Chapter 12 Management of the patient who suffers major blood loss presents many challenges to the intensivists, trauma surgeon and other members of the trauma care team. In the past, crystalloid administration has been the initial resuscitation fluid followed by blood transfusion. However, many patients require immediate infusion of blood, plasma, coagulation factors including platelets and cryoprecipitate, fibrinogen, and tranexamic acid to improve survival as part of a massive transfusion protocol. Although, there is no universally accepted protocol, general principles of massive transfusion should be adopted for rational use of the massive transfusion protocol. This involves collaboration of the intensivists with the surgeon, hematopathologist, anesthetist and other members of the trauma care team. Simultaneous with massive blood loss, patients develop coagulopathy because of the trauma itself as well as the result of hypothermia, acidosis, dilution, and consumption of coagulation factors. Resuscitation should therefore involve not only fluid and blood with blood products and coagulation factors but also monitor preventing and controlling the coagulopathy accompanying trauma with hypoperfusion from major blood loss. In this chapter, we discuss the coagulopathy of trauma and surgery, its etiology and diagnosis with principles of monitoring and management. We also discuss the definition of massive blood transfusion, its identification and criteria for implementing and applying a massive transfusion protocol. The coagulation system is a balance of two major processes — one is aimed at clotting to prevent or stop bleeding (procoagulant and antifibrinolysis) and the other to promote ‘liquidity’ and flowing of blood through anticoagulation and fibrinolysis. It has been reported that as many as 25% of trauma patients present to the emergency room in a coagulopathic state1 with overall 21% of deaths following trauma being due to hemorrhage of which 50% die within 12 hours and 70% in 48 hours. This coagulopathic state is thought to result from a series of factors. Early after tissue injury there is release of tissue factor (thromboplastin) which forms a complex with Factor VIIa with direct activation of Factor Xc and activation of Factor IXa forming a complex with Factor VIIa and phospholipids. Also, Factor Xa complexes with Factor Va and phospholipids to convert prothrombin to thrombin which converts fibrinogen to fibrin and clot formation. With continued hemorrhage there is depletion of fibrinogen and platelets in the process of clot formation leading to consumption of platelets and coagulation factors (consumption coagulopathy) which aggravates the bleeding process. Trauma coagulopathy arises in an environment of a complex mixture of inflammation, and cellular dysfunction with rapid evolution over mins to hours from an anticoagulant to a procoagulant state. Although all mechanisms are not clearly delineated, several key processes have been identified including dysfunction of natural anticoagulation mechanisms, platelet dysfunction, fibrinogen consumption, and hyper fibrinolysis2 compounded by hypoperfuson, dilution from resuscitation fluids, hypothermia, and acidosis secondary to the hypoperfusion. The thrombin–thrombomodulin–protein C anticoagulation system has also been implicated as a mechanism of anticoagulation. Decrease in protein C levels has been attributed to its activation (aPC) by thrombin bound to thrombomodulin.3 The anticoagulation properties of aPC derives from its degradation of activated Factors V and VIII leading to decreased concentration of these factors as demonstrated by Rizoli et al.4 who reported consistent reduction in Factor V among 110 trauma patients. Endogenous autoheparinization has also been