Solid organ transplantation is the surgical procedure in which an organ is removed from a one body (donor) and placed into another body (recipient). Solid organs that have been successfully transplanted are liver, kidneys, pancreas, intestine, heart, and lungs. Before organ transplantation can be considered, there must be failure of the native organ. Each organ failure is associated with specific morbidities and for some organs the severity of these morbidities is associated with the recipient’s place on the candidate waiting list. There is also an association with death that is either direct (after complete organ failure) or indirect (from complications of organ failure). Some organs have a method of support that are advanced from a technological vantage point, but biologically they are primitive and rudimentary. The risks of these supportive therapies should be weighed against the risks of surgery and immunosuppression. Ideally, patients would have a choice and transplant physicians would be able to guide them in their decision. However, the demand for all organs outstrips the supply, engaging the transplant physician in a complicated ethical dialogue on a daily basis while patients wait on candidate lists. Each specialty has its own formula for determining who should receive the next available organ, but all struggle with the shortage of organs.
The organ shortage means that organ support is the only option for many individuals awaiting transplantation. Xenotransplantation as a bridge remains a concept, not a reality. Patients with chronic renal failure may spend years on hemodialysis or peritoneal dialysis. Patients with diabetes mellitus from endocrine pancreatic insufficiency are medically managed with insulin replacement therapy. Patients with heart failure may be connected to ventricular assist devices while they await their new heart. For respiratory failure, support may culminate in noninvasive or invasive mechanical ventilation, but it often starts with supplemental oxygen therapy. Artificial liver support remains experimental. Patients with fulminant hepatic failure are more likely than patients with chronic liver disease to benefit from liver replacement therapy. The vast majority of patients with liver disease, however, have chronic liver disease and develop acute-on-chronic liver failure. Complications such as intractable ascites and variceal hemorrhage are caused by portal hypertension and are not corrected by treatment with an artificial liver or a liver dialysis machine. Hepatic encephalopathy before or after a transjugular intrahepatic portosystemic shunt (TIPS) may be amenable to artificial liver support, but it is very unlikely that this therapy would ever replace pharmacologic management with lactulose and oral antibiotics. The development of total parental nutrition (TPN) has increased the survival of short bowel patients; however, when central venous access becomes an issue, intestinal transplant is the only option for long-term survival.
Types of Donors
The quality of the donor organ as well as preoperative medical history of the recipient must be known to manage a postoperative transplant patient. Organs come from both deceased and living donors. Donors may have been pronounced dead by fulfilling brain death criteria (donation after brain death or DBD) or by cardiorespiratory criteria (donation after cardiac death or DCD).
The concept of brain death was created during the infancy of transplantation; however, the practice of pronouncing someone dead because of absent brainstem function was already accepted in many cultures. The controversy that still exists is brain death criteria may differ by country. The brain-dead individual is ideal for organ recovery because organs are removed under controlled circumstances. This limits warm ischemia time, which is when organs undergo the greatest injury. Cold ischemia time is the time after removal of the organ when it is cooled and perfused with preservation solution. Presently, most organs recovered for transplantation are DBD.
The first heart and liver donors were people pronounced dead by DCD, a method of retrieving organs for transplantation that is becoming popular again. When organs are recovered from these donors, sometimes called non–heart-beating donors, there is always a period of warm ischemia depending on each transplant center’s preferences, rendering the organs less desirable for transplantation. However, because the supply of organs is limited, donor organs that are more marginal than desired are being transplanted into patients. These organs, classified as expanded-criteria organs (ECD), have specific donor or graft characteristics that increase the probability of poor graft function postoperatively and that are ultimately associated with poor graft or patient survival. Recipients must consent to these marginal organs, but this may be the only choice for many on a waiting list.
Many transplant experts believe that the only meaningful answer to the organ shortage will come from increasing the number of living donors. The first living donor was a living related kidney transplant performed in Boston, Massachusetts, between identical twin brothers in 1954. The first successful living donor liver transplantation was performed between an adult and a child in 1989 in Brisbane, Australia, and between two adults in 1994 in Tokyo, Japan. In the United States, living donation increased until the first death of a living liver donor in 2001. For a decade following this tragedy, living donation decreased; however, according to the Organ Procurement and Transplantation Network (OPTN), there has been a slow increase in the recent years largely because of organ shortages.
Immunosuppression
Advances in solid organ transplantation correspond with the advancement of immunosuppression therapies. Many of the early transplants may have been surgically successful, long-term graft function and survival of patients was not achieved until the development of calcineurin inhibitors. The goal of immunosuppressive therapy in solid organ transplant patients is to prevent rejection while limiting the adverse effects of the drugs, which include direct drug effects and secondary effects of immunosuppression (e.g., infection and cancer). The major classes of immunosuppressive drugs are corticosteroids, antimetabolites, specific lymphocyte-signaling inhibitors, and antibodies. Combination therapy decreases the likelihood of adverse effects. Despite five decades of transplantation experience, achieving the delicate balance between immunosuppression to prevent rejection of the transplanted organ and immunocompetence to prevent opportunistic infections and cancers remains a naive science. During the course of transplantation, different immunosuppressant medications are used in different amounts and at appropriate times. In the initial phase of organ transplantation, there are more cellular and cytokine mechanisms that may lead to rejection; therefore, immunosuppression levels are maintained at a very high level in the beginning. This is also called the induction phase. Later, once recovered from organ transplantation, patients can be maintained on less immunosuppression medications called the maintenance phase. During episodes of rejection, higher dosages of some of the maintenance immunosuppression drugs may be used, or other drugs may be used, or both. Immunosuppressant regimens for the induction phase, maintenance phase, and rejection episodes are not standardized, and they vary widely by institution.
Corticosteroids indiscriminately downregulate the immune system, in particular, the expression and secretion of many proinflammatory cytokines. It is ironic and telling that this drug class, which has the least specificity for the immune system, remains part of the scaffolding for all transplant regimens. Corticosteroids are given in high dosages, followed by a taper for induction and for the first episode of acute cellular rejection in most paradigms.
The calcineurin inhibitors cyclosporine and tacrolimus are the mainstays of immunosuppression. They work by inhibiting the lymphocyte signaling pathways that lead to interleukin-2 (IL-2) transcription. Cyclosporine and tacrolimus traverse the cell membrane and combine with cytoplasmic cyclophilin and FK binding protein (FKBP), respectively. Either one of these complexes inactivates calcineurin, an essential component of the enzyme complex that dephosphorylates nuclear factor for activated T cells (NFAT). NFAT traverses the nuclear membrane and ultimately prevents the transcription of IL-2. The major adverse effects in the early postoperative period include both neurotoxicity and nephrotoxicity. Both tacrolimus and cyclosporine cause a renal arteriolar vasoconstriction that may be reversible with low-dose intravenous nicardipine.
A newer drug class of agents, the mTOR inhibitors sirolimus (rapamycin) and everolimus, work through different cellular mechanisms. Like tacrolimus, these agents also bind to FKBP and this complex then combines with mTOR (which stands for molecular target of rapamycin) and prevents protein translation from mRNA. Calcineurin is not involved in this reaction. Sirolimus and everolimus do not have the degree of neurotoxicity and nephrotoxicity seen with the calcineurin inhibitors and they are often used instead of, or in combination with, calcineurin inhibitors when renal function is of concern.
Antimetabolites is another class of immunosuppressive agent that is often used in combination with calcineurin inhibitors and/or mTOR inhibitors. Azathioprine, a prodrug that is converted to mercaptopurine, is an example. It is nonspecific and affects all rapidly dividing cells. Because lymphocytes are rapidly dividing cells, they are preferentially affected, which decreases the immune response. Intestinal cells divide rapidly and are injured by azathioprine, leading to the adverse side effect of diarrhea. The more tailored antimetabolites are mycophenolic acid and its prodrug mycophenolate mofetil, which has greater bioavailability. These drugs enzymatically inhibit the formation of the nucleoside guanosine, preferentially in T and B cells, avoiding some of the side effects of the azathioprine.
