Fig. 32.1
Synthetic bone model in simulation lab
Fracture fixation is the most common application in cadaveric and synthetic bone skills training. Leong et al. [4] recruited 21 subjects to validate the use of three models of fracture fixation in the assessment of technical skills. The subjects were divided into three groups according to their experience in trauma procedures (novice, intermediate, and expert). Each subject was asked to perform three procedures: application of a dynamic compression plate on a cadaver porcine model, insertion of an un-reamed tibial intramedullary nail, and application of a forearm external fixator, the latter two on synthetic bone models. The primary outcome measures were the OSATS and GRS using video recordings of the procedures and motion analysis of the hand movements of the surgeons. Their results revealed significant differences among all three levels of expertise based on dynamic compression plate fixation of pre-fractured porcine tibia. External fixation of the pre-fractured synthetic ulna model did not differentiate among different expertise levels, and intramedullary nail insertion into a pre-fractured synthetic tibia model failed to differentiate between surgeons with intermediate and expert levels of expertise [4]. The authors noted: “This study has validated a low-cost, high-fidelity porcine dynamic compression plate model using video rating scores for skills assessment and movement analysis. It has also demonstrated that Synbone models for the application of an intramedullary nail and an external fixator are less sensitive and should be improved for further assessment of surgical skills in trauma” [4]. In the United States, the Orthopaedic Trauma Association (OTA – www.ota.org) and the AO Foundation a non profit organization of international surgeons specializing in the treatment of trauma and disorders of the musculoskeletal system lead the way for orthopedic trainees to gain experience at surgical simulation. During these courses, trainees become familiar with the various tools and implants utilized to repair common fractures. They learn reduction techniques as well as proper implant installation, drill technique, and screw placement.
Tuijthof and colleagues [16] developed the Practice Arthroscopic Surgical Skills for Perfect Operative Real-life Treatment (PASSPORT) training environment, a physical knee model that allows for realistic surgical actions. It includes standard portals, menisci that can be probed, and simulates problems such as bleeding and air bubbles. Medial and lateral springs enable application of varus and valgus stresses. Construct validity was assessed by testing 20 experienced arthroscopy surgeons (>50 knee arthroscopies per year) and 8 less-experienced residents in training (<20 arthroscopies per year). The experienced surgeons were significantly more efficient on PASSPORT than the residents, taking only 19.7 s on average to complete a specified set of tasks vs. the 55.2 s taken by the residents to complete the same tasks [16].
Howells et al. [17] using a laboratory-based shoulder simulator demonstrated that regular repetition of arthroscopic Bankhart repair was necessary to maintain optimum performance. Six fellowship-trained lower-extremity surgeons were trained to perform an arthroscopic Bankhart repair using the Alex Shoulder Professor simulator (Sawbones Europe, Malmö, Sweden) (Fig. 32.2). Having had no previous experience with Bankhart repair, these six surgeons demonstrated a learning curve as they improved significantly through their first 12 repetitions. The surgeons then returned to their respective lower-extremity practices and came back after 6 months to be tested again. The second set of results demonstrated that the surgeons had not retained any of their original improved technical skills; instead, they produced learning curves indistinguishable from the first set confirming loss of skill in performing Bankhart repair [17].
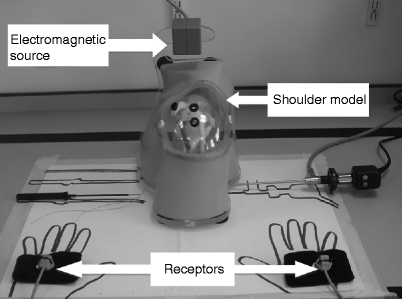
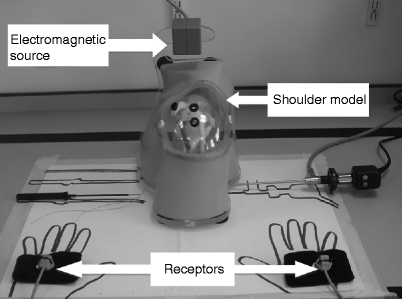
Fig. 32.2
The Alex Shoulder Professor benchtop simulator (Sawbones Europe, Malmö, Sweden) and the three-dimensional electromagnetic motion tracking system (PATRIOT; Polhemus, Colchester, Vermont) used to assess surgical performance (Howells et al. [17]. Used with permission from Journal of Bone and Joint Surgery)
Computer Tools
Orthopedic surgery training requires knowledge and understanding of the three-dimensional (3D) anatomy of the skeleton and its spatial relation to other anatomical structures, encouraging the development of computer-based simulators aimed at residency training. Software simulation programs assist the trainee in conceptualizing complex fracture anatomy and allow them to carry out a given surgical procedure in a virtual 3D environment (Figs. 32.3a and b) [18]. Citak et al. [19] created standard acetabular fracture models in synthetic pelves and performed CT scans that were either registered with 3D planning software or used for conventional 2D paper planning. After the planning, the fractured pelvis model was submerged in a gel medium to simulate soft tissue resistance and to allow the fracture position to be maintained when the reduction was complete. The accuracy of the reduction was found to be significantly better following planning with virtual 3D software compared to the standard technique (Figs. 32.3c, d and e). Similar software with the ability of 3D image production and manipulation based on patient-specific CT data is also available for joint resurfacing arthroplasty or total joint replacement procedures to improve accuracy and shorten learning curves [20, 21].