implicated possibly secondary to shedding of the endothelial glycocalyx as suggested by the ability to reverse the anticoagulation by adding heparinase to the blood of trauma patients with coagulopathy.5 The observation that platelet counts are typically normal in early trauma coagulopathy suggests that platelet dysfunction rather than actual dilution or depletion of platelets is a primary contributing factor to the coagulopathy of trauma. Kutcher et al.6 demonstrated early platelet dysfunction through impedance aggregometry in trauma patients on arrival in the emergency room. These platelet defects have been shown to contribute to increased trauma mortality.7 Platelet contribution to clot firmness as measured by rotational thromboelastometry (ROTEM) was also diminished in non-survivors of major trauma.8 Fibrinogen after activation by thrombin polymerizes to a fibrin mesh which forms a clot with platelets to stop hemorrhage at sites of vascular injury. This is the primary process for hemostasis in areas of injury. Consumption of fibrinogen and fibrinolysis through the action of plasmin are major components of the coagulopathy of trauma. Admission fibrinogen levels correlate with measurement of clot firmness by ROTEM and is an independent predictor of early and late deaths following trauma as reported by Rourke et al.9 Fibrin clot formation is counterbalanced by fibrinolysis which is due to the binding of tissue plasminogen activator (tPA) to plasminogen which is then converted to plasmin which destroys the scaffold of the clot by lysing the fibrin fibers. Increased fibrinolysis or hyperfibrinolysis is a known accompaniment of hemorrhagic shock as demonstrated by increased levels of tPA and the clot breakdown product, D-dimer in patients with hemorrhagic traumatic shock.3,10 Using visco-elastic methods, hyperfibrinolysis has been shown to correlate with exceedingly high mortality in trauma patients.11 In addition to the above mechanisms implicated in trauma coagulopathy there is evidence for a significant role of pro-inflammatory cytokines, neutrophil activation, histones, extracellular DNA etc. uncovering a host of diverse reaction to shock and hemorrhage following trauma with potential disastrous consequences. The main goal of treatment is, therefore, early control of hemorrhage to stop the continued requirement for clot formation and consumptive coagulopathy with depletion of clotting factors and hyperfibrinolysis. With established trauma induced coagulopathy mortality is increased 3–4 fold and 24 hours mortality is increased 8-fold with resulting increase in transfusion requirement, increased ICU stay, mechanical ventilation days and incidence of multiple organ failure. This coagulopathy is not only due to hemodilution and consumption of clotting factors but also direct tissue injury leading to release of potent mediators of the inflammatory response and hypoperfusion which is compounded by hypothermia and acidosis all resulting in hyperfibrinolysis and bleeding. Identifying and treating these triggers of hyperfibrinolysis by maintaining perfusion thus decreasing the base deficit, preventing hypothermia which affects platelet function and other clotting factors, by active aggressive warming techniques are important treatment strategies. However, the coagulation defect itself also needs to be identified and targeted for successful outcome. Whereas, minimal trauma with hemorrhage that is successfully treated, is traditionally managed by initial crystalloid followed by packed cells as needed, the massively bleeding trauma patient in prolonged shock with a predilection to hypothermia and acidosis has to be managed not only by treating the surgical lesion but by early identification and correction of the associated trauma induced