effect or systemic absorption. The utility of gastric emptying diminishes with time and is most effective if done early after ingestion when unabsorbed drug is still present within the stomach (operationally, within the first 30 minutes to 1 hour). In certain circumstances, such as the delayed gastric emptying accompanying intoxication with anticholinergic drugs, benefit may be noted longer after ingestion. Induced emesis (with syrup of ipecac) was once a favored means of gastric emptying, but the American Academy of Pediatrics no longer recommends that syrup of ipecac be used routinely for the poisoned patient in the home or healthcare facility. This was in response to inconsistent data regarding decreased drug absorption, no evidence that it changes clinical outcomes, potential for abuse in vulnerable patients (those with eating disorders, Munchausen syndrome by proxy), and the concern that it may delay time to administration of other more effective therapies.
An alternative to ipecac-induced emesis for emptying the stomach is gastric lavage. This procedure has very limited indications and is usually reserved for patients who have ingested a potentially life-threatening amount of poison, in cases where the procedure can be performed safely very early after ingestion and charcoal alone is not believed to be adequate. To carry out a satisfactory lavage, the patient should be on his or her left side, head slightly lower than feet, and the largest orogastric lavage tube that can reasonably be passed should be used (e.g., 24F orogastric tube for a toddler, 36F orogastric tube for an adolescent). A smaller caliber nasogastric (NG) tube is sufficient only for some liquid toxins. Gastric contents should be aspirated initially before any lavage fluid is introduced. Normal saline aliquots of 50 to 100 mL in young children and 150 to 200 mL in adolescents can be lavaged repeatedly until the return is clear. Like induced emesis, gastric lavage’s efficacy in reducing drug absorption has been reviewed critically in more recent studies. Again, the efficacy has been highly variable and lavage has not been demonstrated to improve outcome in poisoned patients. Several important risks are associated with gastric lavage, including oxygen desaturation, aspiration, and mechanical trauma to the oropharynx and esophagus. Gastric lavage is rarely recommended anymore but might be considered for patients presenting very early after very dangerous ingestions (of toxicants such as colchicine or arsenic). Contraindications to the procedure include caustic or corrosive ingestions, impending loss of airway protection, and the presence of cardiac arrhythmia.
TABLE 110.9
SUBSTANCES POORLY (OR NOT) ADSORBED BY ACTIVATED CHARCOAL
Activated Charcoal. Activated charcoal minimizes absorption of drugs by adsorbing them onto its molecular surface area. Charcoal administration has become the decontamination strategy of choice to prevent pediatric poisoning after toxicant ingestion and is most effective when used in the first hour after ingestion. Therefore, if activated charcoal is considered as a treatment option, quick triage of an exposed patient may be necessary to allow charcoal administration in a timely fashion. A number of notable compounds, such as iron and lithium, do not adsorb well to activated charcoal (Table 110.9). The usual dose of activated charcoal is 1 g per kg; adolescents and adults should receive 50 to 100 g. Most activated charcoal is now available premixed with water to make slurry that can be taken orally or administered by NG tube. Simply adding soda or another nonparticulate flavoring agent to the charcoal can improve palatability.
Activated charcoal was “rediscovered” by the toxicology community during the 1980s, with several studies finding its use to be superior to gastric emptying alone and, at least, equivalent to the combination of gastric emptying plus charcoal administration. The use of charcoal alone is less invasive and less likely to be associated with complications in the clinical setting than is gastric emptying. Aspiration of charcoal can be a serious concern among patients with poor airway protective reflexes, and vomiting remains the most common difficulty associated with its use. The use of an NG tube, which renders the esophagogastric sphincter patent, may also increase aspiration risk. Charcoal is contraindicated in patients with an unprotected airway or a disrupted GI tract (e.g., after severe caustic ingestion) or in patients in whom charcoal therapy may increase the risk and severity of aspiration (e.g., hydrocarbons). The use of multiple doses of activated charcoal to achieve enhanced drug elimination is addressed later in this chapter.
Catharsis. Little evidence exists to suggest that standard cathartics (sorbitol, magnesium citrate, magnesium sulfate) reduce drug absorption by speeding intestinal transit. It is also not established whether coadministration with activated charcoal minimizes the risk of constipation, and there does appear to be an increased risk of vomiting, cramping abdominal pain, and hypernatremic dehydration in young infants. As such, a single dose of premixed charcoal/sorbitol is safe for most pediatric ingestions, but should not be used in young infants. Oil-based cathartics (mineral oil, castor oil) are discouraged because they may be aspirated, increase absorption of some poisons, or unnecessarily extend the cathartic effect.
Whole Bowel Irrigation (WBI). An additional technique of cathartic GI decontamination is that of intestinal irrigation with large volumes and flow rates of a high–molecular-weight polyethylene glycol-balanced electrolyte solution such as GoLYTELY or Colyte. Typically, these solutions are not significantly absorbed nor do they exert an osmotic effect, so the patient’s net fluid/electrolyte status is unchanged. They have a long safety track record in patient populations such as infants and in those surgical patients requiring application of preoperative bowel preparation. WBI has been found to be particularly useful in pediatric iron overdoses, in which gastric lavage may be limited by tube size, the fact that metals do not bind to charcoal, and the possibility that the ingestion is not a recent one. It has been used for other metal ingestions (e.g., lead), overdoses of sustained-release medications (e.g., lithium, theophylline, bupropion, verapamil), ingested pharmaceutical patches, and ingestions of vials or packages of illicit drugs. It might also be useful in particularly massive, dangerous, and/or late-presenting overdoses for which severe toxicity refractory to standard therapies may result and for which the efficacy of gastric emptying and/or charcoal is expected to be suboptimal. The technique may be used by mouth in cooperative patients or by NG tube; the usual recommended dosing is 500 mL per hour in toddlers and 2 L per hour in adolescents and adults.
GI Decontamination Strategies
It should be apparent that no unique approach to GI decontamination of all poisoned patients is optimal in every case. Factors to be considered include the expected degree of toxicity from the drug, the physical nature of the drug, the current location of the drug within the body, and the presence of contraindications or alternatives. A risk–benefit decision must be made before the institution of any decontamination strategy.
Due to the considerations discussed above, in the patient for whom GI decontamination is appropriate, activated charcoal and WBI are the favored procedures. Ipecac-induced emesis is no longer endorsed as appropriate home first aid for poisoning, and is not favored in the ED. Gastric lavage still has some role, albeit confined to patients with recent ingestions of extremely toxic and potentially lethal substances, especially when those substances do not bind well to charcoal. Likewise, patients with truly massive overdoses may benefit from lavage when the charcoal dose given does not achieve the ideal charcoal-to-drug ratio of 10:1. (10 g of charcoal to 1 g of drug).
The correct technique for decontamination, regardless of method, requires that careful attention be given to prevent aspiration and anatomic trauma.
Some of the patients in question would have undergone endotracheal intubation during the initial life support phase of management, as detailed previously, or they may be intubated pre-emptively because of borderline mental status and in anticipation of their ensuing critical course. Others may be awake, alert, and cooperative, with normal airway protective reflexes, and thus be given activated charcoal without prior endotracheal intubation. The combative, agitated patient poses a dilemma and must be carefully managed on an individualized basis.
