The modern era of antibiotics starts with the discovery of penicillin by Alexander Fleming in 1928. Since then, antibiotics have transformed the face of modern medicine and enabled major advances in the treatment of patients. So much so that antibiotics could partially be credited for the staggering increase in the general population’s life expectancy from around 60 years old in the UK in the 1920s, according to the Office for National Statistics, to 81 years old in 2015.
Critical care is a young specialty whose evolution has largely been shaped by the evolution of antimicrobial therapy. It can be argued that the only treatment we can offer our patients is antimicrobials, the rest – vasoactive medications, mechanical ventilation, diuretics and so on – are merely supportive measures.
The aim of this chapter is to present the aspects of antimicrobial therapy most pertaining to a cardiothoracic critical care context. Data are presented as valid in 2016. We do not assume to give an exhaustive representation of the myriad of issues faced by the modern intensivist, but to offer a brief update of the most important issues we are currently confronting.
‘Appropriate’ Antibiotic Therapy
There is no universal recipe for which antibiotics should be used first in a critically ill patient. As shown in Figure 12.1, the choice is individualised for the patient, unit, hospital and geographical area. Most clinicians, when faced with a patient with a severe infection or septic shock, would choose to start with a broad spectrum antibiotic or combination and narrow down as pathogens and their susceptibilities are identified.
The comparative effects of appropriate and inappropriate antibiotic therapy are well illustrated in a recent retrospective study by Neinaber et al. conducted over 6 years in a single institution in the USA. In this study, the risk of nosocomial infections, the median length of stay and the hospital mortality were significantly higher in the inappropriate antibiotic group.
Figure 12.1 Flow chart for choice of antibiotic.
An example of an algorithm for presumptive antibiotic therapy for pneumonia in a critically ill patient is presented in Figure 12.2.
Figure 12.2 Presumptive antibiotic therapy for pneumonia in the ICU. HCAP Health Care Associated Pneumonia; HAP hospital acquired pneumonia.
Critically ill patients have multiple risk factors for serious and life-threatening infections. Exposure to antibiotics and other treatments which eradicate and modify the commensal flora, compromised host defences due to nutritional deficiencies, critical illness, compromised integument (intravascular devices, percutaneous interventions and devices) and disruption of cellular and humoral immunity as in the transplant patients lead to a high susceptibility to sepsis. In the context of severe infections, Table 12.1 highlights the most prevalent microorganisms in Western world critical care patients.
Table 12.1 Most prevalent organisms in Western world critical care patients
Diagnosis | Potential pathogens | Initial therapy | Alternative therapy |
---|---|---|---|
Catheter-related blood stream infections |
|
|
|
Infectious endocarditis |
|
|
|
Pneumonia, including VAP | Gram-negative rods, Haemophilus, S. pneumoniae | Piperacillin-tazobactam |
|
Sepsis/bacteraemia |
|
|
|
Antibiotic Administration in the Critically Ill Patient
In the last few years there has been a focus on the most appropriate way of administering and dosing antibiotics in intensive care. This has been triggered by a better understanding of the critical illness pathophysiology, as well as the emergence of new technologies that alter the pharmacodynamics and pharmacokinetics of drugs in this patient population (Figure 12.3).
Figure 12.3 The kill characteristics of antibiotics. Modified from Tsai et al. (2015).
Time dependent antimicrobials (fT>MIC) include beta-lactams, glycopeptides, macrolides and linezolid. Their concentrations have to be well above the minimum inhibitory concentration (MIC) for the pathogen for at least 40% of the interval between doses in order to achieve their therapeutic targets. There is a growing body of research concerning the administration of beta-lactams as a continuous or prolonged infusion so that the optimum concentration is achieved for sustained periods of time.
Concentration dependent antimicrobials (fCmax/MIC) include aminoglycosides, fluoroquinolones, metronidazole, echinocandins, polyenes and daptomycin. These antibiotics need to be administered in doses which achieve target concentrations well above 8–9 times MIC of the pathogen.
The antibiotic concentrations in the target tissues are influenced by the pharmacokinetic changes in the critically ill patient.
1. Volume of distribution, Vd – increased for hydrophilic drugs due to increased total body water; unchanged for lipophilic drugs.
2. Clearance and elimination – may change according to the renal and hepatic blood flows and function; severely deranged in septic shock; drug clearance and elimination also dependent on whether renal replacement therapy is instituted.
3. Decreased albumin levels – the volume of distribution and clearance of protein-bound drugs increase, decreasing their efficacy.
4. End-organ dysfunction – cardiac dysfunction in the context of septic shock increases the elimination half-time and the risk of toxicity and drug accumulation.
5. Tissue penetration – can be variable due to microcirculatory dysfunction.
Special Case – Antibiotics and Extracorporeal Circuits
The pharmacokinetics of antibiotics in patients on extracorporeal circuits is a growing area of research. The volume and distribution and clearance of drugs is altered and these are compounded by the added potential for sequestration in the circuit. The importance of understanding the pharmacology and changing the doses and intervals of administration of antimicrobials is colossal, since subtherapeutic doses are associated with worse outcomes.
An example is provided in Figure 12.4, which shows the effect of two dosing regimens of meropenem, as described by Shekar et al. (2013).
Figure 12.4 Meropenem concentration during ECMO run with two different dosing regimens.
Unfortunately, data are very limited to be able to generalise these results for all antimicrobials. What is certain, though, so far, is that ‘one size does not fit all’ when it comes to drug dosing in patients on extracorporeal circuits. If feasible, drug levels should be monitored in this category of patients in order to establish the appropriate therapeutic regime.
