Fig. 28.1
The simulator interface allows neurosurgeons to explore a 3D MRI scan of a patient’s brain during presurgical planning. From the surgeon’s perspective, the interface is analogous to holding a miniature head in one hand which can be “sliced open” or “pointed to” using a cross-sectioning plane or a stylus tool, respectively, held in the other hand (Reprinted from Publication Hinckley et al. [17]. With permission from IOS Press)
New technologies have emerged from the “Props” interface and Netra that not only offer the ability to physically manipulate objects in space but also incorporate immersive VR systems. These new systems allow for computer-based head-tracking as well as the physical manipulation of objects and tools. Stoakley et al. [19] introduced a system called “Words in Miniature (WIM)” that offers a second dynamic viewport that allows for manipulation via head-tracking. Now, not only can the user operate on-screen images through physical manipulation of objects in space but he or she can also control images through simple head motions (Fig. 28.2).
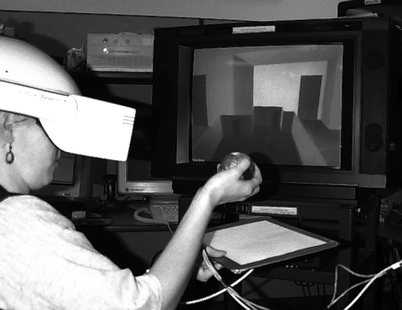
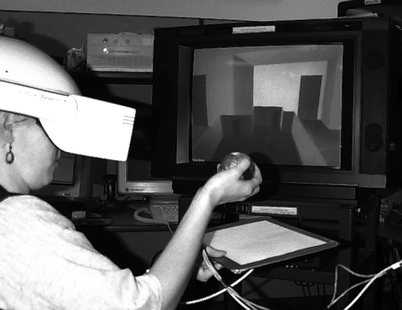
Fig. 28.2
Immersive VR system. The WIM graphics attached to the clipboard are a miniature copy of all the surrounding graphics in the immersive environment. Each of the objects in the WIM copy is tied to their counterparts in the immersive environment through pointers and vice versa at the point of WIM creation. The user can manipulate the objects in the WIM, and the objects in the world will follow (Reprinted from Stoakley et al. [19]. With permission ACM Press/Addison-Wesley Publishing Co.)
Stereotactic surgery is one area of neurosurgery in which preoperative planning is critical for a successful outcome. The ability to precisely calculate and localize surgical coordinates is paramount to successful stereotactic, frame-based functional neurosurgery. The first product introduced for this purpose was StereoPlan by Radionics (now Integra LifeSciences). Integra has numerous commercially available products [20] to aid functional neurosurgeons in their preoperative planning. These systems not only provide precise coordinate information but also have the ability to incorporate multiple image sets together, provide multiple measurement lines, and afford the surgeon the ability to track the entire trajectory through the anatomy that will be encountered during surgery. Therefore, a more complete analysis of patient data is possible, and multiple surgical approaches can be rehearsed and considered.
Adams and Wilson [21] developed a 3D simulation model of the cerebral ventricular system using commercially available computer software. This model was developed for the purposes of neuroanatomical education; however, it can also be applied for preoperative planning purposes. The team’s main concern was that most anatomical education is based on either 2D images or on artist renderings of what the “ideal” brain should look like. Consequently, they developed a virtual anatomical model based on postmortem MRI analysis to develop an anatomically accurate ventricular and cerebral model. This model can be projected stereoscopically to allow the user a more realistic setting in which they view and manipulate the structures. In addition to the anatomical structures, Adams et al. modeled the flow of CSF through the ventricular system. These two abilities not only allow for proper education on relevant anatomy but can be used for successful preoperative planning. Using accurate models gives the surgeon the ability to create 3D models of abnormal anatomy such as shifted or slit ventricles and abnormal CSF flow as seen in noncommunicating hydrocephalus. This system provides a very good preoperative planning model for diseases involving the ventricular system.