An attempt to summarize these considerations is diagrammed in Figure 110.1; however, it should be reiterated that all decisions regarding gastric decontamination involve multiple patient and toxic agent-related factors and should not be made with a “cookbook” approach.
Antidotal Therapy. Beyond the setting of acetaminophen overdose, the overall number of toxic ingestions for which a specific antidote is necessary or available is small. When a specific antidote can be used, it is vital that it be administered as early as possible and in an appropriately monitored dose. Those antidotes that should be available for immediate administration include sodium bicarbonate (tricyclic antidepressants), sodium nitrite/sodium thiosulfate or hydroxocobalamin (cyanide), atropine and pralidoxime (cholinesterase inhibitors), ethanol or fomepizole (ethylene glycol and methanol), dextrose (ethanol, salicylates, oral hypoglycemics), methylene blue (methemoglobinemic agents,), oxygen (carbon monoxide), flumazenil (benzodiazepines), pyridoxine (INH and Gyromitra mushrooms), digoxin immune Fab (digoxin), IV lipid emulsion (local anesthetic toxicity), and naloxone (opioids). Other antidotes usually do not require such urgent administration and may be given subsequent to initiation of other management modalities. Even when available, antidotes do not diminish the need for meticulous supportive care or other therapy. Indiscriminant use of antidotes without other forms of management should be discouraged. Table 110.10 summarizes a list of commonly used antidotes, suggested doses, and their indications for use. Because of its frequent use, naloxone is discussed further here.
FIGURE 110.1 Approach to gastrointestinal decontamination. aFor patients in whom the toxicant is no longer believed to be in the stomach, whole bowel irrigation might still be a valid consideration.
TABLE 110.10
SUMMARY OF ANTIDOTES
Naloxone. Naloxone, a pure opioid receptor antagonist, is one of the broadest-acting, safest, and most effective of any true antidotes now available. It is effective against all opioids. Naloxone is a synthetic congener of oxymorphone but is devoid of morphine agonist or depressant effects. It has no significant side effects in the treatment of acute overdose except narcotic withdrawal symptoms in the addicted patient. These symptoms include GI upset, tachycardia, hyperpnea, mydriasis, piloerection, yawning, rhinorrhea, diaphoresis, sialorrhea, increased blood pressure, anxiety, restlessness, discomfort, and hyperalgesia. These symptoms are not usually life-threatening to teenagers and adults but can be potentially dangerous to an infant born to an addicted mother. Withdrawal symptoms secondary to naloxone, if observed during acute overdose treatment, would be expected to last no more than 30 minutes and should generally be treated with supportive care. The serum half-life of naloxone is 1 hour; its duration of action 1 to 4 hours. Initial reversal of narcosis may then revert to coma, requiring ongoing reassessment and readministration of naloxone. There are a few case reports of other adverse effects, including hypertension, pulmonary edema, ventricular irritability, and seizures after naloxone-induced reversal of narcosis in the perioperative setting, typically in patients with underlying cardiopulmonary disease and in the presence of additional medications or anesthetic agent use.
The mechanism of action of naloxone is by competitive displacement of narcotic analgesics at central opioid receptor sites. It can be used as a diagnostic test when faced with a questionable history. Current dosage recommendations reflect the proven safety of naloxone in large doses and the necessity of such doses to reverse effects of synthetic opioids such as propoxyphene, pentazocine, oxycodone, and methadone. If severe respiratory depression is present, the initial dose should be 2 mg IV in any patient. Repeat doses may be given every 2 minutes until 10 mg has been administered for adolescent patients with suspected opioid overdose who fail to respond to the lower dosages. Of course, concomitant airway management is vital. In patients without respiratory depression, an initial dose of 0.4 to 1 mg can be used. In adolescents suspected of chronic opiate abuse, smaller initial doses (e.g., 0.05 to 0.4 mg) are warranted. Again, if there is no response but a strong clinical suspicion, 2-mg doses can be repeated up to a total of 10 mg before concluding that further dosing will be of no benefit. Naloxone can also be given intramuscularly (IM), sublingually, intranasally, or by endotracheal tube if no IV access is available.
If a patient demonstrates a response to naloxone, naloxone will have to be repeated at the effective total dose every 20 to 60 minutes. An alternative approach is to provide a continuous IV infusion; generally about two-thirds of the total reversal dose will need to be infused per hour initially, with subsequent adjustments as necessary. This is more likely to be needed in long-acting opioid overdoses.
Nalmefene and naltrexone are longer-acting opioid antagonists that may have use in some clinical situations in which a longer duration of action (4 to 6 hours for nalmefene, 24 hours for naltrexone) is deemed beneficial, such as in reversal of procedural/postoperative opioid depression or as aids in opioid detoxification programs. However, as antidotes for acute opioid overdose in the adolescent or pediatric population, their longer duration may be problematic in assessing the actual time course for resolution of clinical toxicity and/or in precipitating prolonged withdrawal symptoms in habituated patients. Nalmefene may be a useful substitute for prolonged naloxone infusions in cases for which such opioid antagonism is necessary, but little pediatric experience and few dosing guidelines for its use are currently available.
Enhancing Excretion
The procedures available for enhancing the elimination of an absorbed poison that have the greatest value are multiple-dose activated charcoal, diuresis/urinary alkalinization, dialysis, and hemoperfusion. Because some risk is involved, these measures are indicated only in those cases in which the patient’s recovery would be otherwise unlikely or in which a specific significant benefit is expected.
Diuresis/Urinary Alkalinization
Diuresis has historically been advocated in cases of poisoning with agents that are excreted primarily by the renal route. Although it is important to maintain high glomerular filtration rates in the presence of rhabdomyolysis or when chelating with agents such as EDTA, forced diuresis has limited value in the treatment of acute poisoning. Similarly, diuretic use has fallen out of favor with the possible exception of mannitol therapy for ciguatera poisoning.
Ionized diuresis takes advantage of the principle that excretion is favored when a drug is in its ionized state. Urinary alkalinization promotes excretion of salicylate (a weak acid). It may also enhance clearance of phenobarbital, chlorpropamide, and chlorophenoxy herbicides, but in these poisonings, it cannot be considered a mainstay of therapy. Urine alkalinization can be initiated with sodium bicarbonate at a dose of 1 to 2 mEq per kg per hour IV over a 1- to 2-hour period, until such time as the intoxication is resolving. Careful attention should be given to total fluid and sodium load administered, especially in patients at risk for congestive heart failure or pulmonary edema. Serum electrolytes need close monitoring; in particular, hypokalemia can interfere with the ability to achieve an alkaline urine pH and should be corrected. The rate of bicarbonate infusion can be adjusted to maintain a urinary pH of 7.5 to 8.5. Urinary acidification is never indicated because it may lead to serious side effects such as systemic acidosis and exacerbation of renal impairment in the context of myoglobinuria.
