Fig. 38.1
Mock CT scanner. The scanner is a 1:10 size scale model of the clinical scanner. The small size allows children to prepare for the actual scan by placing a doll or stuffed animal on the table and moving it through the gantry
Several studies have been performed evaluating the utility of mock MRI in preparing children for MRI and evaluating its effect on the need for anesthesia [1, 2]. In the largest study, a mock MRI program was able to reduce the need for sedation by almost 17% in patients aged 3–8 years old [1]. It is likely that such programs would benefit patients with anxiety, claustrophobia, or other limitations when undergoing MRI.
Fluoroscopy is another modality where demonstration is often used. The fluoroscopic environment is often frightening to patients as they are forced to lie down on a hard table in a dark room while a large camera comes down close to their body. The group of medical personnel standing over them performing the study can also be intimidating. Because of the way the test is performed, the patient is rarely sedated; yet, they are subject to invasive and sometimes painful procedures. Demonstration cannot completely prepare a child for this scenario, but it can help them to understand what will happen during the study.
Child life specialists are an invaluable resource in helping children prepare for fluoroscopic procedures in an age-appropriate manner. Before the study is performed, the child life specialist welcomes the child and brings him or her to the fluoroscopy suite. Once there, the child life specialist shows the patient the fluoroscopy machine and how it works. Patients are allowed to touch the machine and the table. Then, the child life specialist explains the procedure to the patient using a picture book where a doll is shown to be the patient. Depending on the child and his or her level of understanding and anxiety, the child life specialist can then demonstrate what the child will experience by using the doll (Fig. 38.2). The child is able to feel all tubes or catheters that will be used during the procedure so that he or she is better prepared once the radiologist enters the room.
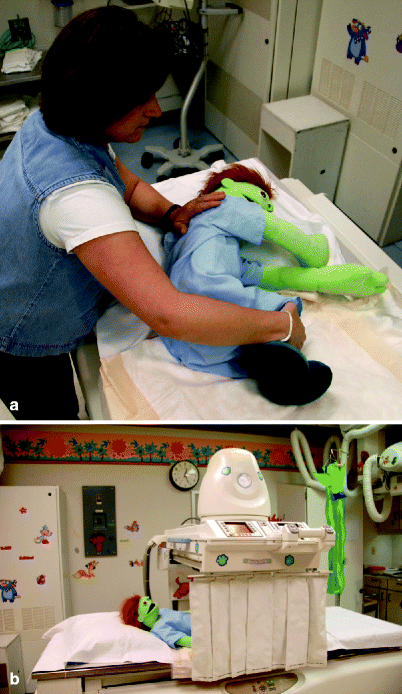
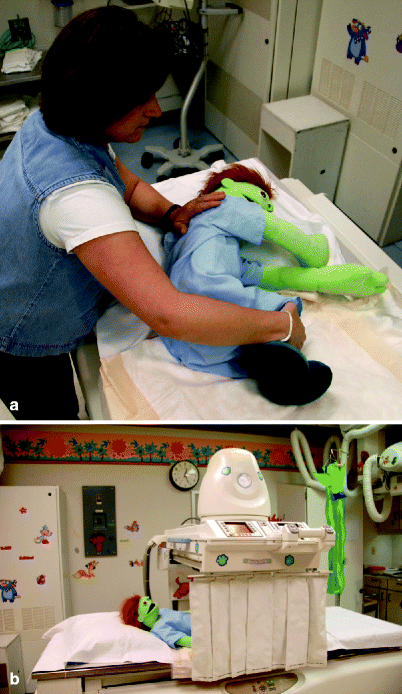
Fig. 38.2
(a) Dolls can be used to help prepare children for different examinations. In fluoroscopy, child life specialists can demonstrate correct positioning for a contrast enema using a doll. (b) Dolls can also be used to show children how the machines works
Practice is another method that is used extensively to help simulate the procedure and help the patient prepare for an exam. An example of practice is in preparing patients for MR elastography (MRE). In this procedure, the patient undergoes an MRI while a 7-in. plastic speaker, called the passive driver, is placed on the patient. The passive driver is connected via pneumatic tubing to the active driver, an audio subwoofer, in the MRI control room. In order to prepare patients for the vibration sensation that they will feel during scanning, child life specialists use a vibrating passive driver simulator on the child prior to the MRE (Fig. 38.3). The pre-scan simulation helps prepare patients for the sensation they will feel during scanning and helps to reduce patient anxiety and sudden movements at the start of the actual MRE sequence.