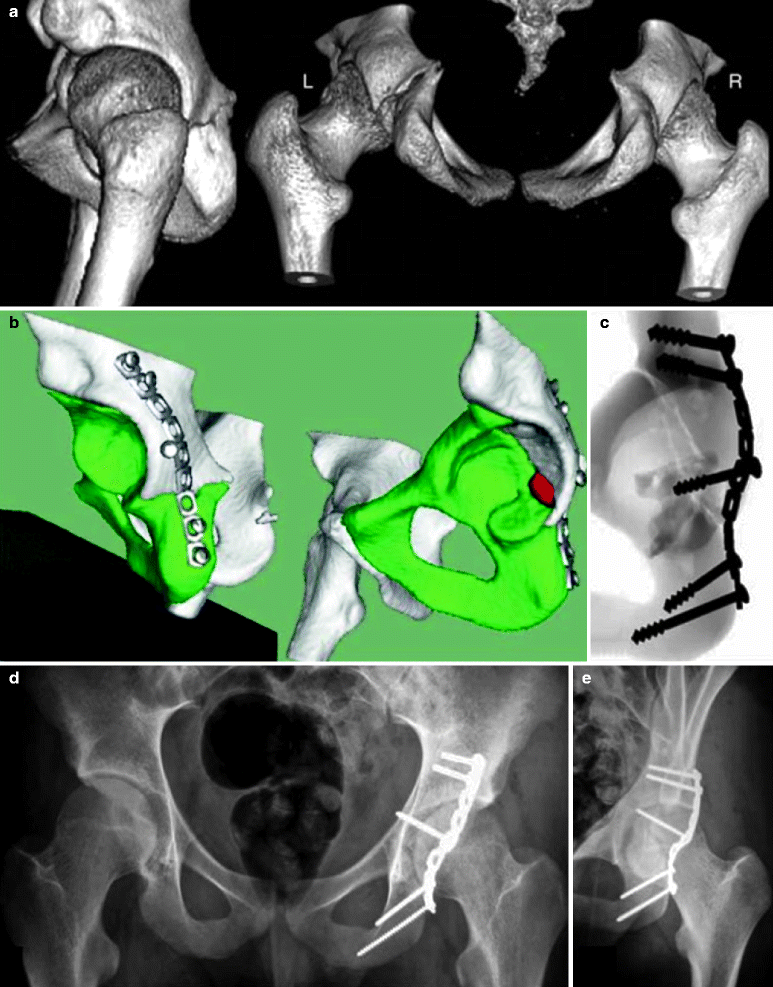
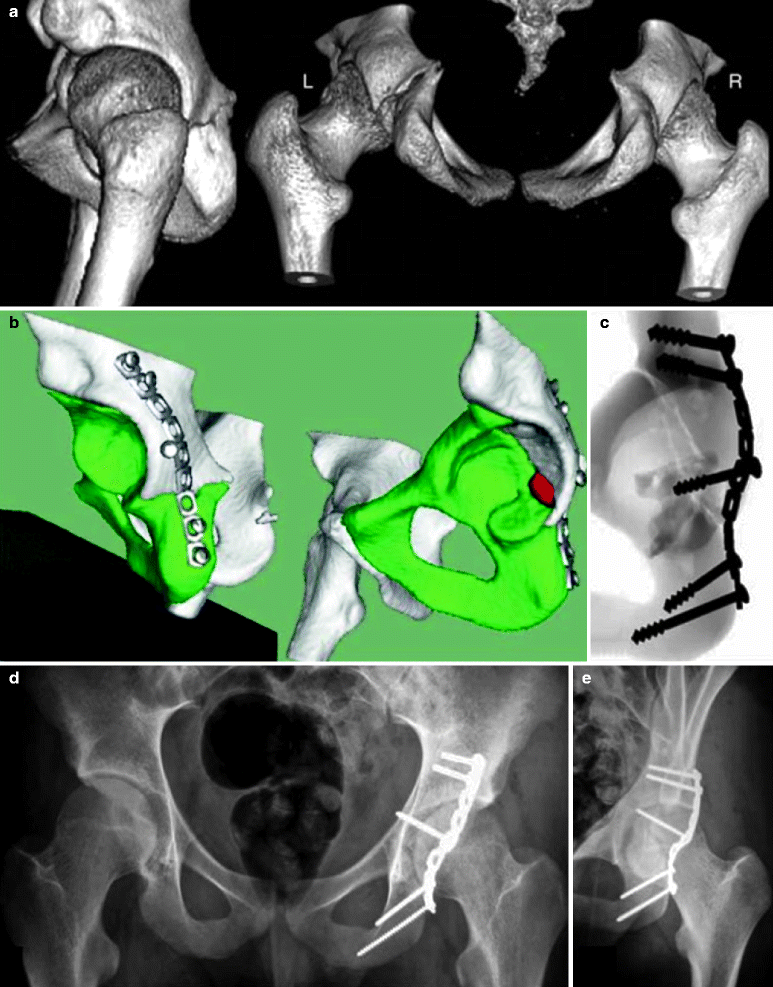
Fig. 32.3
(a) Lateral (left) and posteroanterior (right) three-dimensional (3D) computerized tomography (CT) reconstructions of a left acetabulum posterior wall fracture. (b) Virtual image of the fracture generated by the software based upon the preoperative CT images. The fracture fragments can be manipulated by the surgeon and reduced virtually after which the plate and the screws are applied. The fracture fragments are color-coded and the femur has been digitally subtracted for better visualization. (c) Rendering the bone translucent, as the surgeon would see it on fluoroscopy, created this virtual image. This enables better control of screw length and direction. (d, e) Actual postoperative radiographs of the pelvis and left hip. The final operative result is closely approximated by the preoperative virtual planning (© American Academy of Orthopaedic Surgeons. Reprinted from Atesok and Schemitsch [18], with permission)
Blyth et al. [22] studied a PC-based virtual reality training system designed to evaluate and improve a trainee’s ability to accurately reduce and internally fix a hip fracture, using standard anteroposterior and lateral radiographs. The simulator software, with a download size of 4 MB, could be accessed over the Internet or run on a stand-alone computer. The software incorporated all relevant tasks of pinning a hip fracture, from positioning of the C-arm and fracture reduction to skin incision and placement of the sliding hip screw, plate, and cortical screws (Fig. 32.4a–e). Ten participants were included in the study with each performing six operative scenarios on the simulator. Results indicated that the simulator had good “face validity,” providing a realistic view of the operating environment as well as testing problem-solving ability. The study was not comparative and conclusions were based on participants’ subjective feelings with regard to potential advantages that the software might provide [22].