Multiple-Dose Activated Charcoal (GI Dialysis)
Several studies have shown significant increase in clearance for a number of drugs when repeated doses of 0.5 to 1 g per kg of activated charcoal are given every 4 to 6 hours. By using a nearly continuous stream of fresh charcoal that descends through the intestinal tract, a constant concentration gradient is maintained that favors the back diffusion of free drug from periluminal capillary blood into the intestinal lumen, where it may be bound immediately to the newer charcoal, so the free drug concentration in the intestinal lumen remains low. In addition, enterohepatic recirculation of some drugs may be interrupted as reabsorption from bile is prevented. To be safe and effective, this technique requires active peristalsis and an intact gag reflex or a protected airway. Common pediatric poisonings for which repetitive charcoal dosing may be considered include phenobarbital, carbamazepine, phenytoin, digoxin, salicylates, and theophylline. Cathartics, such as sorbitol, should be administered no more frequently than every third dose.
Renal Replacement Therapy
Renal replacement therapy is indicated for selected cases of severe poisoning to enhance toxin clearance and correct severe acid–base or electrolyte disturbances. High-flux hemodialysis is the modality of choice for expeditious toxin removal. Other methods such as exchange transfusion, plasmapheresis and peritoneal dialysis have little role and are much less effective in rapidly reversing the course of a given poisoning. Hemoperfusion, the process of passing blood through a dialysis circuit containing an adsorbent column, was historically used to remove higher–molecular-weight substances that could not be extracted through standard dialysis but has become obsolete since the arrival of newer “high-flux” dialysis membranes. Continuous renal replacement therapy, such as continuous venovenous hemodiafiltration (CVVHDF), also has much slower rates of toxin removal and should be reserved for the hemodynamically unstable patient who cannot tolerate conventional HD.
TABLE 110.11
A PARTIAL LISTING OF DRUGS AND THEIR PLASMA CONCENTRATIONS FOR WHICH HEMODIALYSIS SHOULD BE CONSIDERED
Acute extracorporeal removal should be considered in light of patient- and drug-related criteria. Patient-related criteria include (i) anticipated prolonged coma with the high likelihood of attendant complications, (ii) development of renal failure or impairment of normal excretory pathways, and (iii) progressive clinical deterioration despite careful medical supervision. Drug-related criteria are (i) serum concentrations in the potentially fatal range of a dialyzable substance, (ii) a correlation between serum drug concentration and toxicity, (iii) anticipated clinical benefit from faster toxin removal than what would be expected from endogenous clearance. Those xenobiotics with low volumes of distribution (less than 1 L per kg), low–molecular-weight (less than 500 Da), and low protein binding are the most amenable to enhanced clearance with dialysis. These characteristics typify the most commonly dialyzed toxicants: salicylic acid, methanol, ethylene glycol, lithium, and theophylline. Other conditions which may be amenable to extracorporeal removal or metabolic correction include poisoning by valproic acid, phenobarbital, methotrexate, and metformin-induced lactic acidosis. Table 110.11 summarizes the generally accepted common drugs and drug concentrations for which renal replacement therapy should be considered.
Risks include complications associated with central venous access, electrolyte disturbances, and hemodynamic instability. Of note, while typical dialysis patients are often hypervolemic, most poisoned dialysis patients are hypovolemic; it is incumbent upon the ED care provider to strive for euvolemia prior to hemodialysis. Very young infants in particular require extremely close attention to volume shifts. In rare neonatal cases, exchange transfusion may in fact be preferable for this reason. Nonetheless, the use of hemodialysis for other indications in pediatrics is somewhat commonplace, and in the hands of an experienced nephrologist can be safely performed. Extracorporal therapy should not be withheld even if it means transfer to another institution, as it may be essential in the critically ill poisoned child.
Supportive Care
The final step in optimizing treatment for the poisoned child is the direction of scrupulous attention to supportive care, including continued close monitoring of ABCDs, fluid and electrolyte status, urine output, and level of consciousness. The value of these efforts usually far outweighs that which may be ascribed to any specific toxicologic interventions in most cases. Severely symptomatic patients are most properly cared for in specialized facilities that have skilled pediatric critical care staff and access to toxicology consultation.
TABLE 110.12
PRODUCTS THAT ARE NONTOXIC WHEN INGESTED IN SMALL AMOUNTS
NONTOXIC INGESTION
Often, the emergency provider will be asked about a childhood ingestion of some common household products, many of which are nontoxic unless taken in huge amounts. The availability of a list of such nontoxic products often leads to immediate relief of parental anxiety and avoids the institution of unnecessary noxious interventions. Before using such a list, however, several precautions need to be kept in mind. The fact that an ingestion is nontoxic does not necessarily mean that it has no medical significance. Ingestions often occur in the context of a suboptimal environment. There may be poor supervision or unusual family stresses surrounding the incident, or the ingestion may not have been purely exploratory in nature. Several criteria have been suggested to qualify an ingestion as “nontoxic.” These include the assurance that only one identifiable product is ingested in a well-approximated amount, that the product label includes no cautionary signal word, that the child is symptom free and younger than 5 years, and that an appropriate mechanism is available for telephone follow-up. When used with these criteria, Table 110.12 provides an updated list of nontoxic ingestions. In certain cases, consultation with a regional poison control center (in the United States, the phone number 1-800-222-1222 may be used nationwide) is often helpful.
PHARMACEUTICALS
Acetaminophen
Background
Acetaminophen, N-acetyl-p-aminophenol (APAP), is the most popular pediatric analgesic–antipyretic and has now become one of the most common pharmaceutical preparations ingested by young children. It is also one of the 10 most common drugs used by adolescents and adults in intentional self-poisoning. Acetaminophen also occasionally turns up as an unreported coingestant in intentional overdoses. Fortunately, exploratory ingestion in young children has been associated with low morbidity, although occasional cases of hepatotoxicity occur, particularly in the context of inadvertent repetitive overdosing.
Pathophysiology
The major toxicity of APAP is severe hepatic damage. Acetaminophen is metabolized in three ways by the liver: (i) Glucuronidation, (ii) sulfation, and (iii) metabolism through the cytochrome P-450 pathway to form a potentially toxic intermediate, which conjugates with glutathione. In a massive overdose, glutathione becomes depleted, thus allowing the undetoxified intermediate to bind to hepatocytes, leading to cellular necrosis. This damage is reflected by rising levels of liver enzymes, by hepatic dysfunction, and in severe poisonings, by hepatic failure and death. The use of N-acetylcysteine as an antidote relates in part to this molecule’s ability to act as a glutathione precursor.
Clinical Findings
Initially, the signs and symptoms of APAP ingestion are vague and nonspecific but include nausea and vomiting, anorexia, pallor, and diaphoresis. These manifestations usually resolve within 12 to 24 hours, and the patient appears well for 1 to 4 days. During this latent period, levels of liver enzymes may rise, and jaundice with liver tenderness may ensue. Most patients have a gradual resolution of their hepatic dysfunction, although without antidotal treatment about 2% to 4% of intoxications that develop toxic plasma levels will go on to hepatic failure and death. Such patients with severe toxicity develop further clinical evidence of hepatic disease at 3 to 5 days after ingestion, and some develop renal damage. Anorexia, malaise, and abdominal pain may progress to signs of liver failure with hepatic coma.