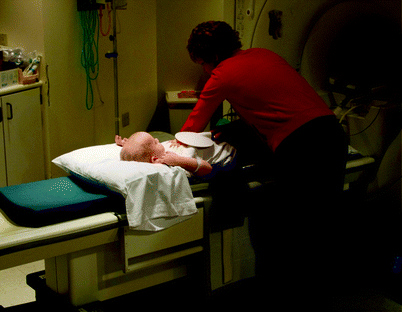
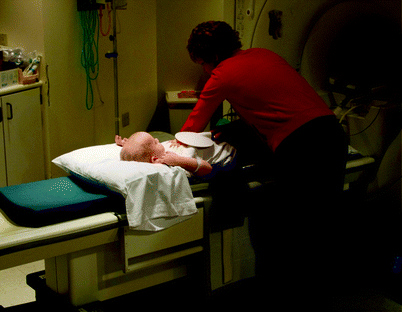
Fig. 38.3
In order to obtain high-quality images, it helps to prepare patients for unexpected or unusual sensations. In MR elastography, child life specialists prepare children for the vibrations they will feel by placing the passive driver on the abdomen prior to scanning
Practice is often coupled with demonstration in an attempt to prepare patients for a procedure. What is practiced often depends on the invasiveness of the procedure. In noninvasive procedures, patients are able to experience a large portion of what they will undergo in a nonthreatening and staged approach.
Using a full-scale mock MRI as an example, the following procedure has been described as a method to help prepare children for scanning [1]. First, the child and his or her caregiver are assessed for their level of anxiety. The responses then help to guide the remainder of education, practice, and demonstration. The child life specialist then uses age and developmentally appropriate methods to teach the patient about the procedure. This education can involve picture books, videos, listening to sounds recorded from the MR scanner, and demonstration with dolls. Finally, the patient is able to lie in the mock scanner, lie in a radiofrequency coil, and practice lying still. They are able to pick the music they would like to listen to or the movie that they would like to watch during scanning. In this scenario, they are able to simulate the entire scanning process prior to the scan itself.
In more invasive procedures such as a voiding cystourethrogram (VCUG) or contrast enema, it is not possible to practice the most difficult portions of the procedure. For these procedures, the child life specialist demonstrates the procedure through picture books or demonstration on dolls. The child is then placed on the fluoroscopy table where they are able to practice relaxation techniques and the positions in which they will be placed. Child life specialists use age-appropriate tools such as party blowers or bubbles not only to perform deep breathing during painful portions of the procedure but also as a means of distraction and play (Fig. 38.4). Prior to the procedure, children practice these techniques while on the fluoroscopy table to simulate as much of the procedure as possible.