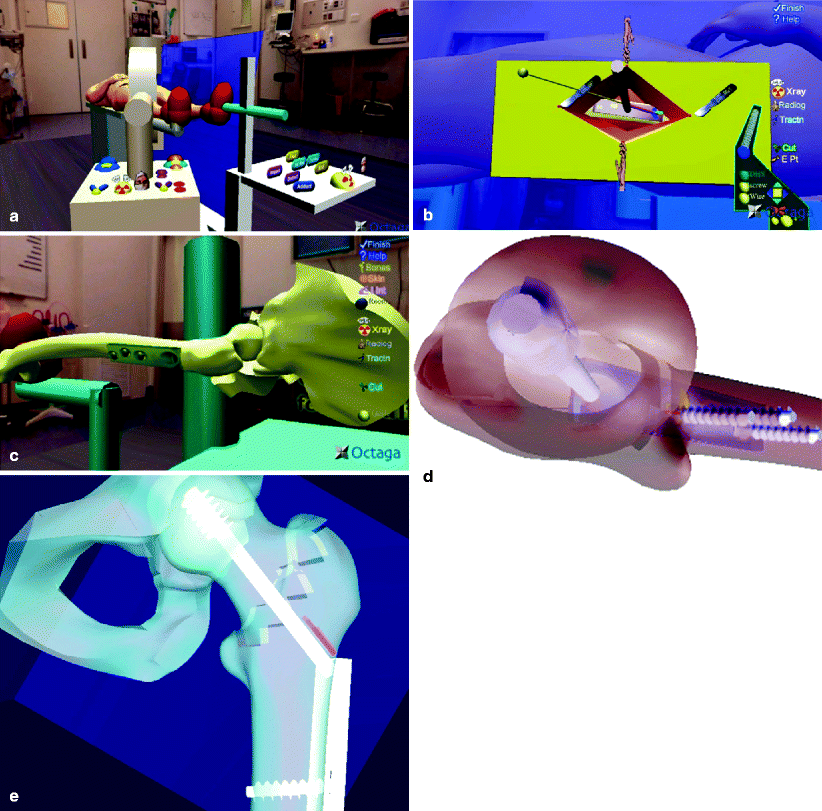
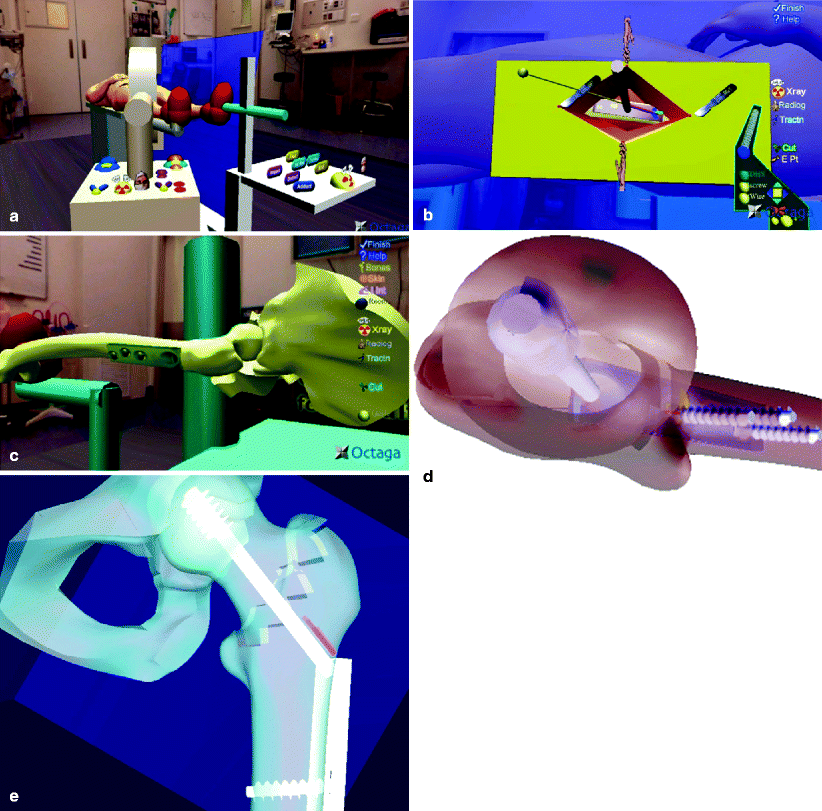
Fig. 32.4
Illustrations of a PC-based virtual reality training system for hip fracture fixation. (a) View of the virtual operating theater, showing the image intensifier and fracture table. (b) View of the virtual operative scene showing the skin incision, with the 135º guide plate visible on the femur. (c) Final reduction at the completion of the procedure with soft tissues removed. (d) Translucent femur demonstrating superiorly placed lag screw. (e) Virtual postoperative AP radiograph demonstrating fracture reduction and fixation placement (Reprinted from Blyth et al. [22], Copyright (2007), with permission from Elsevier)
Although available for several years, rapid prototyping, or 3D printing, has dropped significantly in price, making it accessible to surgeons who wish to practice an upcoming surgical procedure on realistic plastic models generated from the patient’s actual computerized tomography (CT) data [23].
Advances in computing processing and graphics cards now allow researchers to simulate complex injury mechanisms in real time. This is particularly useful in training in combat medicine. With body armor saving more and more lives, first responders and soldiers in the field are seeing a dramatic increase in extremity injuries including open fractures [24]. Reinig et al. [25] are developing a virtual environment for the United States Army to teach combat medics about thigh trauma. Using finite element modeling (FEM), the authors can predict and display how the femur and its surrounding soft tissues will respond to the application of various loads. The results are depicted as appropriate images on simulated radiographs, ultrasound, or computerized tomography. Thus, combat medics can practice ahead of time for the wide range of devastating injuries they will encounter in the field.