The potential severity of an acute intoxication may be predicted by the amount ingested, if accurately known, and the plasma level of APAP. APAP in single doses of less than 200 mg per kg in young children is likely to be harmless. Severe toxicity in adolescents or adults usually occurs with overdoses of at least 7.5 to 10 g. Initial GI symptoms, although vague, are generally more pronounced when the overdose is large. However, the only reliable indication of the potential severity of the hepatic damage is the plasma APAP level, taken at least 4 hours after ingestion. After a single acute overdose at a known time of ingestion, a nomogram (Fig. 110.2) is available for using the serum APAP level in the prediction of likely toxicity. We recommend use of the lower line of the nomogram, plotted 25% below the possible toxicity line, to err on the safe side in making therapeutic decisions. Importantly, the nomogram is not validated for chronic APAP toxicity.
Management
The basic toxicologic principles of preventing absorption apply to APAP overdoses, and it is important to note that both immediate- and extended-release preparations exist. Activated charcoal therapy may be used for adsorption of gastric APAP. Many APAP-poisoned patients will benefit from the use of the oral antidote N-acetylcysteine (NAC), and some have speculated that activated charcoal might decrease the bioavailability of the NAC. However, studies have demonstrated clinically insignificant decreases in NAC absorption, even when using large doses of charcoal. For most cases of acetaminophen overdose per se, and particularly for those typically seen early after ingestion, charcoal alone is probably effective and should not significantly alter the ability to use NAC several hours later. In cases that present after 4 hours have elapsed, gastric decontamination is usually not warranted.
NAC, given orally or IV, is most effective at ameliorating hepatotoxicity when instituted within 8 hours of ingestion. It also lessens the severity of hepatic damage if used in the setting of clinical presentation beyond 8 hours. The major adverse reaction associated with IV NAC is the occurrence of anaphylactoid reaction, which typically occurs during the relatively higher dose loading infusion. Clinicians administering IV NAC should be skilled at recognizing and treating anaphylactoid reactions and should be particularly cautious when using this route in patients with a history of asthma. The inhalational form of NAC can be administered enterally and can be mixed with fruit juice or soda to disguise its foul smell, or it can be administered by NG tube. Only mild GI side effects result from its use, but persistent vomiting is an occasional obstacle to completing the course of therapy. This may be obviated by giving the dose slowly or by NG or duodenal tube infusion. Antiemetic therapy with metoclopramide or ondansetron may also be helpful.
The protocol for NAC therapy may be summarized as follows:
1. Consider GI decontamination options as already noted.
2. If patient presents less than 4 hours after a single acute ingestion, wait to draw 4-hour level and base therapeutic decision on nomogram (assumes rapid turnaround time so level will be available by 6 hours after ingestion); if necessary, initiate treatment as described next. For extended-release preparations, McNeil Consumer Products Co. (Fort Washington, Pennsylvania), a manufacturer of acetaminophen, suggests a second APAP level drawn 4 hours after the first; antidotal therapy is to be instituted if either level suggests possible toxicity.
3. If patient presents more than 6 to 8 hours after ingestion, initiate NAC therapy, obtain level, and base subsequent course of therapy on nomogram.
4. If level plots out above the lower line on the nomogram, admit the patient to hospital and continue NAC until APAP is not detectable in the blood and liver function is either normal or clearly recovering. Monitor complete blood cell count (CBC) and renal and liver function tests.
5. Treatment for patients who present more than 24 hours after ingestion is controversial, as is treatment for patients with subacute, repetitive overdosing over several days. We consider children who receive more than 150 mg per kg per day for 1 to 2 days to be at risk; again, a combination of a nondetectable APAP level and normal aminotransferases identifies a patient at low risk of hepatic injury from APAP.
FIGURE 110.2 Nomogram for estimating severity of acute acetaminophen poisoning. (Modified from Rumack BH, Matthew H. Pediatrics. 1975;55:871–876. Copyright 1975, American Academy of Pediatrics. Reprinted by permission.)
Antihistamines
Antihistamines are used to treat children with allergic diseases, as sedatives and antinauseants, and to prevent motion sickness. They are present in many cough syrups, available both over the counter (OTC) and by prescription. Antihistamines may also be found in combination with analgesics, sympathomimetic amines, and caffeine for the symptomatic relief of the common cold. They are combined with analgesics, such as salicylamide, and an anticholinergic drug, such as scopolamine, for use as a nonprescription sleep medication. Finally, they are included in some liquid cough and cold preparations that may also contain ethanol as the solvent.
Antihistamines may depress or stimulate the CNS. Used therapeutically, CNS depression is most commonly seen as drowsiness or dizziness. With increasing doses, stimulation results in insomnia, nervousness, and restlessness. In antihistamine overdose, the CNS stimulatory effects of the drug predominate. In children, CNS stimulation causes excitement, tremors, hyperactivity, hallucinations, and with higher dosages, tonic–clonic convulsions. Children are also more likely to have signs and symptoms of anticholinergic poisoning: flushed skin, fever, tachycardia, and fixed dilated pupils. The nonsedating antihistamines terfenadine and astemizole (both no longer available in the United States) have caused cardiac arrhythmia after overdose and as a result of drug–drug interactions. Cetirizine, loratadine, and fexofenadine have not produced this complication. Death from antihistamine ingestion in children is usually the result of uncontrolled seizures that progress to coma and cardiorespiratory arrest.
The treatment of antihistamine poisoning requires an accurate history of the time of ingestion and the type and quantity of drug consumed. Of particular importance is the type of drug ingested because numerous sustained-release antihistamine products are available on the market. Options for GI decontamination include the use of activated charcoal, and overdoses with the sustained-release preparations may benefit from WBI.
Patients with seizures (see Chapters 67 Seizures and 105 Neurologic Emergencies) require anticonvulsant therapy immediately. Preferably, short-term control may be gained by using diazepam, in a dose of 0.1 to 0.2 mg per kg IV. Severely agitated patients with a clear anticholinergic toxidrome may have improved sensorium after administration of physostigmine. This is usually administered in an initial dose of 0.02 mg per kg (not to exceed 0.5 mg per dose) IV slowly over 3 minutes. The dose may be repeated every 10 to 15 minutes (adult max 2 mg) to establish the effective total dose. This minimal effective dose may be repeated in several hours, if necessary. It should be noted that when administered too rapidly or in too large of a dose, physostigmine might precipitate seizures or asystole. Physostigmine would be particularly dangerous to use in the context of any coingestants that might affect intracardiac conduction, such as tricyclic antidepressants. A 12-lead EKG should be examined for conduction delays before physostigmine is given. Cardiac rhythm should be monitored closely during antidote infusion, and atropine should be available to reverse severe cholinergic effects that may also occur with physostigmine use. The potential risks encountered with physostigmine may favor use of a benzodiazepine for treatment of anticholinergic delirium.
Meticulous attention to supportive care is critical. Some patients may develop extreme hyperthermia and thus require aggressive measures to reduce core body temperature, including ice water baths or misting and fans. There is little evidence for therapeutic efficacy of dialysis or hemoperfusion because of the high plasma protein binding and large volumes of distribution for most of these agents.