Antibody agents are used both for induction and for rejection. A polyclonal antibody called antithymocyte globulin is prepared by injecting rabbits with human thymocytes. The resulting antibody then attacks human T cells. It is often used as rescue therapy when cellular rejection develops. Basiliximab is a chimeric mouse–human monoclonal antibody to the IL-2 receptor of T cells. Basiliximab competes with IL-2 to bind to the IL2 receptor on the surface of the activated T lymphocytes and thus prevents the receptor from signaling. This prevents T cells from replicating and also from activating B cells, which are responsible for the production of antibodies. Alemtuzumab is a monoclonal antibody that binds to CD52, a protein present on the surface of mature lymphocytes, but not on the stem cells from which these lymphocytes are derived. After treatment with alemtuzumab, these CD52-bearing lymphocytes are targeted for destruction. All antibody therapies can cause the release of cytokines and patients need to be monitored closely during administration. Finally, physical removal of antibodies can be performed with plasmaphorisis.
Liver Transplantation
Thomas Starzl completed the first successful human liver transplant in 1967 after experimenting for 7 years with dogs. More than 40 years later, over 1 million liver transplants have been performed worldwide.
Recipient
Candidates for liver transplant have end-stage liver disease as a result of multiple etiologies varying by global region. According to United Network for Organ Sharing (UNOS) and OPTN databases, nonalcoholic steatohepatitis, alcoholic hepatitis, and hepatitis C virus were found to be the most commonly cited etiologies of chronic liver disease in adult patients (18 years and older) in the United States. Allocation of livers is primarily determined by the severity of disease according to Model for End-stage Liver Disease (MELD) score, which consists of serum bilirubin, creatinine, and International Normalized Ratio (INR) for prothrombin time. It was initially developed to predict mortality within 3 months of surgery in patients who had undergone a transjugular intrahepatic portosystemic shunt (TIPS) procedure. The pediatric equivalent is the PELD, which also takes into account albumin and growth failure. The morbidity of liver cirrhosis include both direct and indirect factors, which include coagulopathy, portal hypertension resulting in ascites with possible spontaneous bacterial peritonitis and gastrointestinal bleeding from varices, poor nutritional status, adaptive immune dysfunction, cardiomyopathy, and associated renal, pulmonary, and hematologic dysfunctions, which consequently make these patients poor surgical candidates. Patients and their families should be well informed about the mortality and morbidity of the albeit life-preserving nature of a liver transplant.
Donor
Liver grafts can be from DBD, DCD, or living donors and can be used as a whole or partial graft. Graft function is determined by several donor-related risk factors for primary nonfunction and initial poor function, which includes moderate steatosis, cold ischemia time greater than 12 hours, and donor age over 50 years. Warm ischemia time is also a known risk factor, with 16%–26% increased risk of biliary system complications in DCD grafts. Other donor-related factors include duration of intensive care, renal function, size/body weight index, hemodynamic stability, and blood transfusions. Living donor grafts can be from the left lateral or the right lobe depending on the size of liver needed for the recipient. The risk of living donor death and complications are both 1%–2%.
Postoperative Management
The first attempted liver transplant was performed by Thomas Starzl in 1963. The recipient was a child with biliary atresia, who died intraoperatively because of massive hemorrhage. Bleeding remains the first major concern after the operation. The management of postoperative bleeding after hepatic transplantation is similar to the management of postoperative bleeding after any surgical procedure. The first priority is to determine whether the bleeding is surgical or nonsurgical. There is a heightened urgency after liver transplantation, because survival of the graft or the patient may be impacted by ischemic damage sustained in these early hours. It must be rapidly determined whether there is any surgical bleeding that would require reexploration. A negative reexploration is likely to do less harm than graft injury from poor perfusion. If the recipient is bleeding, plasma and platelets should be given to raise the platelet count and correct the coagulopathy. Procoagulant-like substances including α-aminocaproic acid, desmopressin (aqueous vasopressin), and recombinant activated factor VII may be given, although data are lacking to support the routine use of these agents. During this time, the recipient must be monitored closely for the possible development of abdominal compartment syndrome. Elevated peak airway pressures may be the first clue to this diagnosis. If this possibility seems likely before the transplant operation is complete, it may be worthwhile to leave the fascia open initially and to only close the skin. Later, the fascia can be closed in a staged second operation.
A careful record should be made of the operation, with specific details of the events during reperfusion of the allograft, such as the clearance of lactate, the appearance and production of bile, and the need for hemodynamic presser support. Because lactate is converted to pyruvate in the liver, early clearance of lactate is evidence of graft function, even if bile production is not observed. As liver function improves, clearance of lactate increases. The more common scenario of overproduction of lactate in a critically ill patient, as pyruvate is shunted to lactate because of tissue hypoxia, may also be present in the post-transplant patient. Circulating lactate concentrations in liver transplant recipients may represent either end of this metabolic spectrum. Another marker of early liver function after transplantation is the quality and quantity of bile measured through an externalized biliary drain. Intraoperative vasoactive pressor agent infusion is often necessary during allograft reperfusion, and it is usually weaned within several hours postoperatively. An ongoing requirement for pressors to maintain hemodynamic stability often implies graft dysfunction.
Circulating transaminase levels typically peak approximately 24 hours after operation. The release of these enzymes is a manifestation of ischemic liver injury and reperfusion injury. If the transaminase levels continue to rise after 24 hours, or if encephalopathy does not clear, or a nonsurgical coagulopathy develops, or lactic acid does not clear, the patient may have primary nonfunction (PNF) of the allograft and needs a retransplant urgently. This diagnosis is one of exclusion, however, and hepatic artery and portal venous thrombosis must be ruled out by duplex ultrasonography (US) first. Postoperative management has little or no impact on this devastating complication.
Vascular complications may also be devastating in the immediate postoperative period. The most common early vascular complication is hepatic artery thrombosis (HAT). Some advocate routine ultrasound to screen for this problem. Concern for HAT is heightened if the resistive index (RI) on US is high, even if there is flow. The normal range is 0.6 to 0.9, and a low RI value is most consistent with HAT. If HAT is suspected on US, then the diagnosis should be confirmed angiographically. Return to the operating room for restoration of hepatic artery flow is dependent on timing. In many cases, surgical correction still results in biliary complications and eventual need for retransplantation. When patients sustain HAT, biliary complications are likely to occur in the future because flow to the bile canaliculi is dependent on the hepatic artery and not the portal vein. Collaterals from the diaphragm that traverse the bare area of the liver offer a second circulation route to the bile canaliculi. These vessels are transected during the transplantation. This phenomenon appears to be the reason that hepatic artery occlusion is better tolerated in the nontransplant patient than in the transplanted patient. Biliary complications can include biliary strictures and the biliary cast syndrome, which are often managed with endoscopic stents and/or percutaneous drains. Immediate post-transplant portal venous thrombosis is a less common but more life-threatening complication. An urgent surgical restoration of portal flow should be undertaken. Another vascular complication may be a technical error that causes Budd–Chiari syndrome, which also needs surgical correction in the immediate postoperative period.
The liver is a forgiving organ for acute cellular rejection in the immediate postoperative period and, when rejection does occur, it is usually easily managed with corticosteroids. Low-grade fever and elevated transaminase levels are often clues, but a high index of suspicion and confirmation of the diagnosis with a liver biopsy are frequently required.