Additionally, two other high-fidelity VR simulators are available that greatly improve the ability of the surgeon to plan preoperatively. The Virtual Workbench [22] and the Dextroscope [23] create a very fluid, 3D representation of patient data that is easily manipulated, allowing the surgeon to visualize patient anatomy in any orientation before operating. Although these systems are modeled on the binocular microscope, they remain “hands-on” in a way that microscopes cannot. Because of the binocular glass platform, users control the system with their hands and 3D images co-localized in the same position in real time. Consequently, the VR simulation mirrors that of a true OR situation. These systems are designed to incorporate and fuse together multiple patient image sets (magnetic resonance imaging, magnetic resonance angiography and venography, and computed tomography) so that a 3D representation of patient data is created. As a result, the user has the ability to manipulate this 3D model in real time and visualize the exact anatomy that will be encountered during the surgical procedure (Fig. 28.3). Without these VR systems, users are forced to plan procedures using standard techniques of looking at patient images in a two-dimensional, slice-by-slice fashion. Both the Virtual Workbench and the Dextroscope eliminate this problem by integrating all relevant data, ultimately presenting the surgeon with as complete a picture as possible. Users of this technology believe that this is helpful in the surgical planning, which is an abstract process that relies mainly on displaying and viewing certain key structures, combined with an ability to interact with those structures quickly and intuitively. Yet there is no data that rehearsing of the actual surgery was beneficial in decreasing the complication rates or shortening the surgical procedure. At this point this surgical planning software is still experimental and is mostly used in group conferences and teaching courses [24–26].
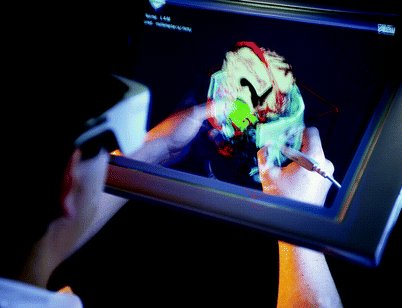
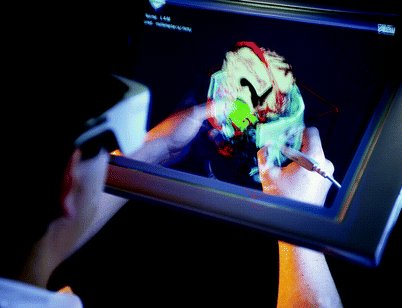
Fig. 28.3
Working with the Dextroscope, the user’s hands are collocated with the virtual stereoscopic objects displayed as a reflection behind a mirror (Reprinted from Kockro et al. [24]. With kind permission from Wolters Kluwer Health)
The potential advantages of these products are that they afford surgeons the ability to see all potential complications of surgery before actually operating. Users have the ability to plan the best surgical approach for each patient without facing concerns about the problems that may arise during surgery. As a result, intraoperative mistakes can be minimized, operating time can be reduced, and the overall safety of the patient can be ensured. Because this field is in its infancy, there is no data to support this potential benefit.
Stereolithographic Modeling
In addition to all of the VR simulation systems developed for preoperative planning, a more simplistic approach can also be utilized: stereolithographic modeling (Fig. 28.4) [1, 2]. This procedure has been used to model cerebral vasculature for the study of aneurysms and its application in microsurgical clipping. Physical modeling, though not as comprehensive as VR simulation, provides a few unique benefits for preoperative planning that VR simulation does not. First, it provides a physical model of patient data that surgeons can use for multiple purposes, one of which is to explain the complexities and intricacies of the procedure to the patient and their families. Because neurosurgical procedures tend to be very complex, it is difficult for the surgeon to explain the intricacies of the operation to patients and families. However, by having a physical model that a surgeon can manipulate, patient and family education becomes much easier, and any confusion the patient may have is inevitably reduced. Additionally, this type of modeling allows the surgeon to practice aneurysm clipping and aides in the process of choosing what surgical clip or clips will be appropriate for successful treatment.