Aspirin
Background
Aspirin continues to be a common cause of poisoning in children and adolescents. Salicylism is the result of acute ingestion in about 60% of cases and chronic ingestion in the remaining 40%. Clinical features of acute versus chronic salicylate intoxication often require a different management approach, depending on the manner of intoxication.
Several factors work in concert to make chronic salicylate intoxication so common. The primary factor is aspirin’s elimination pattern. As serum salicylate concentrations increase, the ability of the liver to metabolize the drug diminishes until predictable, first-order elimination kinetics are replaced by unpredictable, dose-dependent, zero-order elimination. Thereafter, increments in dose are associated with disproportionate increases in serum salicylate concentration. Also, much of aspirin elimination is through urinary excretion of unchanged drug. Therefore, in the face of dehydration and decreased glomerular filtration, drug clearance is impaired even more. Finally, because aspirin is often prescribed for illnesses that may be associated with hepatic dysfunction, reduced biotransformation initiates the spiraling increase in serum concentration. Unfortunately, because chronic salicylism is associated with nonspecific symptoms (e.g., fever, vomiting, tachypnea), diagnosis may be delayed until more striking signs of intoxication appear.
Pathophysiology
The direct effects of aspirin on metabolism are multiple. Aspirin stimulates the medullary respiratory center, which leads to tachypnea and respiratory alkalosis—its hallmark. Metabolic disturbances are widespread and include CNS hypoglycemia, as well as abnormalities in lipid and amino acid metabolism. Inhibition of Krebs cycle enzymes and uncoupling of oxidative phosphorylation in conjunction with lipid disturbances create the combined lactic and ketoacidosis responsible for metabolic acidosis (which leads to the mixed respiratory alkalosis and metabolic acidosis found on the arterial blood gas). In addition to inhibiting platelet function, aspirin intoxication is associated with disturbances in vitamin K-dependent and vitamin K-independent clotting factors, resulting in a significant coagulopathy. Mild elevations in liver enzymes are also common. Other features of aspirin intoxication include leukocytosis and electrolyte disturbances, particularly hypokalemia. Physical manifestations include fever, tachypnea, nausea, vomiting, lethargy, slurred speech, and seizures. Children with chronic salicylism are more likely to present with severe metabolic acidosis and seizures than those with acute intoxication.
The combination of respiratory alkalosis with metabolic acidosis produces an arterial blood gas that is almost pathognomonic for salicylism. Serum pH typically ranges from 7.41 to 7.55, except in severe cases in which metabolic acidosis combined with respiratory acidosis from severe CNS depression leads to pH less than 7.35, and PCO2 is generally less than 30 mm Hg. Serum bicarbonate is mildly depressed, often ranging from 15 to 20 mEq per L. However, although adults may continue to hyperventilate for extended periods when poisoned with salicylates, children with mild to moderate poisoning quickly lose this respiratory drive and are more likely to present with metabolic and respiratory acidosis.
As mentioned, glucose homeostasis is seriously altered in acute aspirin poisoning. Early in the course, hyperglycemia usually occurs because of glycogenolysis and decreased peripheral use. Later, hypoglycemia may supervene as glucose stores are depleted. High rates of oxidative metabolism in the CNS may lead to low CNS glucose concentration even in the presence of peripheral hyperglycemia.
Fluid and electrolyte disturbances are multifactorial, resulting in dehydration, hyponatremia or hypernatremia, and hypokalemia. Among contributing factors are increased insensible water losses through both skin and lungs, emesis, and increased renal water and potassium loss. The patient with severe salicylate poisoning may lose 4 to 6 L of water per square meter.
Clinical Findings
The initial clinical signs and symptoms, the estimate of dose ingested, and the measurement of salicylate levels all serve to gauge the severity of a given acute aspirin poisoning. However, in cases of chronic therapeutic salicylism, the clinical picture is the most useful guideline. Because of the nonspecific nature of symptoms with salicylism, the initial differential diagnosis is broad and may include diabetic ketoacidosis, iron intoxication, and ethylene glycol ingestion.
Signs and symptoms of salicylism depend on the method and severity of intoxication. Acute ingestion of amounts of 150 to 300 mg per kg are associated with mild symptoms, 300 to 500 mg per kg are associated with moderate toxicity, and more than 500 mg per kg are associated with death. With mild toxicity (serum concentrations 30 to 50 mg per dL), manifestations may be confined to GI upset, tinnitus, and mild tachypnea. With moderate salicylate poisoning (serum level 50 to 100 mg per dL), more visible signs of toxicity—fever, diaphoresis, and agitation—appear. After severe salicylate poisoning (serum concentrations higher than 100 mg per dL), signs and symptoms are primarily neurologic and consist of dysarthria, coma, and seizures. Pulmonary manifestations, particularly pulmonary edema, may appear in severe cases. In patients of chronic salicylism, these same conditions appear at significantly lower serum salicylate concentrations. Death from salicylism results from severe CNS toxicity with complete loss of function in cardiorespiratory centers, leading to respiratory and/or cardiac arrest. The severity of salicylate intoxication is best assessed by physical examination, electrolytes, and blood gas level analyses rather than through use of a nomogram.
Management
Assessment of the patient of salicylate intoxication begins with an accurate history that identifies the patient as having acute or chronic poisoning. Laboratory assessment is extensive and includes serum salicylate concentration, electrolytes and arterial blood gas levels, liver function tests, CBCs, prothrombin and partial thromboplastin times, urinalysis, and an EKG. In the case of intentional ingestions by adolescents, attention to a serum acetaminophen measurement is important (because many OTC analgesics contain aspirin and acetaminophen in combination).
Supportive care includes assessment of ventilatory function, cardiac monitoring, and vascular access. Because aspirin overdose is associated with delayed gastric emptying and drug coalescence to form bezoars, GI decontamination should receive careful consideration in those patients who present within 4 to 6 hours of ingestion. In patients who present more than 6 hours after ingestion or in those with chronic salicylism, activated charcoal might still be administered because it may enhance postabsorptive elimination of salicylates (through GI dialysis).
Healthcare providers are cautioned to be wary of sedating or mechanically ventilating aspirin-poisoned patients, as depressing the spontaneous ventilation rate may worsen aspirin-induced neurotoxicity. Specific therapeutic goals in salicylate intoxication include correction of fluid and electrolyte disturbances and the enhancement of salicylate excretion.
Fluid therapy should be aimed at restoring hydration and electrolyte balance, preventing distribution of salicylate to the brain, and promoting renal salicylate excretion. Aggressive restoration of intravascular volume is advisable; however, fluids should be given prudently to prevent precipitation of pulmonary edema, particularly in patients with severe intoxication. The blood pH should be kept alkaline, pH 7.45 to 7.5. For patients with symptomatic salicylate intoxication, urine alkalinization should be combined with fluid resuscitation. The administration of sodium bicarbonate, by increasing urinary pH, ionizes filtered aspirin, increasing tubular secretion and inhibiting its tubular reabsorption (ion trapping). The initial fluid is, therefore, designed to replace both sodium and bicarbonate losses as well as promote urine alkalinization. It should contain 5% dextrose with 100 to 150 mEq per L of sodium bicarbonate. Because hypokalemia impairs the ability of the kidney to create alkaline urine and is exacerbated by administration of sodium bicarbonate, potassium must be added to IV fluids. Forced diuresis should not be used as it does not enhance salicylate excretion more than the clearance accomplished by alkalinization alone. Therefore, fluids are given as needed to restore normal hydration and to produce 1 to 2 mL per kg per hour of urine. Calcium homeostasis should also be monitored during therapy with exogenous bicarbonate. Both urine alkalinization and repetitive oral charcoal (up to every 4 hours) should be continued until salicylate concentration falls below 30 mg per dL and symptoms resolve.