In recipients who receive an ABO-compatible but nonidentical liver, there is the possibility of developing passenger lymphocyte syndrome. In this scenario, a group O organ is transplanted to a non–group O recipient or a group A or B organ is transplanted to a group AB recipient. In this syndrome, the transplanted donor lymphocytes promote hemolysis. Biochemical features of this syndrome include indirect hyperbilirubinemia and an elevated serum lactate dehydrogenase concentration. The circulating haptoglobin concentration may be low, but because haptoglobin is synthesized by the liver, this test is unreliable in these circumstances. The direct antiglobulin test is positive. Hemolysis resolves over the course of weeks to months.
Many cirrhotic patients have renal dysfunction preoperatively and postoperatively. Recovery of renal function may depend as much on preexisting renal function as on any other factor, but the course of the operation and the quality of the donor liver are also important factors. Hepatorenal syndrome usually resolves postoperatively, but patients with preoperative renal dysfunction have a greater chance of chronic renal failure postoperatively. Hepatorenal syndrome can also evolve into acute tubular necrosis and the line between the two disorders is not always distinct. The pathogenesis of hepatorenal syndrome is not well delineated and is most likely multifactorial. The end result is splanchnic vasodilatation and intrarenal vasoconstriction. Patients with hepatorenal syndrome have low urine sodium concentration but do not respond to intravascular volume expansion. Early renal replacement therapy with ultrafiltration may help the liver transplant patient who is suffering from encephalopathy and fluid overload. However, encephalopathy is rarely caused simply by azotemia. If the liver transplant patient is fluid overloaded with normal arterial and central venous blood pressures, further volume resuscitation is usually not effective in reversing oliguria.
Kidney Transplantation
Although the scientific story of transplantation began when Alexis Carrel attempted transplantation of various organs in animals, the clinical story began in 1954, when Joseph Murray transplanted a kidney from one identical twin into the other. Originally, it was believed that renal transplantation would confer only lifestyle benefits associated with freedom from dialysis, but it is now clear that there is a survival advantage as well. Younger white patients without diabetes mellitus seem to benefit the most in this regard.
Recipient
Patients with end stage renal disease (ESRD) are referred for kidney transplantation when the estimated glomerular filtration rate (eGFR) is < 30 mL/min/1.73m 2 and is evaluated by the transplant program to detect and treat coexisting illnesses, which may affect perioperative risk and survival after transplantation, as well as transplant candidacy. Generally, kidneys are allocated by basis of time on the waiting list and HLA matching. In some regions, waiting time for a cadaveric graft is up to 10 years; therefor the living donor option is encouraged for most patients. Preoperatively, the kidney transplant recipient is screened for electrolyte abnormalities. Potassium levels of < 5.5 mEq/L are reasonable and even a short dialysis session of 1–2 hours may be enough to reduce the potassium levels acceptable for hemodynamic control during anesthesia.
Donor
Kidney grafts can be from DBD, DCD, and living donors. Deceased donor graft quality is assessed using the Kidney Donor Risk Index (KDRI) scoring system. The original KDRI accounted for 14 donor and transplant factors, each found to be independently associated with graft failure or death; however, a modified scoring system is currently used by OPTN that includes: donor age, history of hypertension, history of diabetes, serum creatinine, cerebrovascular cause of death, height, weight, donation after cardiac death, and hepatitis C virus status. Cadaveric grafts for adult recipients can be a single kidney, dual adult kidneys that are from extended criteria donors (ECD), or en bloc dual pediatric kidneys.
Living donor graft can be from related donor or as a donor exchange or chain. Living grafts have better graft function and survival than cadaveric grafts. Donors are evaluated to exclude major contraindications such as active malignancy, hypertension with end-organ damage, and uncontrolled psychiatric conditions. Other relative contradictions differing by center preferences includes advanced age, obesity, pre-diabetes, some vascular diseases, substantial proteinuria, and recent nephrolithiasis amongst others. Once donors are accepted, CTA is performed to determine vascular anatomy of the kidneys. Most often, the left kidney is selected for donation and laparoscopic donor nephrectomies are the standard of care. Donors generally have an uneventful postoperative course and have little risk of mortality and morbidity from donation. Evidence suggests that the 15-year relative risk of ESRD for a living donor is 0%–5%.
Postoperative Management
The major postoperative complication associated with kidney transplantation is delayed graft function. Primary nonfunction can occur as it does for livers after liver transplantation and may necessitate transplant nephrectomy (removal of the transplanted organ). Both oliguria and increasing plasma creatinine concentration are signs that indicate delayed graft function. The classic categorization of renal dysfunction as prerenal failure, intrarenal failure, and postrenal failure remains useful for classifying the etiology of delayed graft function.
Prerenal delayed graft function can be caused by intravascular volume contraction. The nephrotoxic effects of cyclosporine or tacrolimus also can contribute to delayed graft function. These immunosuppressive agents cause renal arteriolar vasoconstriction that can be distinguished from the effect of intravascular volume contraction by the lack of response to a volume challenge. An additional area of concern is the vascular anastomoses. Postoperatively, both the arterial and venous anastomoses may develop thromboses, a vascular emergency that requires urgent ultrasound evaluation, leading to surgical reexploration as appropriate. Venous thrombosis is more common than arterial thrombosis, but either can endanger the survival of the allograft.
The most common cause of intrarenal delayed graft function is early cell-mediated rejection. Hyperacute (humoral) rejection and accelerated acute (humoral and cellular) rejection are less common today than in the past because of sensitive cross-matching techniques. Early cell-mediated rejection is the most common form of rejection in the postoperative period. However, it must be differentiated from acute tubular necrosis (ATN), and a renal biopsy is the only definitive way to do this. If the cause of delayed graft function is early cell-mediated rejection, it is necessary to adjust and augment the immunosuppressive regimen, and the most common strategy is to increase the dose of corticosteroids and antilymphocyte antibody. Delayed graft function increases the risk of cell-mediated rejection. Additionally, delayed graft function is more likely to lead to allograft failure in patients with a 0-antigen match than in those with a 6-antigen match.
Another cause of intrarenal delayed graft function is ATN. It is a common cause of renal dysfunction among hospitalized patients, including renal transplant recipients. The incidence of ATN after renal transplant is higher when the organ came from a cadaveric donor rather than from a living donor. Indeed, when ECDs are used, it may be appropriate to increase the renal mass and give one patient both of the donor’s kidneys. The logic of this approach has been well documented in an animal model of renal transplantation.
Postrenal causes of delayed graft function are often related to the creation of an ureteroneocystostomy, which may become evident because of leaks or obstruction. Although stenting can be attempted to remediate these problems, reexploration is often necessary. A leak may be first noted because of delayed graft function, but it is sometimes diagnosed by the presence of fluid from a drain or the wound that has a higher concentration of creatinine than is present in plasma.
Lymphoceles and hematomas may also develop in a critical location or to a large size to cause both prerenal and postrenal delayed graft function. These may develop to compress the graft’s vascular supply or ureter externally and may require drainage. Drainage can be approached percutaneously or surgically by open or laparoscopic techniques.
Pancreas Transplantation
The first successful pancreas transplant was performed in 1966 by Richard Lillehei and William Kelly in Minnesota, US. Until about 1990, the procedure was considered experimental. Now it is a widely accepted therapeutic modality. Pancreas transplantation has seen a decline in the last 10 years with more technologically advanced and easy to use methods of glucose monitoring and insulin delivery; however, dual pancreas and kidney transplantation numbers have remained stable since the turn of the century.
Recipient
Pancreas transplantation is considered as therapy only in patients who have a history of frequent, acute, and severe metabolic complications (hypoglycemia, hyperglycemia, ketoacidosis) requiring medical attention, clinical and emotional problems with exogenous insulin therapy that are so severe as to be incapacitating, and consistent failure of insulin-based management to prevent acute complications. Pancreas allocation policy is similar to the kidney allocation policy taking into account time on the waiting list and the suitability of HLA matching. Additional variations occur for dual pancreas and kidney transplantations because the kidney transplant can be from a cadaveric or living donor. Pancreas transplant recipients are generally young and healthy without other morbid medical conditions; however, careful attention to metabolic derangements need to be provided by anesthesia because surgery can increase secretion of cortisol, catecholamines, glucagon, and growth hormone affecting intraoperative glucose control.