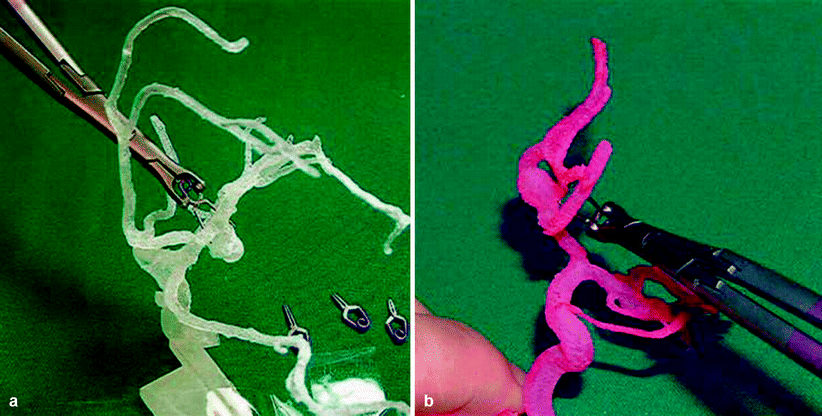
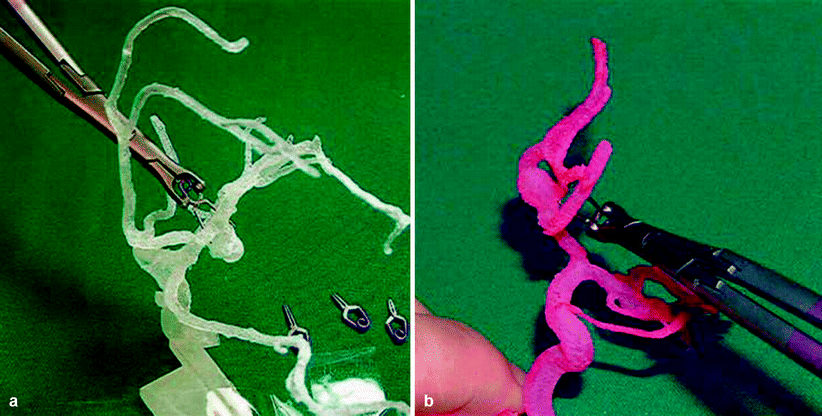
Fig. 28.4
Photographs of an MCA aneurysm clipping model. (a, b) different MCA aneurysm models detailed view of the aneurysm with the parent and branching vessels (Reprinted from Wurm et al. [1]. With kind permission from JNS publishing group)
Despite the benefits of stereolithography, many disadvantages remain in using this technique: primarily the inflexibility of the material used to create the model. Researchers have yet to find a model that realistically resembles in vivo vasculature – most of the material currently in use is frequently too rigid to approximate real human tissue. Consequently, preoperative planning is difficult because the surgeon does not have a completely realistic model on which to practice technique. Additionally, the process of making these models is both costly and time consuming. As the manufacturing process takes 3–7 days [2], it would take too long to create patient-specific models even if the equipment was on site. Additionally, since cerebral aneurysms are very unpredictable and ruptured aneurysms require emergency surgery, there is not enough time to create a planning model prior to surgery. While these models are beneficial for select purposes, mainly patient education, their overall usefulness is questionable until new models can be developed that both approximate human tissue more appropriately and take significantly less time to manufacture. Because there are no virtual reality aneurysm clipping modules available at this point, there are no comparative results to evaluate these two techniques.
Reality Training Applications
As stated above, preoperative planning is, without question, a fundamental part of any operation. However, when the time arrives for surgical execution, no amount of planning can compensate for an untrained, inexperienced surgeon. Various restrictions on work hours and clinical obligations can limit residents’ training time and make new techniques necessary to help teach residents and allow established surgeons to practice and hone their skills. Simulation provides surgeons with a new immersive VR world that not only simulates patient data in 3D but provides tactile feedback to the system user. This affords the surgeons the ability to manipulate patient data as well as “perform” the operation using the immersive VR simulator. Therefore, the user feels as if he or she is actually performing the operation because the VR simulator provides both visual and haptic sensory feedback. The main requirements of a good VR simulator are that the user not only has a realistic sense of the visual world in which they will be working but also has appropriate tactile feedback that simulates a real operation. To date, many systems (Table 28.1) are available that provide a good approximation of real neurosurgery via VR simulation.