Salicylate elimination can also be enhanced by hemodialysis or hemoperfusion. Although hemoperfusion results in superior clearance technique, hemodialysis is usually preferred because it permits correction of fluid and electrolyte imbalances. Hemodialysis should be reserved for seriously ill patients. Hemodialysis might be considered for patients with serum salicylate levels higher than 100 mg per dL after acute ingestion or 60 mg per dL or higher after chronic salicylism. Specific indications for hemodialysis include (i) severe acidosis or other electrolyte disturbance, (ii) renal failure, (iii) persistent neurologic dysfunction, (iv) pulmonary edema, and/or (v) progressive clinical deterioration despite standard treatment.
Cardiac Drugs
β-Adrenergic Blockers and Calcium Channel Blockers
The approaches to overdoses of these two categories of cardiovascular agents are discussed together because of similarities of clinical presentation and management approach. They both are commonly prescribed to adult patients with a variety of cardiovascular disorders, including angina and past myocardial infarction, hypertension, and arrhythmias. As such, experience with pediatric overdoses has been increasing in more recent years.
BBs vary considerably in terms of receptor specificity and pharmacokinetics, but most overdose experience is with propranolol. Similarly, the calcium channel blockers (CCBs) most commonly used in the United States (verapamil, diltiazem, nifedipine, amlodipine, etc.) are chemically dissimilar and have varied degrees of effect on vasodilation, myocardial contractility, and sinoatrial (SA)-atrioventricular (AV) node function.
Both BBs and CCBs may present with fulminant cardiovascular and neurologic findings after a large overdose. Typical presentations of both agents include marked bradycardia and hypotension; particularly with the CCBs, common additional findings are those of abnormal AV node conduction, with AV block or accelerated junctional rhythm. The CNS may also be affected, with coma and/or convulsions that occur in either category of overdoses. Metabolic disturbances include hypoglycemia with BBs and hyperglycemia and metabolic acidosis with CCBs. Bronchospasm may further complicate BB toxicity in patients with underlying reactive airway disease.
Management begins with aggressive gastric decontamination for both types of agents. Activated charcoal/cathartic should be administered to patients presenting soon after ingestion. Sustained-release preparations may cause prolonged effects, and WBI may be considered in this context. Bradycardia and hypotension may improve with standard treatment such as atropine, fluid boluses, and direct-acting vasopressors such as norepinephrine; however, many cases prove resistant to these measures.
Additional therapy includes calcium infusion for the CCBs, with the recommended adult initial dose being 10 mL of 10% calcium chloride or 30 mL of 10% calcium gluconate, which may be repeated two or three times as necessary (e.g., an initial pediatric dose of approximately 0.2 mL per kg calcium chloride or 0.6 mL per kg of calcium gluconate). Serum calcium, as well as the ionized calcium, should be monitored. Glucagon increases intracellular cyclic adenosine monophosphate (cAMP) by a mechanism independent of β receptors and has been used with success to improve heart rate and blood pressure in overdoses of BB agents. The usual adult dosing regimen is 3 to 5 mg by IV bolus, which may be repeated to a total dose of 10 mg, followed by infusion at 2 to 5 mg per hour. Such dosing translates to 50 to 150 μg per kg boluses and similar amounts per hour for pediatric patients.
For hemodynamically significant overdose of a CCB, hyperinsulinemia–euglycemia therapy is recommended; this therapy should be guided by a clinician or poison control center consultant familiar with its use. Bolus dosing of 1 unit per kg IV of regular insulin is then followed by an infusion at 0.5 units to 1 unit per kg with titration performed based on hemodynamics. Blood sugars are carefully monitored. Lipid emulsion infusion has shown promise in animal studies of verapamil toxicity as well as case reports; it is typically given if conventional therapies, including insulin are refractory or if the patient has a cardiac arrest. Severe cases may also benefit from pacemaker insertion and consideration of aortic balloon pump and/or cardiopulmonary bypass. It is unlikely that hemodialysis or hemoperfusion would benefit most of these cases.
Clonidine
Clonidine is an antihypertensive that appears to have growing popularity, part of which comes from its efficacy in illnesses other than hypertension, including opioid withdrawal and attention deficit disorder. Also, the advent of clonidine in transdermal patches has become a convenient and somewhat unique vehicle for drug administration.
Clonidine exerts its antihypertensive effect through stimulation of CNS α2-adrenergic receptors. These receptors are located on presynaptic neurons in cardiorespiratory centers of the midbrain. Their stimulation results in decreased secretion of catecholamines into the synaptic cleft, resulting in decreased pulse and blood pressure. In addition, clonidine appears to interact with or modulate CNS opiate receptors; this interaction has been used to explain clonidine’s efficacy in opiate withdrawal and the picture of coma and miosis that accompanies clonidine intoxication. An imidazoline compound, clonidine is related to other medications, including tetrahydrozoline and oxymetazoline—common vasoconstrictors found in nasal decongestants and ophthalmic agents. Brimonidine drops may be used in an ophthalmology setting and guanfacine is used also for attention deficit disorder.
Clonidine is an extremely potent drug with typical doses of 100 to 200 μg in adults. Therefore, ingestions of small amounts can potentially lead to significant toxicity in children. Initial toxic manifestations include altered mental status that may range from lethargy to coma. Patients may also develop hypothermia. In severe intoxications, coma, miosis, and respiratory depression may appear. The cardiovascular changes that accompany clonidine intoxications may range from an initial transient period of hypertension (often resolved prior to arrival in the ED) to profound hypotension and bradycardia. Clonidine-induced hypertension is believed to result from α-adrenergic effects at peripheral vascular receptors prior to the central, antihypertensive effect. The clinical picture of clonidine intoxication typically lasts 8 to 24 hours.
Management
The treatment of clonidine intoxication requires immediate assessment of the ABCs. Because patients with severe intoxication often have coma and respiratory depression, emergency endotracheal intubation may be necessary. Also, because of blood pressure instability, vascular access should be achieved immediately for better hemodynamic control. Hypotension should be treated with fluids and vasopressors as needed. Hypertension is generally uncommon, is very transient, and would rarely require specific treatment.
Activated charcoal binds clonidine. In addition to supportive care measures, other pharmacologic interventions may be effective. Naloxone has been suggested as a specific antidotal agent after clonidine intoxication, based on case reports of improved mental status and cardiorespiratory function after its administration. However, in reported case series, there have not been consistent improvements after naloxone administration.