Donor
Pancreas grafts can be cadaveric whole grafts from DBD or DCD donors or segmental grafts from living donors. Islets of Langerhans transplantation into the liver can be an autograft or allograft and is still experimental. It has been used successfully to treat patients with chronic pancreatitis who require a total pancreatectomy with autotransplantation of islets. Deceased donor pancreas graft should be without signs of acute pancreatitis, glandular edema, hematoma, fatty infiltration and/or hardened consistency.
Postoperative Management
Pancreas transplantation has several surgical variations. The location of the vascular anastomosis may be to the external iliac vessels, aorta and IVC, or aorta and a portal vessel. The exocrine drainage may be anastomosed directly to the donor pancreas in the case of a segmental graft or with a segment of donor duodenum in the case of cadaveric graft to the recipient’s jejunum or bladder. Whatever the technique, the postoperative management is the same with the major postoperative complications being related to graft failure, which includes vascular and enteric anastomotic leaks, rejection, and pancreatitis.
Pancreatic function is difficult to monitor. Increasing circulating glucose concentration is the best clinical marker of pancreatic graft dysfunction. C-peptide can also be monitored closely. If dysfunction is suspected in the first 2 weeks after transplantation, arterial thrombosis must be in the differential diagnosis. Doppler ultrasonography should be completed urgently. The presence of a necrotic pancreas can lead to systemic inflammatory response syndrome and secondary acute respiratory distress syndrome. It is often wise to perform a transplant pancreatectomy rather than risk the morbidity and mortality associated with these serious complications.
If an exocrine anastomotic leak is suspected, computed tomography (CT) should be performed to look for extraluminal contrast or air and surgical repair is necessary. Further therapy is dictated by the means used to achieve drainage of the exocrine pancreas (enteric or bladder). Revision of the duodenojejunostomy to a Roux-en-Y anastomosis is a common approach to deal with an anastomotic leak with an enteral drainage system. These leaks may result in peritonitis from contamination of the peritoneal cavity with gastrointestinal contents. For bladder drainage, decompression with a Foley catheter is usually sufficient. A follow-up cystogram should be obtained when the patient is better.
All patients develop some degree of pancreatitis after transplantation. Routinely monitored amylase and lipase levels should peak and begin to decline in the first several postoperative days. If glucose control continues to be poor and/or pancreatitis persist, cell-mediated rejection must be gauged in the first weeks. After exclusion of other causes, a pancreatic biopsy may become necessary. The possibility of cytomegalovirus infection can be investigated at that time and treated with antiviral therapy as necessary. Rejection requires enhancement of steroids and the administration of antilymphocyte thymoglobulin.
Intestinal Transplantation
The first attempted intestinal transplant in humans was in 1964 by Ralph Deterling in the United States. In the following two decades, further attempts were universally met with failure as patients died of technical complications, sepsis, and graft failure largely resulting from severe rejection and graft-versus-host disease. However, the discovery of calcineurin inhibitors revolutionized the field and in 1988 Eberhard Deltz performed the first successful living donor intestinal transplant in Germany and David Grant performed the first successful cadaveric intestinal transplant combined with a liver in Canada. In the United States, nearly three thousand intestinal transplants have been reported in the OPTN database. There are three types of intestinal transplants: isolated intestinal graft, a combined intestine and liver graft, and multivisceral graft, which can include stomach, colon, liver, pancreas, and kidney.
Recipient
The indication for intestinal transplant is intestinal failure with failure of medical management. This includes loss of venous access for parental nutrition, multiple episodes of life-threatening sepsis, uncontrollable fluid and electrolytes abnormalities, growth and development failure in children, and TPN-induced liver disease. Etiologies of intestinal failure differ by age and range from congenital diseases (microvillus disease, Crohn disease, generalized Hirschsprung disease, pseudo-obstruction), neonatal and pediatric bowel complications requiring multiple resections (necrotizing enterocolitis, gastroschisis, intestinal atresia, small bowel volvulus), and adult abdominal catastrophes (trauma, internal volvulus following gastric bypass, thrombosis of mesenteric vessels). Most intestinal transplants are complex because of multiple previous abdominal surgeries. Candidates should be optimized preoperatively to limit anesthesia complications from metabolic and electrolyte imbalances. Additionally, intravenous and central venous access should be well mapped out prior to transplantation because many of these patients have poor veins from multiple peripheral and central venous lines.
Donor
Intestinal and multivisceral grafts should be from DBD or living donors. Donors are ideally young otherwise healthy with stable hemodynamics because the small bowel is very sensitive to ischemic injury. Recipient’s antibody profile is rigorously taken into account when considering a donor graft because the intestine is a highly immunogenic organ and rejection is one of the most common complication. Additionally, because intestinal recipients may have had previous surgeries that may have resulted in loss of abdominal domain, donor size matching is also important.
Postoperative Management
The surgical technique varies widely depending on the type of graft, but all patients should have central line access, nasogastric tube, feeding tube, ileostomy as well as other standard drains and tubes for close monitoring and management. Early surgical complications include infection, vascular thrombosis, bleeding, dehiscence, and fistula. The care of the intestinal portion of the transplant is the same as any other general surgery patient who had bowel surgery albeit with a heightened suspicion for infection, anastomotic dehiscence, and primary nonfunction. For a multivisceral transplant, the care of the other organs is similar to that discussed previously for the specific graft. Ultrasound or CT evaluations should be promptly performed for any suspicious abnormalities in laboratory evaluations or concerning physical examinations and explored surgically as indicated by the imaging evaluations.
Acute rejection (AR) is the most common complication that can be caused by or exacerbated by an already present surgical complications. AR is a clinical diagnosis, which is then confirmed with endoscopy through the ileostomy with pathological evaluation of biopsies. A wide range of laboratory tests are routinely taken including weekly B-cell and T-cell subsets, IgA, IgG, IgM, Calprotectin, ESR, CRP, CMV, and EBV as well as the standard daily hematology and chemistry labs to aid in the clinical diagnosis. Subtle physical examination findings such as low-grade fevers and/or high enteric output from NGT or ileostomy can also indicate rejection. Cell-mediated AR is most common and treated with increased levels of tacrolimus and steroid pulse per institutional protocol. Other forms of immunological complication are antibody-mediated rejection, graft versus host disease, and inflammatory bowel disease.
Heart Transplantation
The French surgeon Alexis Carrel, in collaboration with American physiologist Charles Guthrie, is credited with performing the first canine heart transplant in 1905. American surgeon James Hardy performed the first human heart transplantation using a chimpanzee xenograft on January 23, 1964, at the University of Mississippi. The first human-to-human heart transplant was performed in 1967 by South African heart surgeon Christiaan Barnard in Cape Town, South Africa, but unfortunately, the patient died 18 days later from complications arising from pneumonia. Three days later, a New York heart surgeon Adrian Kantrowitz performed the first pediatric heart transplant at Maimonides Hospital in Brooklyn, New York, on an 18-day-old male infant. The infant survived only 6.5 hours and died of severe metabolic and respiratory acidosis. A month later, in 1968, Norman Shumway who was widely regarded as the father of heart transplantation performed the first adult human-to-human heart transplant in the United States at Stanford Hospital using techniques he had perfected in the laboratory with Richard Lower. The recipient died of multiple systemic complications after 15 days. Over the next several years, poor clinical results resulted in a worldwide moratorium on heart transplantation. However, as a result of the persistent and pioneering efforts of Shumway with his colleagues at Stanford and the emergence of a new immunosuppressive agent cyclosporine, there was a resurgence of heart transplantation in the 1980s as a viable therapy for end-stage cardiomyopathy. Currently, more than 2200 heart transplantations are performed annually in the United States.