Table 28.1
A list of the virtual environments discussed in this chapter
Author | Year | VR | Surgical modules |
---|---|---|---|
Burtscher | 1999 | 3D virtual images | Virtual endoscopy |
Luciano | 2005 | Tactile haptics | Ventriculostomy |
Chui | 2006 | Tactile haptics | Vertebroplasty |
Tsang | 2008 | Tactile haptics | Endovascular surgery |
Schulze | 2008 | 2D visual | Endoscopy |
Banerjee | 2011 | Tactile haptics | Thoracic pedicle screw placement |
Delorme | 2012 | Tactile haptics | Craniotomy and tumor debulking/cauterization |
Ventriculostomy
In 2006, Luciano et al. [3] developed what is perhaps the most widely used VR simulator for ventriculostomy: ImmersiveTouch. It is an augmented VR system and is the first of its kind to integrate a haptic device with a high-resolution stereoscopic display. The haptic device includes both head- and hand-tracking capabilities. Therefore, the user can control the on-screen images by either manipulating the hand controllers or by moving their head in space. This approximates real OR situations where the surgeon has the ability to do both of these maneuvers: manipulate surgical instruments and position his or her body and head in space to best visualize the surgical field. The haptic system was developed to provide multiple sensory modalities including visual, aural, tactile, and kinesthetic. Additionally, the system was developed so that different tissue types (e.g., CSF, parenchyma, ependymal lining) provide different haptic feedback responses. As a result, the user receives feedback similar to that in real surgery (Figs. 28.5 and 28.6).
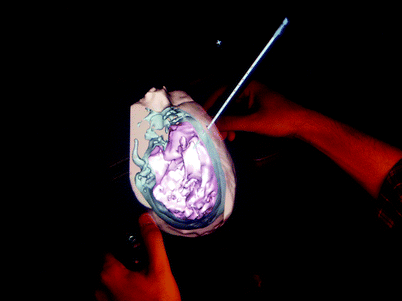
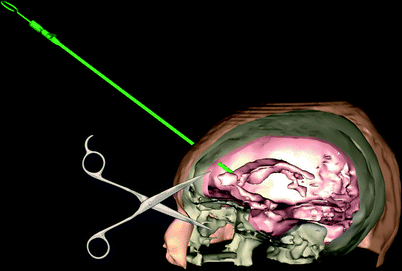
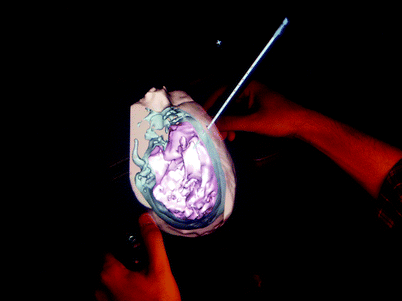
Fig. 28.5
A ventriculostomy simulation. The trainee is inserting the ventricular catheter according to skull landmarks. A virtual cranial window is shown to check the location of the tip of the ventricular catheter (Image courtesy of ImmersiveTouch)
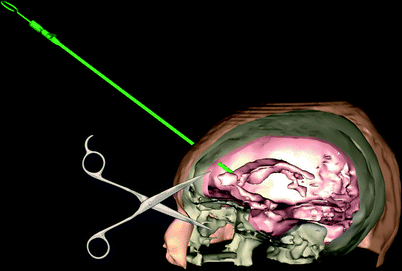
Fig. 28.6
Checking the location of the ventricular catheter at the end of the procedure. The catheter color changes green if the tip is within the ventricular system (Image courtesy of ImmersiveTouch)
In theory, ImmersiveTouch could be used for many different types of surgical interventions, but the device was validated for a simple ventriculostomy procedure that is often learned during the first year of residency. Luciano et al. [3] used patient data to develop a VR module based upon normal anatomy and had neurosurgery faculty, residents, and medical students test the system. All of the users found the platform to have realistic visual, tactile, and handling characteristics. Additionally, it was found to be a viable alternative to standard training. Lemole et al. [13] sought to prove that the system could be used with abnormal anatomy as well. After several attempts, ImmersiveTouch users were able to properly cannulate the abnormal virtual ventricle demonstrating proof of concept in a different spectrum of pathological scenarios.