Because naloxone is a benign agent in an opioid naïve child and may potentially improve mental status to the extent that intubation becomes unnecessary, a trial dose of 0.1 to 0.2 mg per kg should be administered. Large amounts of naloxone (up to 8 mg) must be provided before it can be concluded that the intoxication is not responsive to this therapy. If effective, repeat doses or a continuous infusion of naloxone may be useful. Other pharmacologic agents that have been used in the past include yohimbine, tolazoline, and phentolamine; but specific efficacy from these agents has not been demonstrated, and they are not considered important in the treatment of clonidine intoxication.
Digoxin
Digoxin is still widely used in young infants with congenital heart disease and elderly patients with congestive heart failure. This medication’s continued popularity, its narrow therapeutic index, and the appealing color of digoxin elixir make it a source of many childhood poisoning episodes annually. Also, related agents, particularly the foxglove and oleander plants, are occasionally ingested by children, leading to a clinical picture identical to that of digoxin.
Digoxin’s primary pharmacologic action is to inhibit activity of sodium–potassium adenosine triphosphatase (ATPase), which is responsible for maintaining the electrical potential of excitable tissues through transmembrane concentration of electrolytes. Therefore, the effects of digoxin are largely related to disturbances in this action.
In all patients of digoxin poisoning, two distinct pictures of toxicity exist: acute and chronic. These pictures have several differences: the patient of acute digoxin ingestion is typically a toddler who ingests a relative’s medication. The toddler is generally healthy with no underlying cardiac disease. The child with chronic digoxin poisoning, however, by definition has pre-existing heart disease and is likely to be taking other medications known to modulate the effects of digoxin poisoning (e.g., diuretics). Therefore, it is the latter patient who is more likely to have severe toxic manifestations after digoxin intoxication.
Digoxin pharmacokinetics are complex. After ingestion, absorption is complete within 2 to 4 hours. However, after peak serum levels are achieved, the drug is rapidly redistributed, resulting in dramatic falls in serum concentration. This principle has particular importance with the patient of acute digoxin intoxication who may have an initial serum digoxin concentration (SDC) in the highly toxic range that falls to the therapeutic range within a matter of hours. After redistribution, digoxin elimination occurs primarily through renal excretion of unchanged drug. Therefore, any condition associated with decreased renal function may be associated with the insidious development of intoxication.
The therapeutic SDC is less than 2 ng per mL. A concentration in the slightly higher range often does not correlate with clinical manifestations and may be of limited value. However, when SDC exceeds 4 ng per mL, some evidence of intoxication usually appears. This toxicity is influenced by many host factors, including patient age; underlying illness; and disturbances in serum potassium, magnesium, and calcium.
With significant intoxication, the symptoms of digoxin poisoning include nausea, vomiting, and visual disturbances. With more severe intoxication, additional symptoms, including lethargy, disorientation, electrolyte disturbances, and cardiac disturbances, appear. The hallmark of severe acute digoxin toxicity is hyperkalemia, the result of profound inhibition of sodium–potassium ATPase activity. The typical pattern of cardiac toxicity with digoxin overdose initially is prolonged AV dissociation that appears as heart block that ranges from first to third degree. These conduction disturbances can lead to the development of ventricular or supraventricular escape rhythms. In patients with chronic digoxin intoxication, these symptoms may be more striking than in those with acute, single digoxin overdoses. In fact, children with acute digoxin intoxication rarely develop life-threatening illness if their peak SDC remains below 10 ng per mL.
Management
The management of the patient with digoxin intoxication begins with evaluation of the vital signs, particularly hemodynamic status. Patients should have an EKG performed, followed by continuous cardiac monitoring. If significant cardiac arrhythmias are already present, they are treated initially according to advanced cardiac life support protocols.
GI decontamination should include administration of activated charcoal. Clinical assessment typically includes an EKG, electrolytes (including magnesium and calcium) level, urinalysis, and SDC. Electrolyte disturbances should be treated aggressively because they will aggravate any digoxin-induced arrhythmias.
Digoxin-specific antibody fragments have become specific antidotal therapy for reversing the toxic manifestations. These fragments are the result of sheep-derived immunoglobulin that is cleaved to extract only the Fab fragment. This low–molecular-weight antibody fragment is capable of avidly binding free digoxin so a gradient results that favors digoxin removal from receptor sites into interstitial water. The effect of this gradient is that sodium–potassium ATPase function is immediately restored. The digoxin–antibody complex is then rapidly excreted in the urine. Of note, after digoxin-antibody fragments are administered, SDC increases astronomically, reflecting bound, inactive digoxin that has diffused into the vascular compartment.
These antibody fragments are indicated in the following circumstances after digoxin poisoning: (i) Progressive signs and symptoms of intoxication, (ii) life-threatening cardiac arrhythmias, or (iii) severe hyperkalemia (defined as a serum potassium level of 5.5 mEq per L or higher). The dose of antibody fragments is calculated on the basis of ingested digoxin dose (in the case of acute intoxication) or on the basis of SDC (in the case of chronic intoxication). Each 40-mg vial of digoxin-Fab will bind 0.6 mg of digoxin. The total dose of Fab needed (in vials) may be estimated by dividing a known ingested dose by 0.6, or calculated for the steady-state context as body load of digoxin:
Complications from the administration of antibody fragments are low and consist of an allergic reaction (in approximately 0.6% of patients), precipitation of congestive heart failure (secondary to the abrupt loss of digoxin’s inotropic action), and rebound hypokalemia. These complications should be anticipated and treated accordingly. Infusions should be given over 30 minutes, and some prefer to use a 0.22 μm in-line filter. If the patient is in cardiac arrest, the antibody fragments may be infused over 5 minutes.
Iron
Background
In the 1990s, iron poisoning was one of the most common, potentially fatal intoxications in children. Most serious childhood poisonings result from ingestion of prenatal vitamins or ferrous sulfate tablets (which unfortunately often look much like candy) that were intended for adults. A common scenario is that the patient is a toddler whose mother has just had a new baby; the increased demands on the mother’s attention and almost universal prescription of iron to postpartum women combine to set the stage for this ingestion. In addition, numerous exposures result from ingestion of iron-fortified children’s vitamins, but these tend to be far less toxic.
Sufficient data to define a safe lower limit for toxic iron ingestions are not available. As little as 20 mg per kg of elemental iron has caused GI toxicity, whereas ingestions of more than 40 mg per kg often produce moderate toxic effects with profound toxicity seen after doses of 60 mg per kg. Of course, it is often impossible to know the exact number of tablets ingested. As few as ten 300-mg FeSO4 tablets have been fatal to a young child. Furthermore, the elemental iron content of whole bottles of chewable vitamins is usually about 1,200 mg. Industry standards typically lead to the use of child resistant caps for vitamin bottles that contain more than 250 mg of elemental iron. Unit dose (blister) packaging has been advised for pills with high iron content. These measures have led to a dramatic reduction in deaths due to exploratory iron poisoning.
Pathophysiology
Iron toxicity results from direct caustic effect on the GI mucosa and the presence of free iron in the circulation. Pathologic changes include hemorrhagic necrosis of stomach and intestinal mucosa and lesions in the liver that range from cloudy swelling to areas of complete necrosis. Occasionally, pulmonary congestion and hemorrhage are noted. Excess free iron is believed to act as a mitochondrial poison, particularly in the liver, with resulting changes in cellular energy metabolism and the production of metabolic acidosis.