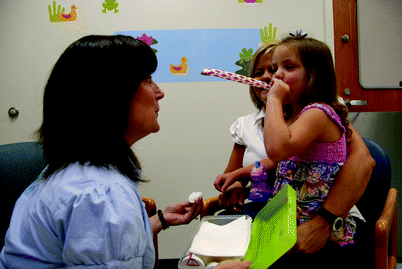
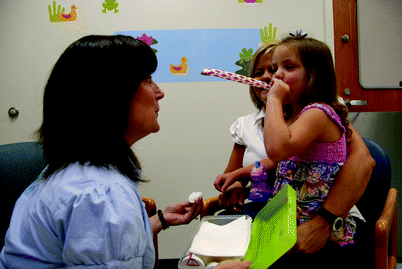
Fig. 38.4
Child life specialists use age-appropriate tools such as party blowers or bubbles to help children learn deep-breathing techniques for relaxation prior to undergoing a painful or anxiety-provoking procedure
The final technique of simulation used in patient preparation in pediatric radiology is play therapy. This is perhaps the most immersive technique in that children are able to become more comfortable with the procedure by simulating the examination through play. One of the benefits of play therapy is that a child life specialist can observe the child and identify their specific anxieties. They are then able to target education and relaxation techniques directly to this portion of the examination.
In preparation for CT or MRI, play therapy can be used as another method to reduce anxiety. Scale model scanners have been developed so that a doll or stuffed animal can undergo the scan (Fig. 38.1). The child is able to place his or her doll on the model scanner and push it through the machine. While they are playing, children will often explain the procedure from their point of view to their doll. Child life specialists are able to use this play to help explain specific parts of the examination to the child.
Play is often a large part of practice as well. For example, when practicing deep breathing with party blowers, child life specialists can turn the deep-breathing technique into a game. Instead of just blowing through the party blower, the children can aim for their parents or a technologist’s nose (Fig. 38.4). This can turn an anxiety-provoking scenario into a game where everyone in the room is laughing.
While demonstration, practice, and play therapy often help to prepare children for a procedure, the material used must be appropriate for the child. At least one study has suggested that these techniques may lead to an overall increase in anxiety. This study showed that in children 7–12 years of age, the preparatory material used led to increased questioning and anxiety by the child [7]. It is possible that the anxiety provoked in this study is an anomaly related to the techniques used as several other studies have shown that demonstration, practice, and play can be used to decrease anxiety and the need for sedation during a procedure [1–3, 6].
Simulators in Radiology: Preparing the Imaging Technology
Simulators are most extensively used in radiology to help test and calibrate the imaging modalities. Every radiology department uses phantoms as part of its check on quality control and quality assurance for each modality. Each test acts as a check on the performance or internal consistency of the machine with key outcomes related to image quality, sensitivity of the machine in detecting subtle lesions, the radiation output of the machine, or the reproducibility of findings. The type of phantom used varies depending on the machine that is being tested and the feature being evaluated. Phantoms are commonly used in radiography, CT, MRI, and nuclear medicine. Because each modality employs a number of different tests, many of which require an understanding of the physics of that specific modality, only a few tests on select modalities are described here. The modalities selected for this chapter are the focus of active research or are thought to be good examples of phantoms used to test the modality.
With the rapid increase in the use of CT for diagnosing and managing a wide variety of conditions, there has been considerable research performed to develop methods to increase image quality while at the same time decreasing the radiation dose to the patient [8]. Because each CT scan delivers radiation to the patient, it is impractical and unethical to perform this testing on patients. Two main methods have been developed to evaluate the radiation dose delivered by a certain study: phantom-based studies and computer simulation.
Phantoms can vary in complexity and sophistication depending on the measured variable. For CT, the simplest phantom is a water phantom [9]. This type of phantom is created by filling a structure with water. The water phantom works on the principal that water has a defined CT density (0 Hounsfield units). Any variation from this is the image noise. As the radiation used to acquire the image is decreased, the noise increases. The water phantom can thus be used to measure image noise.
Test phantoms can vary considerably. One type of phantom called a “performance phantom” allows various cylinders of different densities to be inserted into the phantom. This is also used to detect image noise as well as the reproducibility of measurements [10]. Low-contrast phantoms are used to assess the sensitivity of nodule detection. In these phantoms, there are multiple cylinders of varying diameter. Each cylinder has a density slightly higher than water. These phantoms work on the principal that as noise increases, the lesions will become more and more difficult to identify. Linearity phantoms are used to ensure that a known element has a specific density on CT (Fig. 38.5). In this phantom, there are multiple pins, each containing a known density. The pins are placed in a cylinder that has water density. The phantom is scanned and the density of each element is measured and plotted to ensure the accuracy of the CT numbers.