coagulopathy by replacement of depleted coagulation factors and curtailing the vicious cycle resulting from continued hemorrhage. When the clinical history and presentation suggest major hemorrhage and/or when there is associated acidosis or hypothermia with a continued state of hypoperfusion, the treatment protocol should involve active warming of the patient and prompt initial transfusion of not only packed red blood cells (RBC) but also coagulation factors. Many different “Massive Transfusion Protocols (MTP)” have been described in the literature including different ratios of packed cells, fresh frozen plasma, platelets, cryoprecipitate, fibrinogen, tranexamic acid etc.12 but there is no universal agreement on any one of these protocols. The usual tests of coagulation such as INR, PT, PTT, platelet counts require time to complete and interpret in order to guide therapy. These tests are also performed at the 37°C temperature level in the laboratory thus not reflecting their true measurement in the bleeding patient.13 These tests do not provide crucial information on clot strength and stability and because whole cells are removed by centrifugation before completing the tests, their contribution to clotting from platelets, erythrocytes and tissue factor bearing cells are not considered and the key role of fibrinogen and hyperfibrinolysis are neglected. Availability of a rapid measurement of the strength of the clot, platelet function and fibrinogen level and the degree of fibrinolysis at the bedside would allow immediate correction of targeted abnormalities to correct the bleeding without the necessity to adhere to any particular ratio. It is evident that early identification of predictors of Massive transfusion protocols would result in correction of the above mechanisms of trauma coagulopathy and hopefully stop the vicious cycle leading to increased mortality following hemorrhage and hypoperfusion from trauma. Clinically, a patient’s history of injury associated with significant hemorrhage and shock should and often does trigger the massive transfusion protocol. However, in order to standardize triggers in specific circumstances many other triggers have been described: Replacement of the patient’ entire blood volume in a 24 hours period (10–12 units of PRBC), replacement of 50% total blood volume in three hours (5–6 units PRBC), need for at least four units RBC’s within four hours with continued major bleeding, blood loss exceeding 150 ml/min. The Shock index (SI) defined as the ratio of heart rate to systolic blood pressure has been used by Mutscher et al.14 demonstrating that with increasing SI there was increased need for transfusion. The Individual Transfusion Trigger (Cincinnati ITT or CITT) study,15 the Trauma Associated severe Hemorhage (TASH) score and the Assessment of Blood Consumption (ABC) score16 have also been utilized as MT triggers. The CITT triggers are: Systolic blood pressure, 90 mmHg, Hb <11 g/dl, temp <35.5 C, INR >1.5 and base deficit of 6 or greater. The ABC score includes SBP <90 mmHg, Heart rate 120 or greater per min, positive Focused Assessment sonogram for Trauma (FAST) and penetrating mechanism. Transfusion triggers described by Callcut et al.17 are: INR >1.5, systolic blood pressure, 90 mmHg, Hb, 11 g/dl, base deficit>6, FAST positivity, heart rate 120/min, and penetrating trauma mechanism, combining both CITT and ABC criteria to arrive at a total of eight parameters. From these parameters, a Massive transfusion Score (MTS) was derived by assigning one point to each parameter and obtaining the sum of these points. Using the Prospective Observational Multicentre Major Trauma Transfusion (PROMMITT) model,18 Callcut et al.17 prospectively recruited 1,245 patients with 297 receiving MT. All triggers