Haptics
A major complaint by trainees using early computer-based simulators has been the absence of physical feedback from the device. The introduction of haptics, or force feedback, to current orthopedic simulators gives the trainee a sense of shape and texture for the structure that they are working on. Haptics can produce either active feedback or passive feedback. In active haptic devices, devices such as the PHANTOM (Sensable Technologies, Inc., Woburn, MA) generate artificial mechanical resistance at the tip of the instrument being manipulated. However, some authors have noted that there is a lack of force applied to the shaft of the instrument being handled by the trainee [26]. This has led some researchers such as McCarthy et al. [26] to add passive haptic simulators that provide feedback along the shaft of a simulated arthroscopic instrument which adds to the realistic feel of the procedure. Vankipuram et al. [27] developed a simulation model which provides adequate haptic sensations for simulating drilling realistically. The authors suggested that simulated drilling with a virtual bone may improve trainees’ basic skills and make a positive impact on their drilling tasks in real operating conditions.
Virtual Reality Simulators for Arthroscopy Training
Knee arthroscopy has increased 49% between 1996 and 2006 with 984,607 arthroscopes being performed in 2006 alone [28]. One might argue that this increase in numbers bodes well for the trainee; however, the majority of these arthroscopies are performed in free-standing ambulatory surgery centers, and most of those are not associated with training programs. Thus, there is an increasing demand for arthroscopy in the United States, yet orthopedic residency programs are faced with the challenge of providing sufficient training while restricting work hours. One survey of fifth-year, US orthopedic residents revealed that only 32% believed that their program devoted adequate time for training in arthroscopy in stark contrast to the 66% of program directors [29].