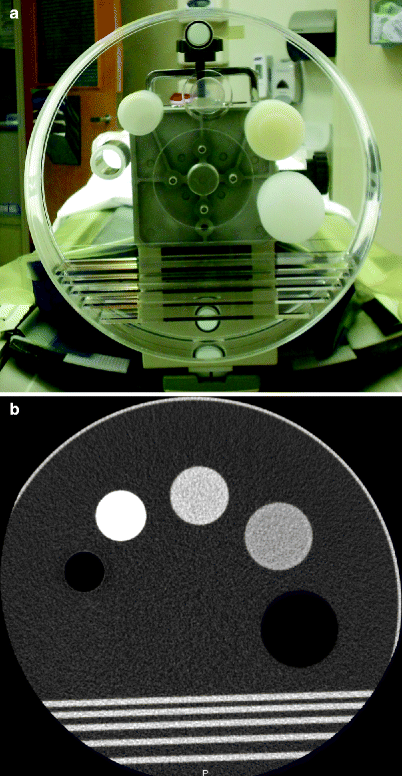
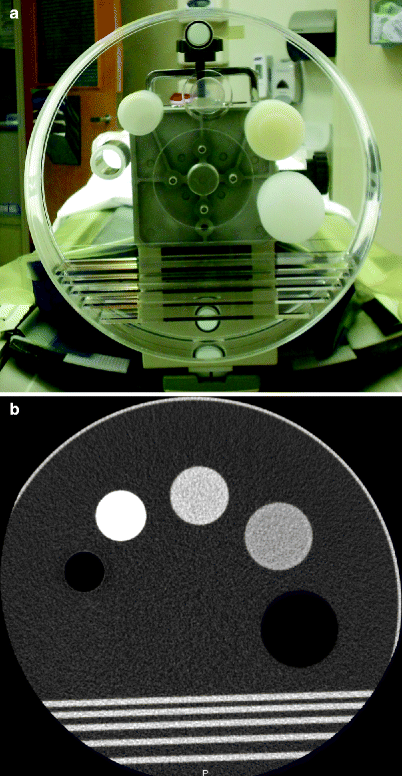
Fig. 38.5
(a) Photograph and (b) CT of a linearity phantom. Phantoms are used in every radiology department to help calibrate the modalities. Linearity phantoms are used for CT to ensure that the density of the structures meets a known standard
The most advanced phantom is the anthropomorphic phantom (Fig. 38.6). Anthropomorphic phantoms can be constructed in various shapes and sizes to simulate the differences between males and females or patients of different sizes and ages. Even though these phantoms are designed to simulate the human body in shape and internal appearance, they can vary considerably. Some anthropomorphic phantoms are designed for a specific application such as lung nodule detection [11], while others are used to evaluate the whole body [10, 12–14].