except penetrating mechanism and heart rate were valid individual predictors of MT with INR being the most predictive. Patients with an MTS of less than two were unlikely to receive MT (neg predictive value −89%). If any two triggers were present, sensitivity for predicting MT was 85%. The authors concluded that parameters which are obtainable early in the initial emergency department evaluation are valid predictors of the likelihood of Massive transfusion. Hemorrhage in Trauma results in loss of whole blood which includes not only RBCs but also plasma, platelets, and other coagulation factors. It seems reasonable that whole blood loss should therefore be treated by replacement with whole blood rather than component therapy consisting of red cells, platelets and coagulation factors. Several studies, particularly in the military setting have demonstrated effectiveness of whole blood transfusion. Cottton et al.19 demonstrated that patients receiving modified whole blood (after use of leukoreduction filter and without native platelet function), required fewer products during a 24 hours period compared to those receiving component therapy. However, there were no differences in 24 hours or 30 day mortality. Before wide spread adoption of whole blood transfusion as replacement for blood loss, several obstacles need to be overcome-possible link between white cell contamination and transfusion complications, adequacy of removal of possible pathogens and the availability and procurement of whole blood itself, to name a few. Until these issues are resolved we are left with using packed RBCs and component therapy. Blood transfusion itself, though very important, has to be combined with other aspects of resuscitation of the trauma patient in hemorrhagic shock viz: Restoration of circulating blood volume, maintenance of perfusion, and prevention of acidosis and hypothermia. Regarding the blood transfusion, several protocols have been advocated. They practically all have in common the infusion of packed red cells, platelets and fresh frozen plasma but in different ratios, the most common being 1:1:1, although there is no uniformity. Use of component therapy, generally results in better outcome12 but the optimum ratio still remains elusive. The non-uniformity in massive transfusion protocol becomes more complex when it is observed that elements such as cryoprecipitate, fibrinogen, calcium, and tranexamic acid are incorporated into some protocols and not in others. While the roles of the other elements such as fibrinogen and cryoprecipitate in some massive transfusion protocols may be evident, special aspects of tranexamic acid are worth considering. Tranexamic acid (TA) is a synthetic derivative of the amino acid lysine and acts as an antifibrinolytic, binding to plasminogen to block the interaction of plasminogen with fibrin and preventing the dissolution of the fibrin clot with an expected effect on decreasing hemorrhage from points of injury. It has decreased 28 day all-cause mortality in bleeding trauma patients, (allcause mortality decreasing from 16.0%–14.5%, reduced death from bleeding from 5.7%–4.9%, early TA–<1 hour from injury — was associated with the greatest decrease — 32% — in death from bleeding, between 1 and 3 hours there was still a decrease in death from bleeding but after three hours of injury there was an increased risk of death from bleeding). Interestingly, there was no increased vascular occlusive events with TA in spite of concern for increased clotting.20 As pointed out above, considering the coagulopathy associated with major trauma, coagulation tests would be expected to be helpful in guiding therapy in the massively transfused trauma patient. The time taken to complete and interpret these tests, the fact that