Additionally, ImmersiveTouch was tested and validated by neurosurgery residents on numerous occasions [13, 14, 27], all of which validated the use of VR simulators. At the 2006 annual meeting of the American Association of Neurological Surgeons (AANS), 78 neurosurgical fellows and residents were tested on ImmersiveTouch during the 3-day-long Top Gun competition. ImmersiveTouch was used to demonstrate VR simulation of ventriculostomy. Banerjee et al. [14] showed that the ImmersiveTouch system allowed for accurate catheter placement that is comparable to retrospective evaluation of freehand ventriculostomy catheter placement as measured by mean distance of catheter tip from the foramen of Monro.
Furthermore, VR simulators have been used for training of catheter placement in abnormal ventricle anatomy [27]. Yudkowsky et al. [27] demonstrated, via the use of multiple image libraries, that ImmersiveTouch was a beneficial system for ventriculostomy training. They tested neurosurgery residents’ ability to successfully place a ventricular catheter into normal, shifted, and slit ventricles using image libraries derived from patient data. Residents were allowed to practice on the ImmersiveTouch system with each type of ventricle before being tested with novel image libraries. Yudkowsky et al. [27] demonstrate that not only did practice on the ImmersiveTouch improve the residents’ ability to successfully place catheters on the simulation post-intervention but also that residents’ live-procedure outcomes showed improvement in the rate of successful cannulation on first pass. Residents felt that ImmersiveTouch provided appropriate tactile feedback and that it was a realistic alternative to actual ventriculostomy procedures.
Spinal Applications
One area of neurosurgery in which VR simulation would greatly benefit is spinal procedures. These include, but are not limited to, vertebroplasty [28], pedicle screw placement during fusion surgery [29], and spinal cord stimulation [30]. Properly executed vertebroplasty requires the surgeon to rely on both sight and touch. The critical step in this procedure is the injection of polymethylmethacrylate (PMMA) into the vertebra. Incorrect injection or reflux of PMMA can have very harmful clinical consequences for the patient. Therefore, if a surgeon trains for this procedure using a VR simulator, surgical errors can be minimized. Chui et al. [28] developed the Immersion CyberGrasp – a VR system designed to simulate PMMA injections during surgery. Utilizing patient CT images and a specially designed glove, surgeons can simulate the injection technique with appropriate haptic feedback. The glove is designed to provide realistic variable resistance felt during needle injection. All these elements together provide a realistic VR simulation.
Discussed above for its applications in ventriculostomy, Luciano et al. [29] recently described the use of their ImmersiveTouch system for thoracic pedicle screw placement. The system allows the user to continuously monitor drill projection via anterior/posterior, transverse, and lateral fluoroscopic views (Figs. 28.7 and 28.8). For more advanced training, the user can also turn the different views off. Additionally, ImmersiveTouch provides haptic feedback and vibration feedback to represent the natural vibration of the drill with corresponding changes in vibration feedback depending on the speed of the drill. Furthermore, like the Top Gun competition held at the 2006 AANS annual meeting, another competition was held at the 2009 AANS annual meeting in which thoracic pedicle screw placement was one of the tasks that residents and fellows had to perform. Luciano et al. [29] described the results from the ImmersiveTouch system and showed that accuracy of thoracic pedicle screw placement using the ImmersiveTouch system correlated with actual placement via a retrospective evaluation of OR screw placements.