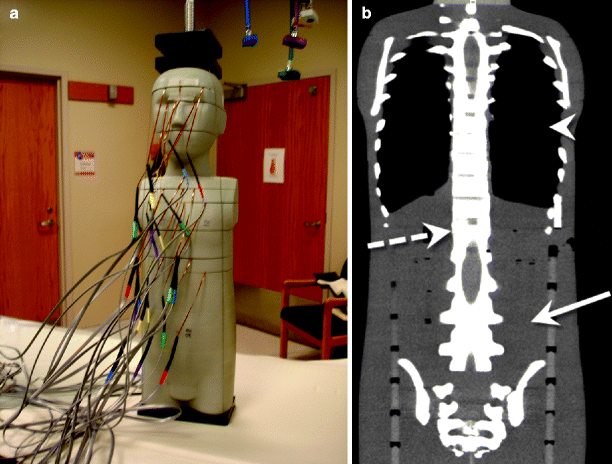
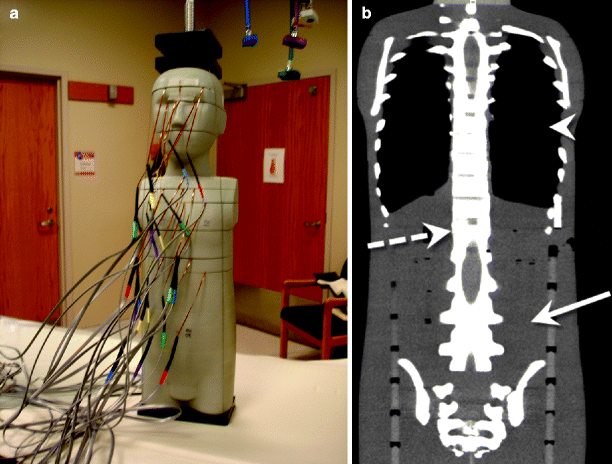
Fig. 38.6
(a) Anthropomorphic phantoms can vary in complexity depending on their purpose. The anthropomorphic phantom in this photograph has multiple leads attached to it in order to measure the radiation dose at different locations. (b) CT image of the same phantom shows soft tissue (arrow), bone (dashed arrow), and air-filled structures (arrow head), similar to a patient
The majority of the current research performed using anthropomorphic phantoms involves the calculation of radiation dose [12–14]. Anthropomorphic phantoms are useful for dose calculations because they take differences in body contour and different tissue interactions into account [14]. High-quality anthropomorphic phantoms will include all major internal organs. Phantoms used for dose calculations have multiple holes within them so that dosimeters can be placed within the phantom and organ doses can be measured. Using anthropomorphic phantoms in this manner allows for a standard method of calculating dose that is reproducible across different machines and differing technologies.
Some of the newest phantoms are entirely computerized. High-quality computerized phantoms help to address the main shortcoming of anthropomorphic phantoms, namely, the cost associated with fabricating the number of phantoms required to simulate the large range of patient sizes, clinical needs, and physiologic motion [8]. Computerized phantoms are therefore incredibly useful in “characterizing, evaluating, and optimizing CT imaging systems and image processing and reconstruction methods” [8]. The biggest disadvantage of computerized phantoms is that there is no physical object to scan; thus, the actual dose cannot be measured.
There are two main types of computerized phantoms: voxelized and mathematical [8]. Voxelized phantoms are based on patient data obtained from CT or MRI and appear very lifelike. They are useful in evaluating specific organs and image quality; however, their main disadvantage is that to simulate motion or anatomic variation, interpolation must be used, introducing error [8]. Mathematical phantoms use geometric shapes to construct organs. Because the shapes are predefined, they are easily manipulated to account for anatomic variation and motion. The major limitation of mathematical phantoms is the simplistic equations on which they are based. This limits exact modeling of human physiology [8]. As computers become more powerful, hybrid phantoms have been created that are based on patient imaging data and use more complex mathematical models to define the internal structures [8].
Because all modern radiologic imaging is digital, the images acquired as part of a clinical scan can be used for further simulation to evaluate the effect that changing imaging parameters has on image quality [15, 16]. Several hybrid studies have been performed where simulated noise has been added to clinical scans as a way to produce scans with noise equivalent to reducing dose. This method allows researchers to perform large-scale studies evaluating the effect reduced dose has on image quality and diagnostic accuracy [15]. The benefit of this data is that new imaging techniques can be evaluated and tested while the patient is imaged based on the current clinical standard.
Although not used to the same degree as CT, simulators have been created to optimize image quality for radiographs [17–19]. These simulators work on principles similar to those of CT simulators. Simulated images are created either through computer modeling, manipulating clinical images, or imaging phantoms of varying complexity.