they are conducted at 37°C rather than the actual patient temperature among other factors make these tests impractical for guiding therapy in acute situations. There are thus two choices in guiding therapy — (a) using ratios of products that hopefully would be effective in curtailing the hemorrhage as in the various massive transfusion protocols discussed so far or (b) using immediate available results of coagulation tests at the patient’s bed side (in the Emergency room, Operating room or ICU) focusing on identified coagulation defects in the hemorrhaging trauma patient to guide rational use of blood and coagulation products — referred to as point of care (POC) coagulation testing. Appropriately applied, this would be expected, not only to target and correct specific deficiencies in a timely fashion but also avoid unnecessary wastage of blood and coagulation products. It has been demonstrated that in massive bleeders, delay in specific coagulation therapy is associated with poor outcome.21 Also, the use of high plasma: Red cell ratios in some patient groups do not improve survival but may also increase complication rates.22 Holcomb et al.23 in the PROPPR Randomized clinical trial, compared transfusion of plasma, platelets, and RBCs in a ratio of 1:1:1 to 1:1:2 in massively bleeding patients and found no significant difference in mortality at 24 hours or at 30 days. However, more patients in the 1:1:1 group achieved hemostasis and fewer experienced death due to exsanguination by 24 hours. Viscoelastic POC coagulation monitoring tools such as ROTEM (ROTEM-Tem International, Munich, Germany) and thromboelastography (TEG-Hemonetics, Braintree, Massachusetts) have made it possible to rapidly assess the initiation of clot formation, clot strength, and clot stability at the patient’s bedside allowing individualized coagulation therapy. Ideally, these tests and results should be immediately available but in situations where they are not immediately available or “setup time” is required, the traditional “blind” protocol ratio guided transfusion is appropriate, the ultimate goal being to support the circulation and hemostasis as expeditiously as possible in the massively bleeding patient. Both ROTEM and TEG allow identification of different causes of bleeding by assessing both the intrinsic and extrinsic coagulation pathways. The fibrin dependent component of the clot can be accurately and instantly assessed providing data on the stability and strength of the clot as well as the degree of hyperfibrinolysis, prompting specific goal directed therapy. In retrospective analysis of data at a level 1 trauma center24 TEG provided real time measure of thrombostatic function with the ability to reduce the need for FFP administration. ROTEM allowed detection of more coagulation abnormalities than PT and aPTT in a prospective analysis of deployed military personnel25 allowing both monitoring and guiding individualized therapy during massive transfusion. The maximum clot strength measured by ROTEM is a result of the interaction of activated platelets, fibrin and aFXIII. As such, a maximum strength of the clot can be achieved in severe bleeding by administration of sufficient fibrinogen. Output from the ROTEM generated measurements allows focus on fibrin deficit for which specific dosage of fibrinogen concentrate would be recommended to correct the deficit. Similarly, thrombin generation deficit may be uncovered prompting specific treatment as well as platelet deficit prompting administration of platelet concentrate. Severe clot deficiency may be treated with TA, fibrinogen concentrate, high doses of FFP, or increasing platelet counts as determined by the ROTEM guided algorithm. The apparatus also allows detection of potential heparin exposure