Recipient
Heart transplantation is considered the “gold standard” therapy for refractory heart failure (HF). The relative scarcity of donors has expanded the indication for and use of mechanical circulatory assist devices such as ventricular assist devices (VAD) and total artificial heart (TAH). Both are used as a bridge to transplantation and destination therapy. Pharmacological therapies, cardiac defibrillators, and devices for cardiac resynchronization therapies have improved the prognosis of patients with left ventricular systolic heart failure. Unfortunately, in spite of the combined use of the best therapies, heart failure progressively advances in the vast majority of cases. In some cases, patients become completely unresponsive to conventional treatments to the extent that even surgical revascularization, ventriculoplasty, and mitral valve surgery are inadequate to rectify the underlying myocardial dysfunction. Patients with acute HF requiring inotropic therapy (milrinone and/or epinephrine) have an approximately 6-month mortality of 25% based on clinical trials and registries on acute HF.
The typical clinical profile of a patient with refractory heart failure often exhibits some of the following characteristics despite optimal medical and pharmacological management: (1) severe symptoms (New York Heart Association [NYHA] Class III to IV); (2) episodes with clinical signs of fluid retention and/or peripheral hypoperfusion; (3) objective evidence of severe cardiac dysfunction that can be demonstrated by at least one of the following: left ventricular ejection fraction (LVEF) < 30%; restrictive mitral inflow pattern at Doppler echocardiography; high left and/or right ventricular filling pressures; and elevated B-type natriuretic peptides; (4) evidence of systemic organ injury, in particular renal and hepatic dysfunction, underlined by an increase in creatinine and bilirubin levels; (5) severe impairment of functional capacity demonstrated by either an inability to exercise (a 6-minute walk test distance < 300 meters) or a peak oxygen uptake < 12–24 mL/kg/min; and (6) history of hospitalization related to heart failure in the previous 6 months. The prioritization of appropriate recipients for transplantation is based on survival and quality of life expected to be gained in comparison with maximal medical and surgical alternatives. Highest priority is given to Status IA. As of 2007, the patient distribution of status is 50% (IA), 36% (IB) and 14% (II).
Since October 2018, the adult heart allocation for the recipients is classified into statuses 1-6 based on the urgency with which the recipients need a heart, with status 1 being those who need a heart most urgently, status 6 being the least urgent, and status 7 being inactive. On the other hand, the pediatric heart allocation remains the same as prior to October 2018 and has statuses 1A (most urgent), 1B (less urgent) and 2 (least urgent). More specific details of the statuses can be found on the Organ Procurement and Transplantation Network (OPTN) website at https://optn.transplant.hrsa.gov/learn/professional-education/adult-heart-allocation/ .
Donor
Once a brain dead individual has been identified as a potential donor, the patient undergoes a rigorous screening regimen. The cardiac surgeons and/or cardiologists of the recipient team search for potential contraindications, determine the hemodynamic support necessary to sustain the donor, and review the echocardiogram, electrocardiogram, chest X-ray and arterial blood gas (ABG). Even when adverse donor criteria are reported, a team is often dispatched to the hospital to evaluate the donor on site. The final and most important screening of the donor occurs intraoperatively at the time of organ procurement by the cardiac surgical team. Direct visualization of the heart is performed for evidence of right ventricular or valvular dysfunction, previous myocardial infarction, and myocardial contusion. The coronary arterial tree is palpated for any gross atherosclerotic disease. If the heart examination is unremarkable, the recipient surgeon is notified and the heart procurement is performed. Coronary angiography is indicated in the presence of advanced donor age (traditionally male donors > 45 years of age and female donors > 50 years of age). Angiography should be performed if there is a history of cocaine abuse or the donor has three risk factors for coronary artery disease (CAD) such as hypertension, diabetes mellitus, smoking history, dyslipidemia, or family history of premature CAD.
Postoperative Management
Postoperative care is determined in part by the medical state of the recipient and in part by the quality of the donor organ. Thus, the care of heart transplant recipients is dictated by their hemodynamic status and degree of end-organ dysfunction prior to transplant.
Postoperative bleeding can be more of a concern in cardiac transplant patients than in typical postcardiotomy patients for several reasons. Transplant recipients may have been therapeutically anticoagulated preoperatively and this treatment may not have been reversed adequately prior to sternotomy. Need for reoperation or prolonged time in the operating room and on cardiopulmonary bypass (CPB) can increase the risk of coagulopathy. Treatment of bleeding caused by coagulopathy consists of administration of blood products and clotting factors.
The goal of hemodynamic support is to maintain systemic perfusion with an appropriate cardiac output (CO) while preventing right heart failure or overload. Appropriate management requires the use of a pulmonary artery catheter to evaluate CO and central venous and pulmonary artery pressures.
The intact heart is innervated by antagonistic sympathetic and parasympathetic fibers of the autonomic nervous system. Transplantation necessitates transection of these fibers during donor cardiectomy, with a consequent denervated heart with altered physiology. Devoid of autonomic input, the sinoatrial node of the allograft fires at an intrinsic rate of 90–110 beats per minute. The allograft relies on distant sites as its source of catecholamines and consequently its response to stress (e.g., hypovolemia, hypoxia, and anemia) is somewhat delayed. There is absence of a normal reflex tachycardia in response to venous pooling and thereby there is increased frequency of orthostatic hypotension.
Denervation of the transplanted heart leads to loss of autonomic nervous system modulation of heart’s electrophysiologic properties. Sinus or junctional bradycardia occurs in up to half of all transplant recipients. Risk factors for sinus node dysfunction include prolonged organ ischemia, angiographic nodal artery abnormalities, bi-atrial anastomosis, preoperative amiodarone use, and rejection. Atrial fibrillation, atrial flutter, and other supraventricular arrhythmias have been reported in 5%–30% of patients after heart transplantation. Supraventricular tachycardia in transplant patients should be treated in the same manner as in nontransplant patients but with lower doses.
Donor myocardial performance is transiently depressed in the immediate postoperative period. Allograft injury associated with donor hemodynamic instability and hypothermic ischemic insult of preservation result in reduced ventricular compliance and contractility. Infusion of inotropes including epinephrine, milrinone, dobutamine, and norepinephrine is routinely initiated in the operating room. Because of depletion of myocardial catecholamine stores with prolonged inotropic support of the donor, the allograft often requires high doses of catecholamines. The inotropic support is often necessary for several days. In patients with pretransplant continuous flow VADs, the resulting vasoplegia requires vasopressor support. Cardiac denervation has several consequences including a chronotropic and inotropic supersensitivity to exogenous catecholamines. Restoration of normal myocardial function permits cautious weaning of inotropic support within 5–7 days.
Systemic hypertension should be treated to prevent unnecessary afterload stress on the allograft. In the early postoperative period, intravenous sodium nitroprusside or nitroglycerin is administered. Preoperative renal insufficiency owing to chronic heart failure and nephrotoxic effects of calcineurin–inhibitor-induced renal insufficiency will usually resolve with a reduction in dose.
Early cardiac failure accounts for 20% of perioperative deaths of heart transplant recipients. The cause of primary graft failure is multifactorial. The most important etiologies are myocardial dysfunction owing to donor instability, pulmonary hypertension, ischemic injury during preservation, and occasionally acute rejection. Mechanical cardiac support with an intra-aortic balloon pump, VAD, or extracorporeal membrane oxygenation (ECMO) can be used in patients who are resistant to pharmacologic interventions; however, this has been associated with increased mortality.