Simulators in Radiology: Preparing the Radiologist
Simulators designed for radiologists are most akin to simulators in other fields of medicine. These simulators can be placed into two broad categories: those used to teach image interpretation and those used to teach a specific technique or procedure.
Diagnostic Simulators
Diagnostic radiology is ideally suited for simulation. All of the imaging and information related to its interpretation are digital. This makes reproduction of any imaging study for simulator use simple and likely effective as long as true representations are provided. Digital images allow creators of radiology simulators to create unique workflows for image interpretation such as creating digital “hotspots” to identify abnormalities. Further, radiologists are used to working in a digital environment so that a simulated environment can look and feel exactly like the real production environment, simply by the presentation of digital material.
Despite the relative ease with which simulation can be used in this field, simulation in diagnostic radiology has lagged behind other medical specialties. Most simulation is designed to teach a specific skill or technique. In radiology, diagnostic interpretation involves complex pattern recognition of a heterogeneous group of studies, involving multiple modalities and encompassing multiple body parts. Basic radiology interpretation involves creating a search pattern and methodically using this pattern every time to identify differences from the imagined standard (i.e., “normal”) for the imaged body part. The complexity of creating such a system to teach image interpretation is beyond the scope of most applications.
One could argue that another reason for the lag is that although simulation is used in radiology, it has not progressed into the digital era. Radiology education has traditionally included case conferences where the trainee is asked to interpret an unknown case in front of his or her peers. This type of education plays an important role in radiology because it allows a large group of trainees to see rare cases that may not be encountered during their training. Case conferences allow the educator to identify key pertinent positive and negative findings based on the discussion by the trainee. This type of simulation is helpful in that other trainees can observe the process their peers use to form a differential diagnosis. The disadvantage of this method is that only one trainee can actively participate at a time. This unknown case format has been transmitted into the digital era via cases of the day or week on popular radiology websites such as the American College of Radiology’s Case in Point (http://www.acr.org/HomePageCategories/CaseInPoint.aspx), AuntMinnie.com’s Case of the Day (http://www.auntminnie.com), and the Society of Pediatric Radiology’s Case of the Week (http://www.pedrad.org).
Perhaps the main reason simulators have not been widely developed for diagnostic radiology is the lack of a perceived need. The impetus of most medical simulators is patient safety, particularly in relationship to resident and fellow training. In diagnostic radiology, the trainees are almost always supervised. This is particularly true for the junior-most residents where their case load is limited both in volume and complexity. For residents, every study is overread by a staff radiologist before the final report is released. During the overread process, trainees are instructed on findings that they missed or overinterpreted. At many institutions, the only time unsupervised preliminary image interpretations are released to the hospital is overnight. Radiology residents must have 1 year of radiology training before they are allowed to take unsupervised call. Even with this regulation, many hospitals have instituted 24-h faculty coverage, making unsupervised image interpretation almost nonexistent.
Given that unsupervised overnight time potentially represents the biggest risk to patient safety in diagnostic radiology, several simulators have been created to help prepare residents for this overnight call [20–28]. These simulators have ranged in complexity in their included cases, included modalities, file format of the digital image, and method of interpretation.
The first digital image simulators focused on specific indications or types of studies to teach one skill such as identifying intracranial hemorrhage on CT of the head or buckle fractures in pediatric patients (Fig. 38.7) [25, 26, 29]. With these simulators, the user was asked to answer one question, namely, is a specific abnormality present or is the study normal? In constructing the simulators, diagnostic images were converted from their native Digital Imaging and Communications in Medicine (DICOM) format to a JPEG formatted image via a screen capture. Digital hotspots were then added so that the user could click on any potential area of abnormality. Using a pretest and posttest, this type of simulator was shown to be effective in teaching trainees to make a specific finding [25, 26]. There have been no studies evaluating the user’s actual clinical performance before and after using this simulator.