The Knee Arthroscopy Surgical Trainer (KAST) developed by the American Academy of Orthopaedic Surgeons’ Task Force on Virtual Reality is designed to provide an intuitive and autonomous experience for the orthopedic resident [30]. KAST consists of a hardware component supported by proprietary software and a didactic component delivered on one of two monitors positioned in front of the trainee (Fig. 32.5a). The core of the hardware component is a pair of PHANTOM® haptic devices that recreate the feel of the arthroscope and the probe within the virtual knee (Fig. 32.5b). The workspace can be raised or lowered electronically to accommodate a wide range of statures, sitting or standing. To complete the feeling of performing a real arthroscopy, the trainee must manipulate a surrogate leg via flexion and extension of the knee and the application of varus and valgus forces. The simulator switches seamlessly between a right and a left knee, forcing the trainee to be ambidextrous with respect to the camera and probe.
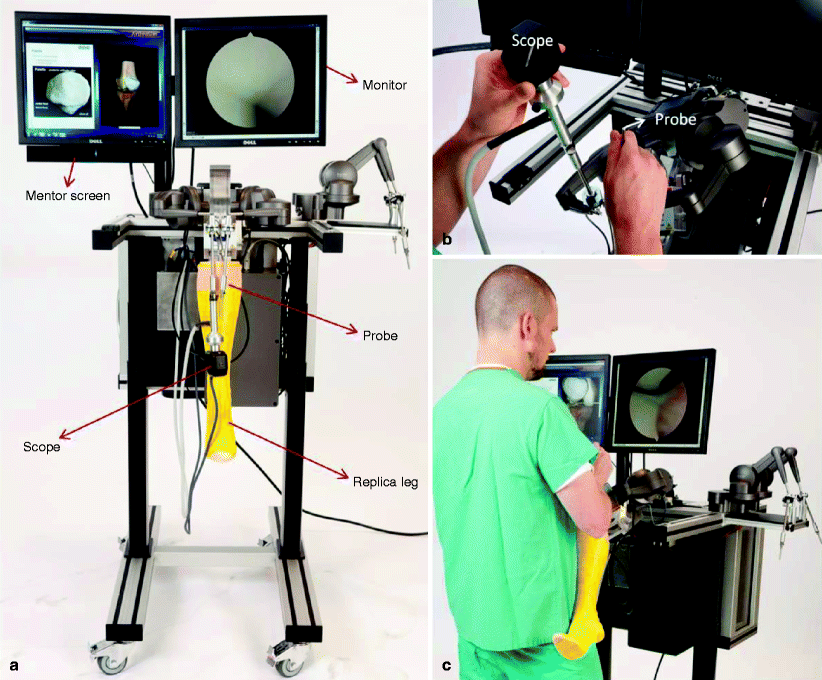
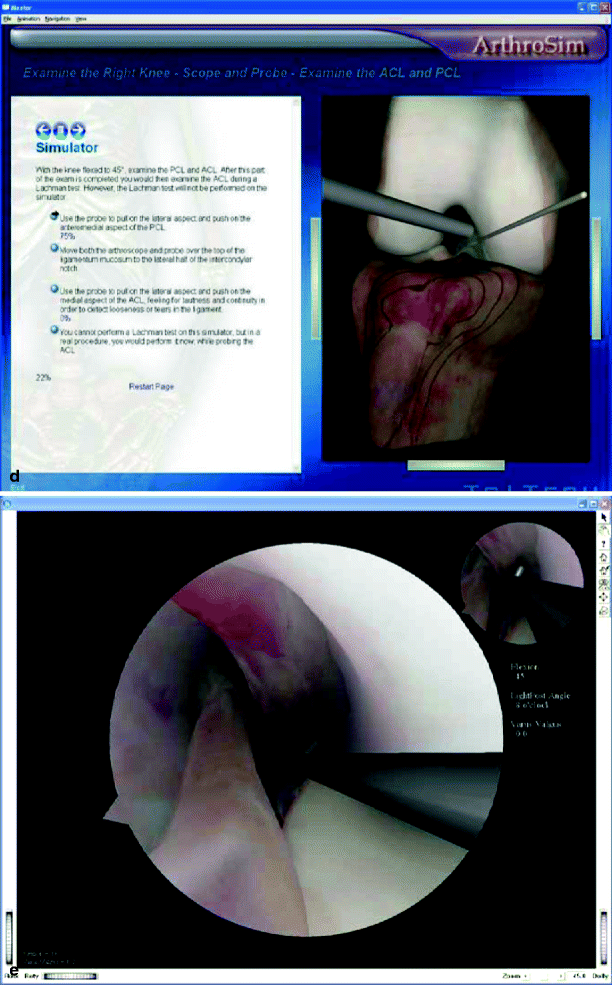
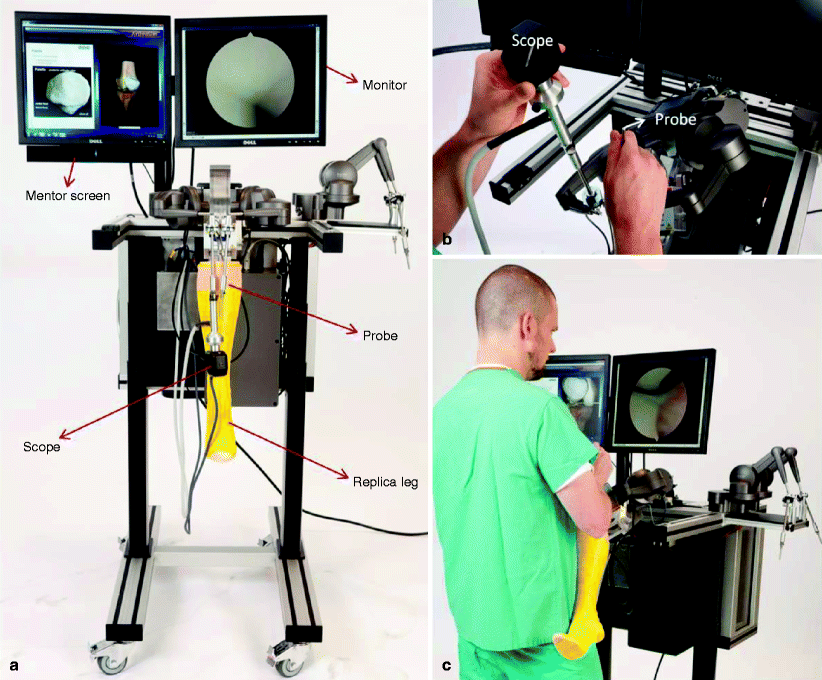
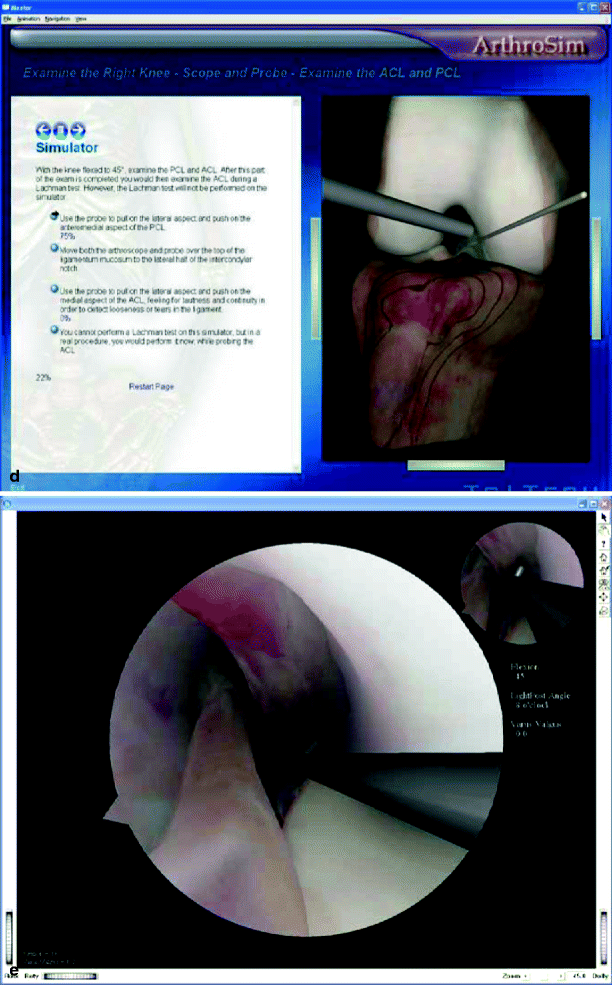
Fig. 32.5
(a) View of the Knee Arthroscopy Surgical Trainer (KAST). The Mentor screen (left) shows the instructions step by step with an animated view of each step. The arthroscopy monitor (right) displays the simulated procedure as in an actual arthroscopic procedure. (b) The scope and the probe are attached to separate PHANTOM haptic feedback devices. (c) The replica leg is used to apply varus and valgus stresses to the knee. Transducers built into the leg convey the information to the program, and the computer model responds appropriately. (d) The “Mentor” screen for KAST displays a set of instructions or tasks for the trainee on the left side and a “God’s-eye” view on the right to help orient the trainee during the initial phases of instruction. Later, during timed tasks, the “God’s-eye” view is not available. (e) The arthroscopy screen displays images as they would appear on a real monitor in the operating room