Chronic right heart failure is frequently associated with elevated PVR and the unprepared donor right ventricle may be unable to overcome this increased afterload. Right heart failure remains a leading cause of early mortality. Initial management involves pulmonary vasodilators such as inhaled nitric oxide and nitroglycerin. Pulmonary hypertension refractory to this sometimes responds to PGE1 or prostacyclin. IAPB and RVAD (temporary) can also be used in patients unresponsive to medical therapy. ECMO support for several days with an open chest has been successful to allow time for graft recovery in cases of inadequate biventricular function.
Cardiac allograft rejection is the normal host response to cells recognized as non-self. The vast majority of cases are mediated by the cellular limb of the immune response through a cascade of events involving macrophages, cytokines, and T-lymphocytes. Antibody mediated rejection (AMR) also known as humoral rejection is less common but more difficult to diagnose. Although 85% of episodes can be reversed with corticosteroid therapy alone, rejection is still a major cause of morbidity in cardiac transplantation.
Right ventricular endomyocardial biopsy remains the gold standard for diagnosis of acute rejection. Most frequently used technique for orthotopic allografts is percutaneous approach through the right internal jugular vein. Interventricular septal specimens are fixed in formalin for permanent section, although frozen sections are performed occasionally if urgent diagnosis is necessary. Biopsies are performed initially 7–10 days in the early postoperative period and then eventually at intervals of 3–6 months in the first year. Suspicion of rejection warrants biopsies. Peripheral blood gene expression profiling is an exciting new tool for this field. The AlloMap test has been cleared by the US Food and Drug Administration and widely implemented since 2006 to help rule out acute cardiac allograft rejection.
Hyperacute rejection results from preformed donor specific antibodies in the recipient. ABO blood group and panel reactive antibody screening have made this a rare complication. Onset of hyperacute rejection occurs within minutes to several hours after transplant. Gross inspection reveals a mottled or dark red, flaccid allograft and histological examination confirms the characteristic global interstitial hemorrhage and edema without lymphocytic infiltrate. Immediate plasmapheresis, intravenous immunoglobulin, and mechanical cardiac support are instituted and retransplantation may be the only successful strategy.
Lung Transplantation
The first clinically attempted lung transplant in humans was reported by James Hardy and Watts Webb in 1963 followed by others with short survival times, except for one case by Fritz Derom in Belgium that same year. In 1986, the Toronto Lung Transplant Program reported the first truly successful single-lung transplantations for two patients with pulmonary fibrosis. The same team went on to perform several other firsts: the first successful double-lung transplant, the first with an en bloc technique that used a tracheal anastomosis, and the first bilateral sequential transplantation technique that not only improved airway healing, but also had the additional benefit of avoiding cardiopulmonary bypass.
Recipient
Candidates for lung transplantation have end-stage lung disease with a life expectancy of less than 2 years. Medical management has not been able to help these individuals. Exclusionary criteria include coexisting diseases that would adversely affect survival after transplantation, such as significant cardiac disease, cirrhosis, and malignancy. One-year adjusted graft survival (2001) was 77% and has been essentially unchanged over the past 9 years (75% graft survival in 1993). Patient survival was only slightly higher than graft survival. Retransplantation is rare (2% in 2002). One- and 5-year patient survivals were 78% and 45%, respectively.
Allocation of donor lungs is determined more by waiting-list time than by geographic area and blood compatibility issues. As a result, patients are often listed early in their course and, if necessary, can become inactive on the list without losing their previous place in line. Patients in their 40s, 50s, and 60s account for the majority of transplant recipients. The largest cohort (between 50 and 64 years old) represents nearly 56%. Lungs are allocated based on the Lung Allocation Score (LAS) introduced in the United States in 2005 and later in Europe and the rest of the world. The LAS estimates the severity of each candidate’s illness and chance of success following a lung transplant. All candidates are placed in order for compatible lung offers according to their score: a candidate with a higher lung allocation score will receive higher priority for a lung offer when a compatible lung becomes available in the same geographic zone. More specific calculation of the LAS can be found on the UNOS website at https://unos.org/wp-content/uploads/unos/Lung_Patient.pdf .
The major primary diagnoses are emphysema, idiopathic pulmonary fibrosis, cystic fibrosis, alpha-1-antitrypsin deficiency, and primary pulmonary hypertension. In 2002, emphysema accounted for 39% of the lung transplants performed. The majority of recipients are not hospitalized at the time of transplantation. Recipients (from 2002 cohorts) in the intensive care unit (ICU) immediately prior to transplantation had significantly lower graft survival rates at all time points, compared with non-ICU and nonhospitalized cohorts.
Patients with emphysema should be considered for transplantation when the postbronchodilator forced expiratory volume in 1 second (FEV1) is less than or equal to 25% of predicted. Single lung transplantation is most commonly performed, although sequential double lung transplantation is offered at some centers. Native lung hyperinflation may occur in the immediate postoperative period, which can be difficult to manage especially when the patient is on mechanical ventilation. For this reason, patients with significant bullous disease are often offered double lung transplants. Another option is lung volume reduction surgery on the native lung to control the underlying disease.
Single lung transplantation is effective treatment for idiopathic pulmonary fibrosis (IPF). Decreases in lung compliance and perfusion of the native lung helps to maintain ventilation–perfusion (V/Q) matching of the transplanted lung for IPF.
Primary pulmonary hypertension can be treated with both single and double lung transplantation and, at times, heart–lung transplant. Centers that advocate double lung transplants argue that there is greater recovery of right ventricular function because of maximal reduction in PVR for primary pulmonary hypertension.
Timing a transplantation for patients with cystic fibrosis is difficult. Malnutrition and colonization with resistant bacteria and fungi complicate decisions about the timing, the choice of single or double lung transplantation, and postoperative management.
The recipient operation can be done without the use of CPB, but CPB is scheduled when the patient has pulmonary hypertension and right heart failure. Single lung procedures are done via thoracotomy with exposure of the left atrium, transection of the bronchus and vascular structures, and then anastomosis of the donor bronchus, left atrium, and pulmonary artery. Double lung transplantation has evolved into sequential single lung procedures via a sternotomy with bilateral subcostal incisions.
Donor
Lung graft can be from a living donor, DBD, or now with renewed interest, DCD. Proper selection of the donor organ is crucial when evaluating lung donors. Most cadaveric donors have undergone a traumatic or other stressful situation that has resulted in extensive resuscitation and mechanical ventilation. Trauma patients could have sustained direct injury to the lung. Therefore, many potential donors are excluded from donating lungs because of pulmonary edema, pneumonia, contusions, or adult respiratory distress syndrome (ARDS).
Living-donor lungs account for a very small number of transplants. Interestingly, the majority of living-donor lung recipients are in the ranges of 11 to 17 years and 18 to 34 years, consistent with recipients having a diagnosis of cystic fibrosis.
Harvesting of the lungs is accomplished via median sternotomy with preservation of the organs with a cold pulmoplegia solution via the main pulmonary artery with simultaneous cardioplegic harvest of the heart.
Postoperative Management
The focus in the following paragraphs is on the underlying disease processes of patients being evaluated for lung or heart–lung transplantation because the baseline pulmonary disease plays a significant role in postoperative management. The decision to do a single or double lung transplant will affect the treatment strategy for both the native and the transplanted lungs. In addition, surgical approaches, with their intricacies, will alter management and pose different problems.
The goals of postoperative care are the same for any patient: appropriate ventilatory weaning and extubating, early mobilization and nutrition, and prevention of infection by limiting the use of invasive lines and unnecessary antibiotics. The goals of respiratory management are no different from those of any ICU patient: ventilatory support to maintain adequate oxygenation and ventilation without causing barotrauma or oxygen toxicity.