Clinical Manifestations
The clinical effects of iron poisoning are classically divided into four phases. Phase I represents the effects of direct mucosal injury and usually lasts 6 hours. Vomiting, diarrhea, and GI blood loss are the prominent early signs; when severe, the patient may lapse into early coma and shock caused by volume loss and metabolic acidosis.
Phase II, which lasts from 6 to 24 hours after ingestion, is marked by diminution of the GI symptoms. With appropriate therapy to replace fluid and/or blood losses, the child may seem relatively well and often goes on to full recovery without any subsequent symptoms. However, this remission may be transient and may be followed by phase III, characterized by metabolic acidosis, coma, seizures, and intractable shock. This phase is believed to represent hepatocellular injury with consequent disturbed energy metabolism; elevated levels of lactic and citric acids are noted in experimental iron poisoning before cardiac or respiratory failure occurs. Jaundice and elevated transaminases are noted in this phase. A phase IV has been described in survivors of severe iron poisoning, marked by pyloric stenosis that results from scarring and consequent obstruction.
Laboratory abnormalities often associated with severe iron intoxication include metabolic acidosis, leukocytosis, hyperglycemia, hyperbilirubinemia and increased liver enzymes, and a prolonged prothrombin time. If fluid loss is significant, there will be hemoconcentration and elevated BUN. Abdominal films may show radiopaque material in the stomach, but the absence of this finding does not indicate a trivial ingestion.
Management
All children alleged to have ingested iron are potentially at significant risk for life-threatening illness. However, severe iron poisoning is uncommon compared with the number of children who develop only mild symptoms or remain entirely asymptomatic. Thus, the emergency physician needs an approach that encompasses the response to the severely poisoned child and to most who will remain well.
As noted earlier, the amount of iron ingested is often hard to quantify, and minimal “safe” amounts are not well established. Serum iron levels have been shown to correlate with the likelihood of developing symptoms (usually a reflection of the serum iron that exceeds the iron-binding capacity and results in free-circulating iron). Usually, when drawn 3 to 5 hours after ingestion, iron levels lower than 350 μg per dL predict an asymptomatic course. Patients with levels in the 350 to 500 μg per dL range often show mild phase I symptoms but rarely develop serious complications. Levels higher than 500 μg per dL suggest significant risk for phase III manifestations. However, the serum iron determination is not always available on a stat basis.
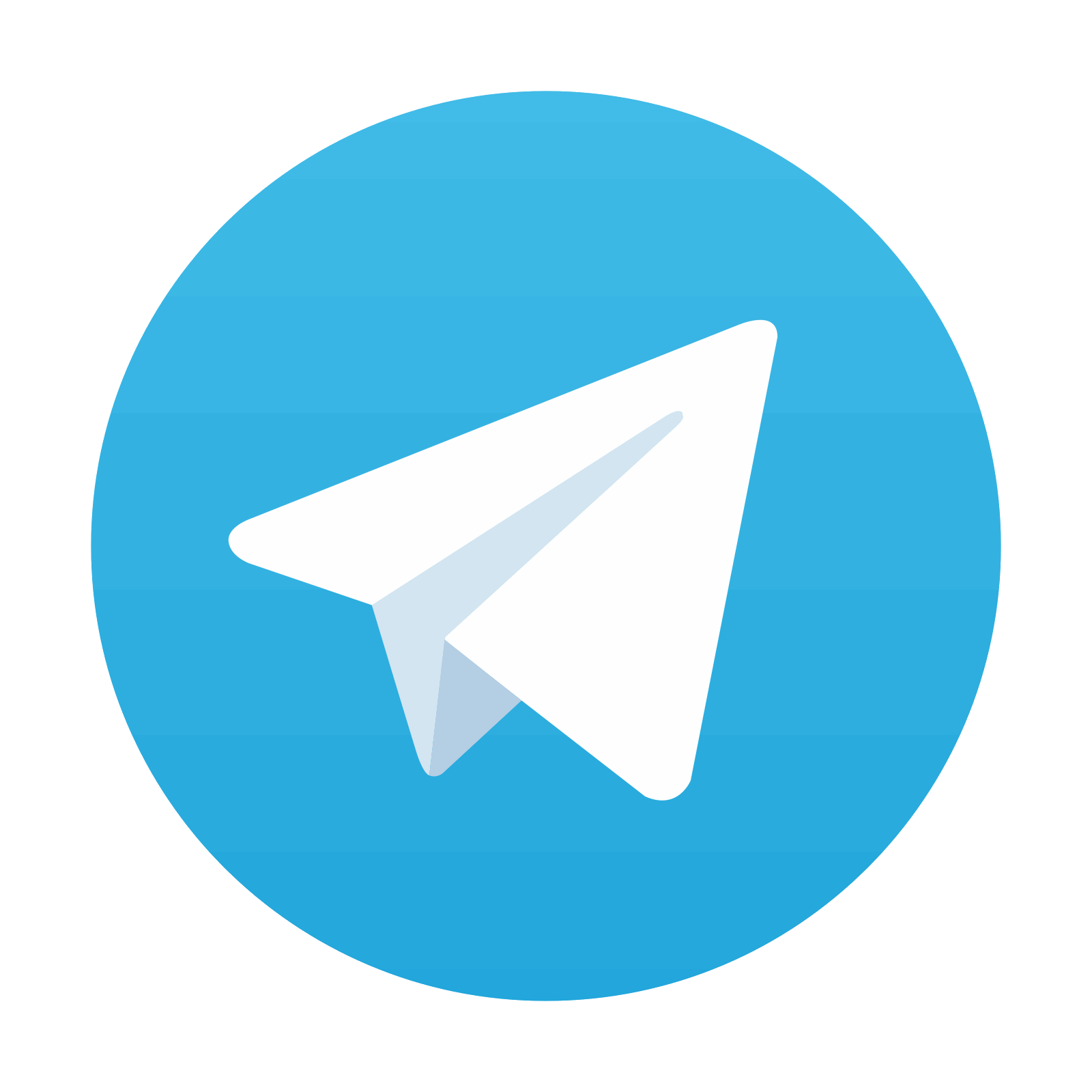
Stay updated, free articles. Join our Telegram channel

Full access? Get Clinical Tree
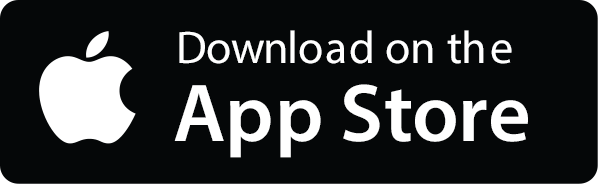
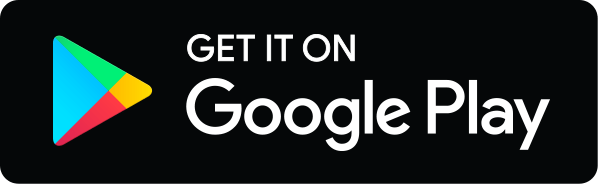