The arthroscopic images, displayed on the right-hand monitor, are generated by a program that recreates three-dimensional models of the internal structures of the knee. These images are based on data from the Visible Human Project [31] or similar but higher-resolution cryosectioned data (Fig. 32.5d, e). These structures interact with the virtual probe and arthroscope, just as the cartilage and synovial lining of a real knee would interact. Techniques developed by Touch of Life Technologies alter the morphology, posture, and pathologies displayed from the data, giving a limitless supply of virtual patients [30].
The didactic component of KAST, known as the “Mentor,” is displayed to the trainee on the left monitor (Fig. 32.5d). After logging on to KAST, the Mentor directs the trainee through a series of brief lessons demonstrating how to run the simulator. The Mentor program utilizes Hypertext Markup Language (HTML) to display text, audio, images, and video. In addition, the Mentor displays Interactive Anatomic Animations (IAA) utilizing texture-mapped polygonal models to render complex anatomic scenes that may be altered by the trainee’s actions. After that, the trainee is instructed on how to perform a complete diagnostic sweep of the knee with the arthroscope only, followed by a sweep with a probe in the opposite hand. The Mentor continuously records all activity generated by the trainee and then provides sophisticated feedback concerning the trainee’s progress.
Throughout the session, trainees must achieve proficiency while following the instructions from the Mentor program (Fig. 32.5d). The Mentor requires the trainee to score 100% on each step before proceeding to the next task. When they have successfully completed all required tasks, they do the entire procedure on their own and then one last time with the entire procedure timed. There is a “God’s-eye view” of the outside of the knee that shows where the arthroscope and the probe are with respect to the knee anatomy to assist the trainee with triangulation (Fig. 32.5a). This view is not available once the trainee advances to the timed tasks. KAST will continue to evolve through software updates to include meniscal resection and ACL repair. KAST has been designed so that the same hardware can be used for simulation of shoulder arthroscopy [30].