As discussed earlier, single lung transplantation for patients with emphysema results in differential compliance and V/Q mismatch. The native lung is more compliant than the donor lung, resulting in air trapping and hyperinflation. Overexpansion of the native lung shifts the lung toward the mediastinum, further decreasing compliance to the allograft. This phenomenon is more likely to occur when the allograft is placed on the left side, because the native organ (in the right thorax) is restricted from expanding in the caudal direction by the liver. Reperfusion injury to the donor lung may further compromise compliance to the allograft. Therapies to combat such a V/Q mismatch include placement of a double-lumen tube and independent ventilation of the two lungs with lower tidal volumes and prolonged expiratory times to the native lung. Positioning the donor lung side upward may aid in improved ventilation to the allograft. With resolution of pulmonary edema from reperfusion injury to the allograft, single-lumen tube placement and unified ventilation can be successful and ventilatory weaning can be initiated.
Differential perfusion to both organs can also occur with single lung transplantation for primary pulmonary hypertension. The donor lung in this situation has lower pulmonary pressures resulting in preferential blood flow to the transplanted organ. The increase in circulating blood volume, in turn, results in pulmonary edema to the allograft, further contributing to a potentially edematous lung from reperfusion injury. As with severe compliance differences, significant perfusion mismatch may need to be managed by independent ventilation of the two lungs with higher positive expiratory pressures to the allograft. Increases in intra-alveolar pressure will reduce the pressure gradient across the capillary bed and help to control pulmonary edema.
Patients who undergo double lung transplants usually do not have significant issues with V/Q mismatch unless there is a difference in ischemia or the preservation of one of the two allografts. However, pulmonary edema from reperfusion injury can occur in both lungs. This would manifest as hypoxia and decreased compliance. The mechanisms of reperfusion injury are believed to be caused by long ischemia time, poor organ preservation, or immune-mediated injury. Long CPB times, large-volume resuscitation, and extensive use of blood products can add to edema of the lungs. In managing this condition, it is reasonable to use the same maneuvers as those used to support patients with ARDS. All of these patients should be ventilated with low tidal volume ventilation (6 mL/kg of patient’s ideal bodyweight).
All lung transplant patients require aggressive pulmonary care including frequent suctioning, bronchodilators, deep coughing, postural drainage, and incentive spirometry. Ventilated patients may require daily bronchoscopy to maintain good pulmonary toilet. Lung transplant patients are kept in a state of relative hypovolemia with judicial use of fluids, blood products, and inotropic support. These therapies are maintained while minimizing prerenal azotemia and maintaining adequate end-organ perfusion. Aspiration can be a deadly complication and needs to be avoided at all costs. Aspiration precautions and swallowing evaluations are part of general care. Patients receiving enteral feedings should be maintained with head of bed elevation at 30 degrees and have feedings stopped well in advance of planned extubations.
Anastomotic complications can have severe consequences for the lung transplant patient in the early postoperative period. These can be described as partial or full-thickness injuries ranging from necrosis and dehiscence to ulceration and formation of granulation tissue. Delayed complications include stricture formation and bronchomalacia. The etiology of anastomotic complications is believed to involve ischemia. Bronchial arteries, which are fed by the aorta and intercostal arteries, are transected with procurement of the lung and are not reestablished with surgical implantation. The bronchial and tracheal anastomosis relies on retrograde flow through the pulmonary artery to collaterals from the bronchial arteries. A lung donor bronchial stump adds to the vulnerability of the anastomosis. Dissection and devascularization of the recipient’s main bronchus up to the level of the carina further compromises anastomotic blood flow. Poor perfusion can be accentuated by hypotension, use of high-dose vasopressors, hypovolemia, poor organ preservation, long ischemia time, infection, and immunosuppression.
Necrosis and dehiscence typically occur in the first few weeks after transplantation. Circumferential and large dehiscence must be treated as a surgical emergency, with debridement and reanastomosis of the bronchus. Coverage of the anastomosis with viable tissue and extensive drainage to treat pleural and mediastinal contamination is necessary. Localized areas of necrosis and dehiscence without pleural communication can be treated conservatively. A removable stent can be placed to act as scaffolding for secondary healing. Weeks to months later, stricture formation may result from a healed necrotic or dehiscent area. Strictures are managed by dilation or laser resection. Stenting with wire or Silastic stents may be a necessary adjunct for the treatment of strictures. Airway obstruction caused by granulation tissue is usually remedied by laser ablation with stent placement if there are recurrent and chronic problems. Bronchomalacia is characterized by airway collapse with obstruction on expiration. It can also be controlled with stent placement to support the involved area of the airway.
Airway complications are reported to occur in 5% to 12% of cases at experienced transplant centers. The sequelae of ischemia to the bronchial anastomosis are preemptively treated by the surgical management of the anastomosis at the time of implantation. Specifically, shortening of the donor bronchus to two or fewer cartilaginous rings proximal to the upper lobe take-off minimizes the watershed area of ischemia. Reinforcing the anastomosis with a vascularized flap (omentum, pericardium, or intercostal muscle) also helps prevent anastomotic leaks. An intussuscepting anastomotic technique is performed at some transplant centers to help to accommodate size mismatch between the donor and recipient airway and to help to avoid extensive devascularization of the bronchus.
Phrenic nerve injury is a potential intraoperative complication of lung transplantation. This is especially true in cases of repeat thoracotomy because of extensive pleural adhesions. Bilateral lung transplantation increases the risk of nerve damage. Nerve damage may be temporary or permanent and can be caused by stretching, crushing, thermal damage, or transection of the phrenic nerve. Phrenic nerve paralysis is a devastating complication that interferes with good pulmonary hygiene and may prevent independence from the ventilator.
Rejection as discussed here relates to the early postoperative period. Hyperacute rejection occurs immediately on reperfusion and is caused by preexisting antibodies to donor tissue. It is a rare event because of the routine screening of recipients and ABO matching. Acute rejection is a cell-mediated process and can occur during the first 30 days after transplantation. It is diagnosed by both clinical and histologic criteria. In the early postoperative period, the histologic criteria are not very distinct and are difficult to separate from infection or from reperfusion injury. Therefore, the clinical presentation becomes crucial in the diagnosis. Symptoms of acute rejection include dyspnea, fatigue, dry cough, low-grade fever, malaise, and pulmonary infiltrates without evidence of infection. Chronic rejection is manifested by obliterative bronchiolitis. This complication is caused by a fibroproliferative process that causes obliteration of tubular structures of the transplanted lung, similar to coronary artery vasculopathy seen in the transplanted heart.
Infections
Many infections can develop in the solid organ transplant (SOT) patient after weeks to months on immunosuppression. In the immediate postoperative period, however, it is imperative to watch for the usual bacterial pathogens. Many of these patients were very sick preoperatively and are now susceptible to nosocomial infections, especially hospital-acquired pneumonia, ventilator-associated pneumonia, catheter-associated urinary tract infections, and catheter-related bloodstream infection. As a result of prolonged hospitalization before transplantation, many of the bacterial pathogens are resistant organisms and antibiotic therapies should be guided by definitive cultures and sensitivities. Catheters and tubes of all types should always be removed from patients as soon as possible. Indeed, these necessary tubes may be more responsible for the high incidence of postoperative infection than early immunosuppression.
Prophylactic antibiotics are used against toxoplasmosis, Pneumocystis pneumonia (PCP), fungal infections, and viral infections in the early months after SOT. Toxoplasmosis and PCP are covered by the same prophylaxis antibiotic. Toxoplasmosis can be a life-threatening opportunistic infection in all SOT with heart being the most frequently affected organ. Either the infection is transmitted to the recipient by an infected organ or it is a reactivation of a latent seropositive infection in the recipient. The median point of disease onset is estimated to be two months. PCP has been known to become epidemic in several transplant units worldwide with mortality as high as 60% and is now classified as a fungus. Prophylaxis antibiotics most frequently used are pyrimethamine-sulfadoxine, trimethoprim and sulfamethoxazole, co-trimoxazole, and atovaquone. These drugs have various levels of myelosuppression and are often interchangeably used depending on the patient’s tolerance profile. Length of prophylaxis is dependent on risk of infection and can range from 1–12 months.