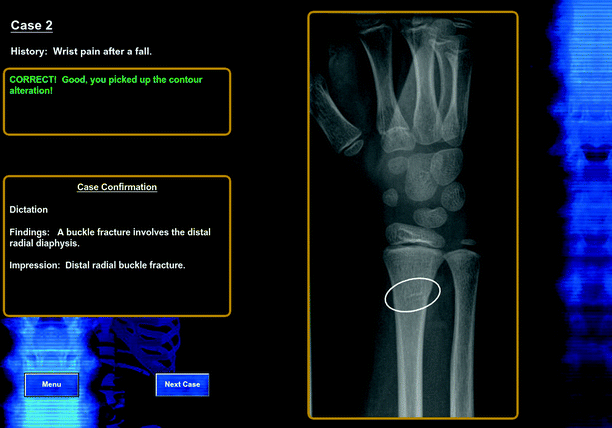
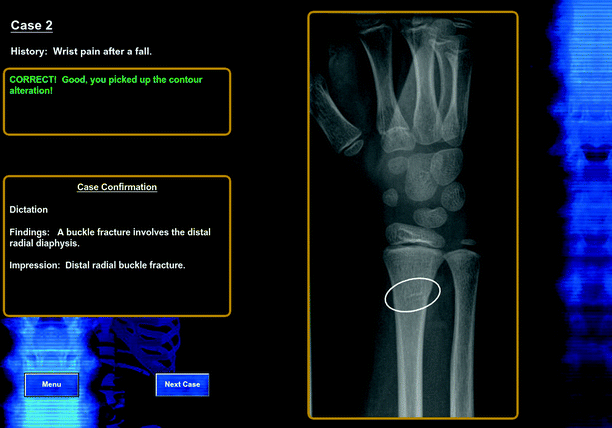
Fig. 38.7
Several indication-specific simulators have been developed. These simulators typically function in a web-based environment where the user clicks on a digital hotspot (oval) that contains the finding
There were several advantages to this type of focused simulator. First, the simulator was easy to use and required little training. Second, the user was able to see a large number of challenging cases in a small amount of time. Finally, because users received instantaneous feedback on what they missed, they learned as the simulation module progressed.
Even though these focused simulators were effective, there were several disadvantages that limited their adaptation. First, there are a limited number of diagnoses that have subtle imaging findings yet occur with a high-enough frequency to warrant inclusion into a simulator. Second, even though radiologists work in a digital environment, they are used to DICOM images. Modern picture archiving and communication systems (PACS) allow the user to magnify images, window/level, make measurements, and even provide sharpening algorithms to improve edge detection. Without these tools, the focused simulators are not truly able to mimic a radiologist’s work environment. Third, focused simulators often used one or several images, not the entire data set. The trainees knew that if an abnormality was present, it would be on the provided image. While this helps the trainee identify subtle findings on the image and can help with the search pattern, it does not mimic the current practice in radiology where a radiologist must scroll through a large volume of images to make a diagnosis. Finally, the creation of focused simulators was often seen to be too time intensive for the indications where they worked best. Most medical simulators are used to help teach clinicians how to manage rare or particularly dangerous indications. The indications that work best in focused radiology simulators are neither rare nor dangerous.