prompting reversal with protamine and clot instability not related to hyperfibrinolysis could be treated by Factor XIII administration just to highlight a few of the specific abnormalities detectable with suggested intervention through examining the ROTEM output. Regardless of the chosen formula for administering and monitoring the process of massive blood transfusion, the protocol requires a detailed well planned cohesive team approach involving physicians, laboratory staff, nurses, communication, and transport services. The protocol described here is extracted, with permission and authored primarily by Dr. Katerina Pavensky our hematopathologist and coordinator of the Massive transfusion service at St. Michaels Hospital in Toronto with the assistance of Dr. Sandro Rizoli, the Transfusion Committee and Trauma Care Committee of the hospital. Protocols will differ from institution to institution but the details presented here is used to highlight features of one protocol to demonstrate the necessity for having a well-planned institution wide protocol in which all sections involved will be aware of their respective roles and responsibilities having reviewed the protocol in detail and agreed to participate fully in adherence to the protocol. This ensures that when the protocol has been approved by all it will be applied uniformly. Otherwise chaos to the detriment of patient care could easily result at a time when urgent cohesive efforts are required and there is no time for ad hoc protocol implementation. In this protocol, massive transfusion is defined as requirement for >6 units of RBCs in a four hours period to replace blood loss from trauma, postpartum hemorrhage, upper GI hemorrhage, postoperative bleeding, ruptured aortic aneurysm etc. The specific goals of MTP include: (a) Provision of optimal resuscitation. (b) Prevent or manage coagulopathy. (c) Timely and appropriate provision of blood components and other supportive measures in a standardized manner. (d) Guide expedient definitive management of bleeding. (e) Avoid unnecessary transfusions and their complications. (f) Avoid wastage of blood components. (a) At the bedside — physicians, nurses, respiratory therapists, MTP leads, and MTP Assistants. (b) Transfusion medicine lab — MTP medical laboratory technologist (MLT). (c) Core lab — medical laboratory technologist. (d) Portering service — MTP PORTER. (e) Central locating — phone, communication, pagers, cellular contact. (f) Others: Critical Care. OR, Endoscopy, interventional Radiology. 1. MTP LEAD is a staff physician or clinical fellow — Gives order to initiate and terminate MTP. — Leads resuscitation efforts (orders blood components, medications, IV fluids and therapeutic interventions. — When necessary, obtains central venous access and secures airway. — Reasseses need for ongoing MTP q1h. — Monitors patient clinically and with laboratory testing. — Mobilizes additional resources as necessary e.g., OR. — Signs the emergency blood release form if applicable. — Following MTP, charts summary of resuscitation. 2. MTP ASSIST is A Registered nurse — Aids in preparing patient monitors and necessary equipment including blood warmer. — Monitors patient. — Obtains peripheral venous access. — Performs phlebotomy for laboratory testing as required. — Administers medication, resuscitation fluids and blood components. — During MTP, documents interventions and clinical data. — Acts as a liaison between bedside team and other areas (laboratory, portering, imaging, OR etc). For example informs Transfusion Medicine of patient’s changing blood components needs, a significant change in patient’s condition or change in patient’s location. — Available to assist MTP Lead as necessary. 