Candida and Aspergillus species are the most frequent causes of fungal infection followed by Cryptococcosis and non- Aspergillus molds. Evidence suggests fungal prophylaxis is most evidence-based for liver, intestine, and lung transplant patients although most institution use fungal prophylaxis for all transplants. Azoles such as fluconazole, itraconazole, and voriconazole have been used among transplant recipients. Some lung regimens include dual therapy with aerosolized amphotericin B. Duration of therapy varies with the type of organ with abdominal solid organs ranging from 1 to 6 weeks and thoracic organs ranging from 1 to 6 months.
Viral infections are emerging as having the most deleterious effects on SOT recipients. Cytomegalovirus (CMV) is one of the major microbial pathogens in SOT, but it is most notable in heart and lung transplantation. Ganciclovir and valacyclovir have been used as prophylaxis for a duration of 3–12 months. Fortunately, both antiviral agents provide prophylaxis against other viral pathogens such as varicella-zoster virus (VZV) and Epstein-Barr virus (EBV). Treatment of hepatitis B virus (HBV) and hepatitis C virus (HCV) especially after liver transplant is controversial. Some institutions will give hepatitis B immunoglobulin (HBIG) followed by a regimen of Retuximab when transplanting recipients who have HBV. HCV drug treatments such as Zepatier and Harvoni are highly effective at eradicating HCV with the result that most patients are treated and cured before transplant. HCV positive donors are a potential source of organs and prophylaxis regimen are being developed to use these ECD grafts safely. Finally, all SOT recipients should receive yearly influenza virus vaccines and prompt treatment with oseltamivir for early infections.
Two organs deserve special mention in regard to bacterial infection. First, the liver is prone to biliary infections, particularly after hepatic artery thrombosis. Abscesses may require drainage. If the infection is confined to the liver, retransplantation is a viable option. Second, pneumonia in a transplanted lung could have disastrous consequences, therefore these patients must be observed closely for any signs and symptoms of pulmonary infection.
Conclusion
The solid organ transplant recipient requires unique postoperative care. The quality of the organ is determined by the health of the donor and by postmortem factors such as warm and cold ischemia time and events at the surgery, including but not limited to, reperfusion. The preexisting health of the recipient also plays a role postoperatively. For patients with diabetes mellitus and renal failure, comorbid conditions include coronary artery disease. For the patient with cirrhosis, years of muscle wasting may leave the patient profoundly weak. Postoperative decisions that would be appropriate for nontransplant patients may be inappropriate for transplant patients. For example, transient hypoxemia or hypotension might be tolerated in other patients, but these problems can lead to early damage to the graft in transplant recipients. It is easy to ignore these issues in the early postoperative period but they are essential to the long-term outcome of the graft and, of course, the patient.
References
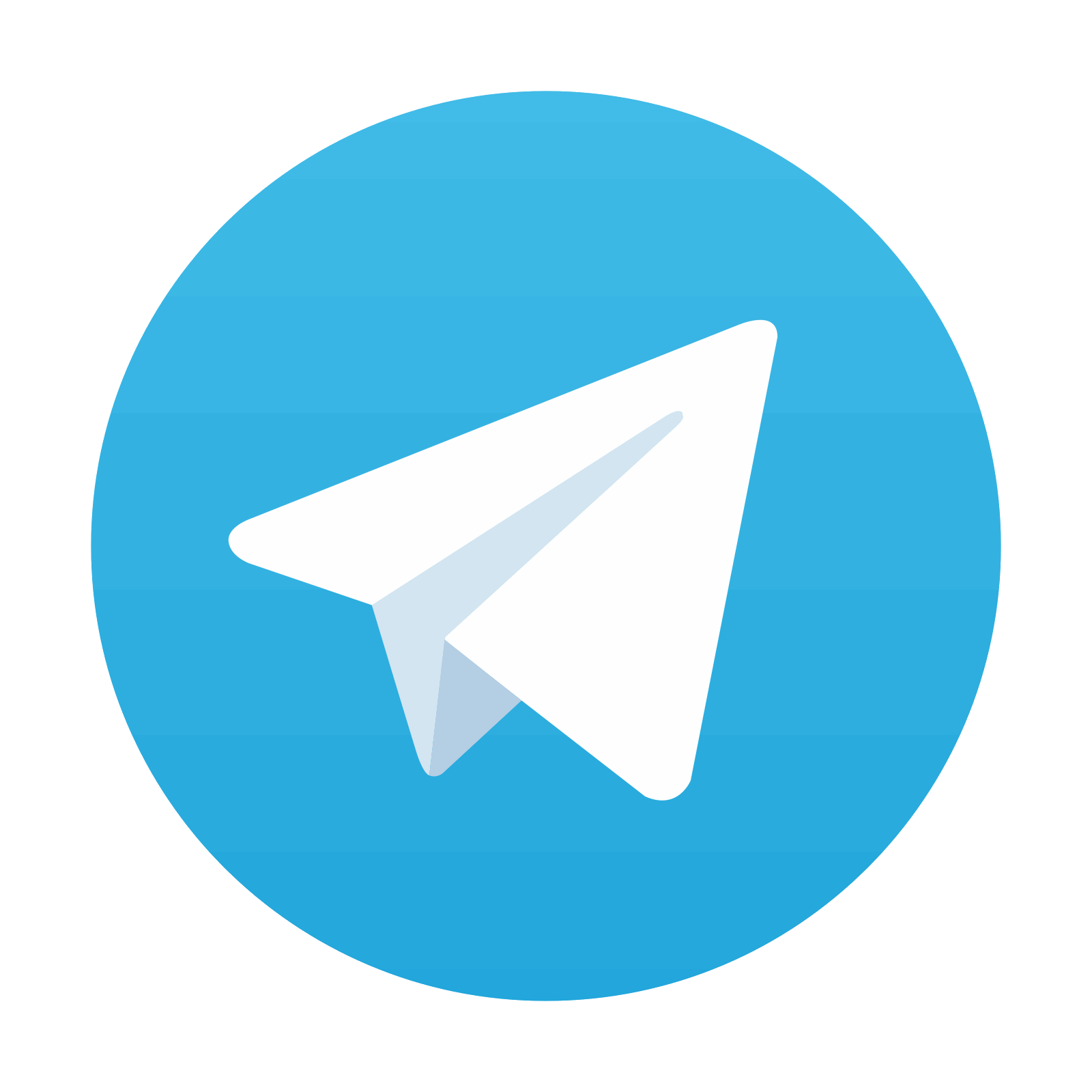
Stay updated, free articles. Join our Telegram channel

Full access? Get Clinical Tree
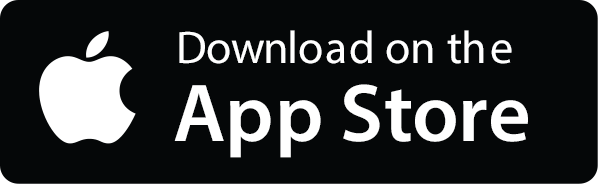
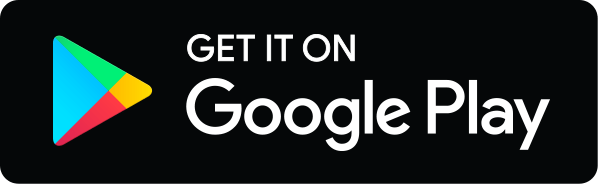