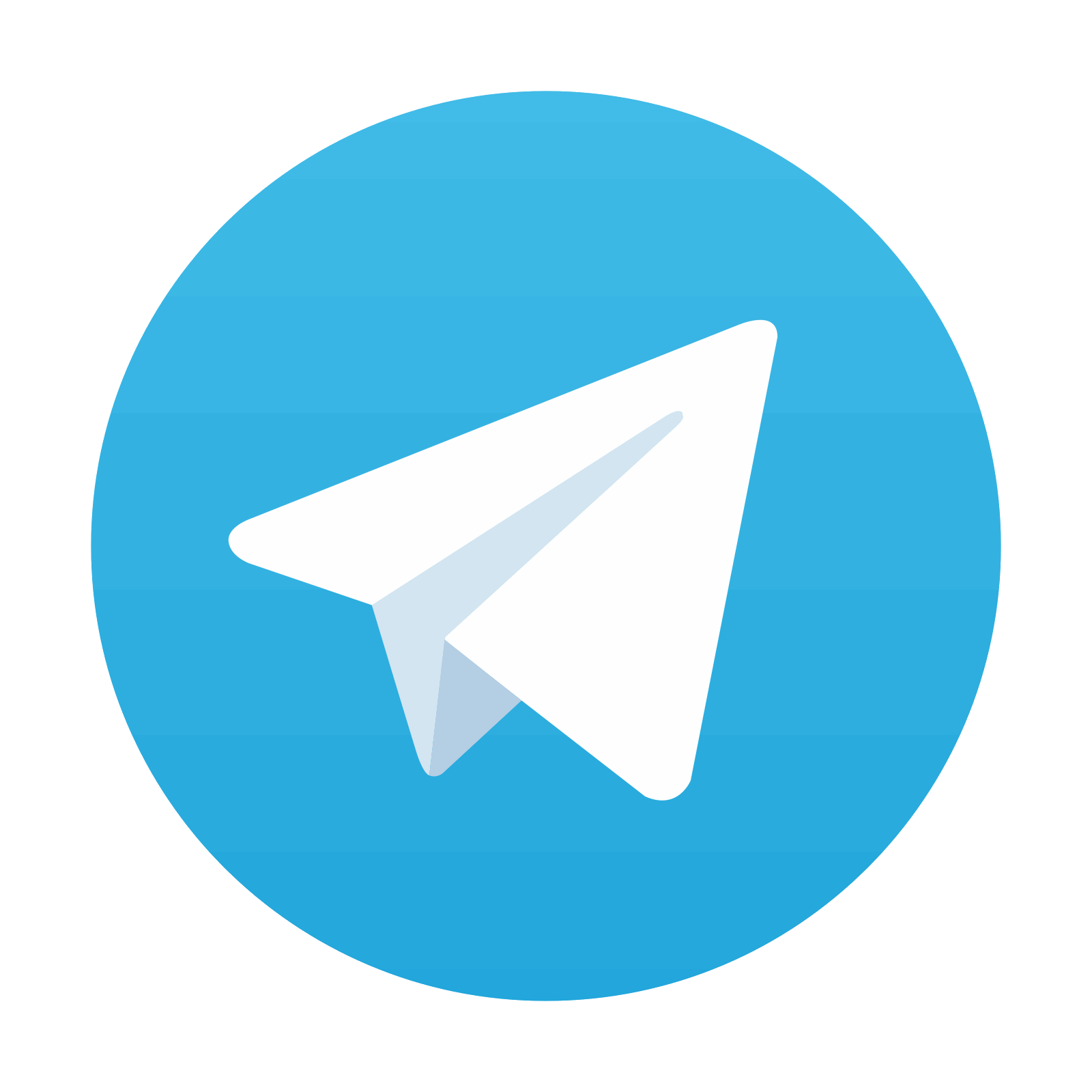
Stay updated, free articles. Join our Telegram channel

Full access? Get Clinical Tree
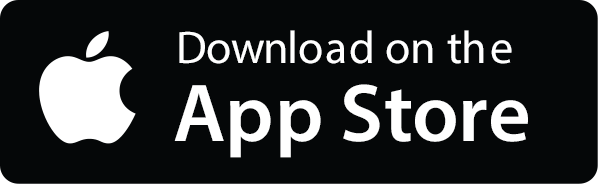
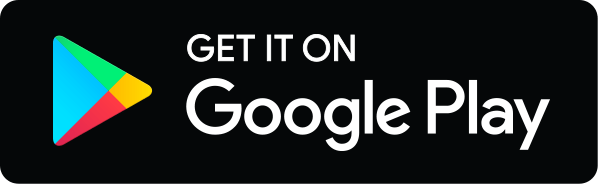