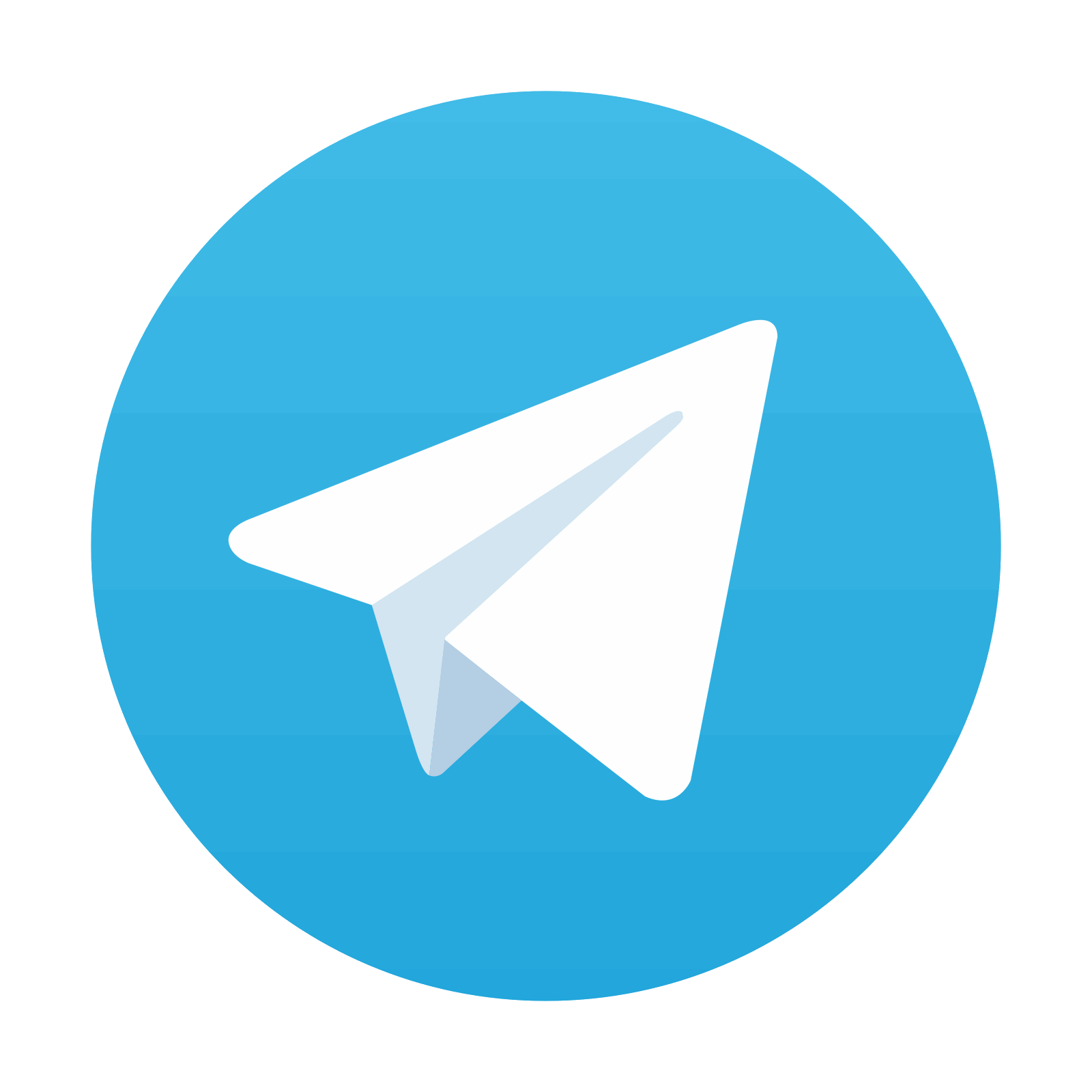
Stay updated, free articles. Join our Telegram channel

Full access? Get Clinical Tree
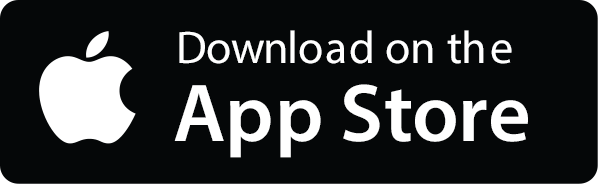
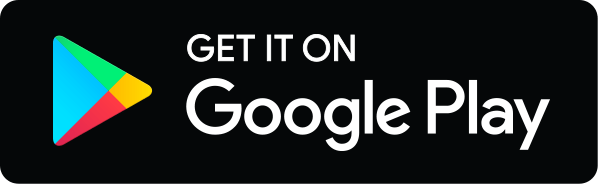