3. RESPIRATORY Therapist — Assists with securing an airway and providing oxygen therapy/ventilation as necessary. — Assists with set up of a blood warmer. — Administers resuscitating fluids and blood components. — Available to assist MTP lead as needed. 4. Perfusionist — Administers resuscitating fluids and blood components. — Available to assist MTP lead as needed. — Sets up and performs cell salvage if needed. — One staff designated as MTP Technologist. — Coordinates blood component preparation and issue. — Communicates with the clinical team. — Prioritizes preparation of blood components for MTP over other work. — May provide recommendations on transfusion management (e.g., Dosing of a component). — Fills out MTP Monitoring Tool (record of components issued). 6. Core Lab — Prioritizes MTP blood specimens over other work (SUPER STAT). — Conveys critical results to the MTP team. 7. Portering Service — One staff is designated as an MTP PORTER for the duration of the MTP. — Transports blood samples and blood components during MTP. — Returns MTP cooler promptly to TM once blood components are transfused or MTP terminated. Note: If MTP is initiated in the OR, the blood portering process is outlined in the “Obtaining and Administering Blood/Blood Products within Perioperative Services” policy will be followed. If the patient is transferred to the OR with MTP in progress, portering Services will continue with blood sample and blood component in transport until the patient is admitted to the Perioperative Services. At this point, the hospital porter will report to the OR Patient Care Coordinator/Charge Nurse for transfer of accountability. The charge nurse will then assign a USW or PSA to the case. In rare circumstances (e.g., Insufficient staffing, multiple complex patients’ demands), the charge nurse may ask the Porter to continue blood transport for an MTP patient the OR. 8. Anesthesiologist staff on call — Is available to assist with obtaining venous access, invasive monitoring, securing airway, and transfusion management. — Be prepared to take over the role of MTP Lead if required. — Once MTP is activated, Central Locating will notify the appropriate parties (see MTP activation). 10. Others Surgery, interventional radiology, endoscopy — MTP lead may consult an appropriate service as needed to coordinate an urgent intervention to definitively control hemorrhage. Critical Care — MTP lead may request transfer of a patient to a critical care unit for further management or monitoring. • GENERAL — A recognized need for uncross matched RBC’s. — Substantial and rapid blood loss (>1,500 cc estimated blood loss or at least six units of RBC transfused with anticipated ongoing uncontrolled hemorrhage in the short term (mins to hours). — Anticipated massive and rapid blood loss requiring transfusion of ≥6 units of RBC within mins to hours. • TRAUMA — Penetrating trauma and persistent hypotension (two measurements of SBP <90 mmHg 5 mins apart in the Emergency Department). — Blunt trauma AND persistent hypotension AND one of the following: (a) Massive hemothorax (b) Positive FAST (c) Pelvic fracture
The Coagulopathic Trauma Patient
and Massive Transfusion Protocol
Jameel Ali, Sandro Rizoli and Katerina Pavenski
Chapter Overview
The Coagulopathy of Trauma
Platelet dysfunction
Fibrinogen consumption and fibrinolysis
Triggers for Massive Transfusion Protocols and the Protocols
The protocols
Point of care parameters and their measurement
to guide massive transfusion
Massive Transfusion Protocol Logistics
MTP TEAM-Roles and responsibilities
Responsibilities during MTP
MTP Activation criteria
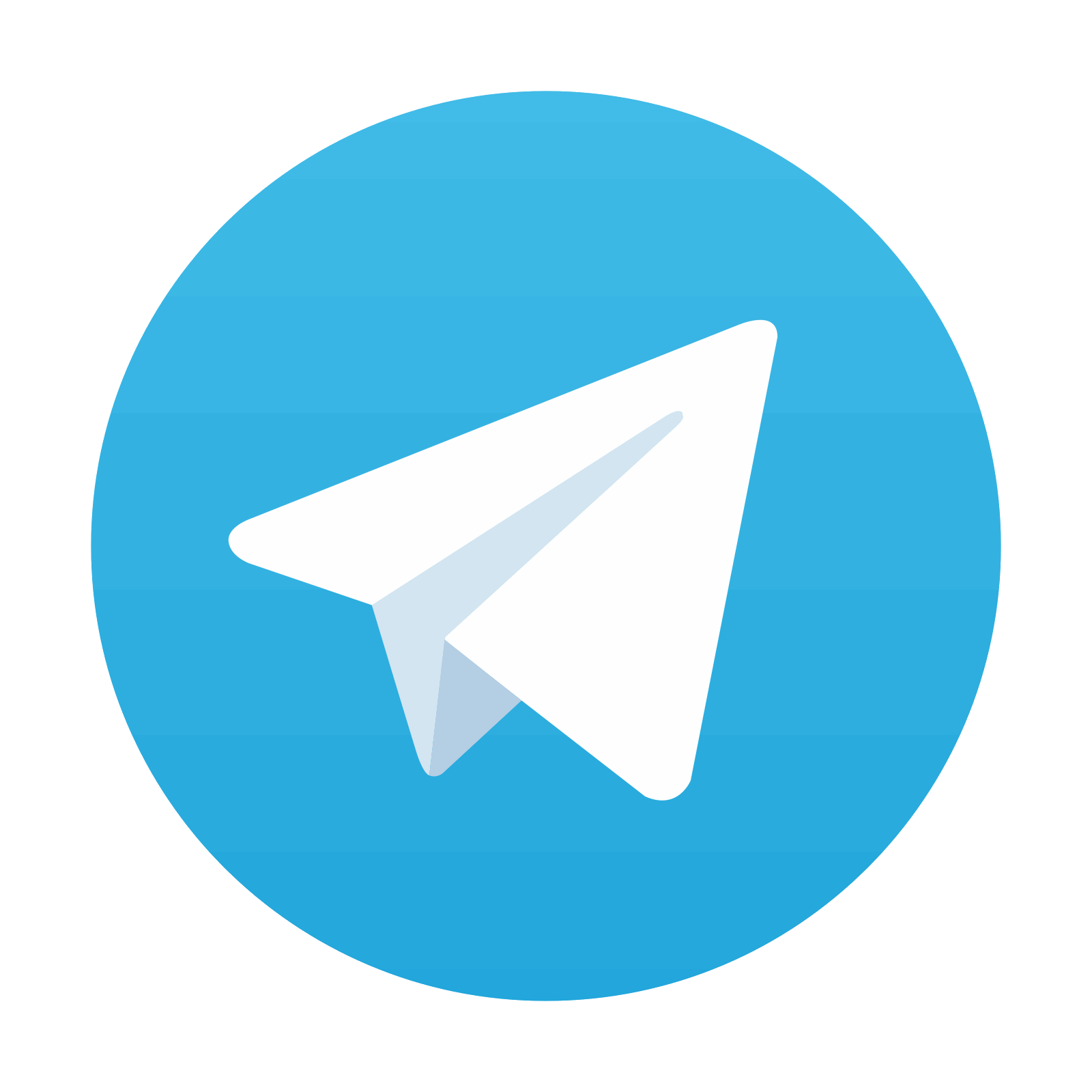
Stay updated, free articles. Join our Telegram channel

Full access? Get Clinical Tree
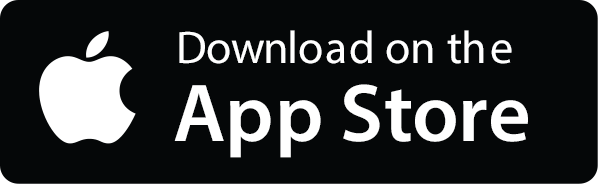
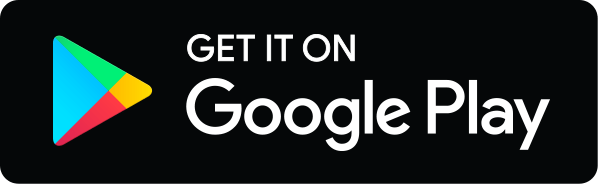