Endocarditis and Intracardiac Device Infection
Infective endocarditis (IE) is a deadly disease. Despite major improvements in diagnosis and treatment, IE remains associated with high morbidity and mortality. IE patients admitted to critical care are among the sickest the intensivist will have to deal with.
The following categories of patients have the highest risk to acquire IE, as well as a poorer prognosis of the disease:
1. Patients with a prosthetic valve or with prosthetic material used for valve repair; this category includes transcatheter implanted valves and homografts.
2. Patients with previous IE; they also have a higher risk of infectious complications than patients with a first episode of IE.
3. Patients with untreated cyanotic congenital heart disease (CHD) or with prosthetic baffles or conduits as part of palliative management; the European Society of Cardiology (ESC) guidelines 2015 recommend that patients with definitive repair of CHD with prosthetic material should only receive antibiotic prophylaxis for the first 6 months, while endothelialisation of the prosthetic material occurs.
Diagnosis of infective endocarditis is based on the modified Duke criteria and the ESC 2015 guidelines, as shown in Table 12.2.
Table 12.2 Diagnosis of infective endocarditis
Definite IE | |
---|---|
Pathological criteria |
|
Clinical criteria |
|
Possible IE | |
| |
Unlikely/rejected IE | |
| |
Major and minor criteria of IE (ESC, 2015) | |
Major criteria | |
| |
| |
Minor criteria | |
|
Successful treatment of IE involves primarily pathogen eradication. Surgical treatment and the treatment of complications are beyond the scope of this chapter. With respect to antimicrobials in IE, there are currently the following recommendations, according to the British Society of Antimicrobial Chemotherapy, 2012 guidelines.
1. Initial empirical treatment of native valve endocarditis (NVE) depends on the clinical presentation.
(i) NVE indolent presentation: amoxicillin + gentamicin.
(ii) NVE severe sepsis: vancomycin + gentamicin.
(iii) NVE severe sepsis + risk of MDR: vancomycin + meropenem.
2. Prosthetic valve endocarditis (PVE): vancomycin + gentamicin + rifampicin; this applies to all PVE irrespective of time.
3. Antibiotic treatment of IE due to oral streptococci and Streptococcus bovis group depends on minimum inhibitory concentration, MIC (usual course is 4 weeks for NVE and 6 weeks for PVE).
(i) Penicillin-susceptible strains, non-penicillin allergic patients:
(a) Benzylpenicillin, or
(b) Amoxicillin, or
(c) Ceftriaxone.
(ii) Penicillin-susceptible strains, penicillin-allergic patients:
Vancomycin BD.
(iii) Strains relatively resistant to penicillin (higher MIC required), non-penicillin allergic patients:
(a) Benzylpenicillin, double the dose for penicillin-susceptible strains and gentamicin, or
(b) Amoxicillin, double the dose for penicillin-susceptible strains and gentamicin, or
(c) Ceftriaxone and gentamicin.
(iv) Strains relatively resistant to penicillin (higher MIC required), penicillin- allergic patients:
Vancomycin and gentamicin.
4. Antibiotic treatment of IE due to Staphylococcus spp., for NVE:
(i) Methicillin-susceptible Staphylococcus spp., non-penicillin-allergic patients:
Flucloxacillin.
(ii) Methicillin-susceptible Staphylococcus aureus, alternative strategy:
Vancomycin or daptomycin.
(iii) Penicillin-allergic patients or methicillin-resistant Staphylococcus spp.:
(a) Vancomycin for 4–6 weeks, intravenous administration,
(b) Daptomycin for 4–6 weeks, intravenous administration.
5. Antibiotic treatment of IE due to Enterococcus spp.:
(i) Beta-lactam and gentamicin-susceptible strains:
(a) Amoxicillin and gentamicin for 4 weeks for NVE or 6 weeks for PVE,
(b) Ampicillin with ceftriaxone for 4 weeks for NVE or 6 weeks for PVE, for E. faecalis,
(c) Vancomycin and gentamicin for 6 weeks if penicillin allergy known or suspected.
(ii) Gentamicin-resistant species – replace gentamicin with streptomycin, if susceptible to the latter.
(iii) Multiresistance to beta-lactams, aminoglycosides and vancomycin:
(a) Daptomycin and ampicillin,
(b) Linezolid,
(c) Vancomycin, if beta-lactam resistance but vancomycin susceptibility detected.
We must stress that these are only guidelines, and that individual hospitals in different regions may have different antibiotic policies, depending on the local ecology.
Catheter Related Bloodstream Infections
The definition of catheter related bloodstream infections, also known as central line associated bloodstream infections (CLABSI), is bacteraemia or fungaemia in a patient with an intravascular catheter, sepsis and at least one positive blood culture taken from a peripheral vein, in the absence of another apparent source except for the catheter. In addition, there should be evidence that the catheter was infected based on quantitative or semi-quantitative methods. Another diagnostic method for CLABSI is differential time to positivity – blood cultures taken from the central line presumed to be infected may turn positive at least 2 hours before blood cultures taken simultaneously from a peripheral vein. This is due to the almost three-fold increase in the microorganism load of an infected catheter compared to blood.
Prevention of CLABSI relies on several measures, as follows:
1. Choice of insertion site – femoral lines should be a last resort; they should be changed as soon as possible, within a maximum time frame of 48 hours.
2. Insertion techniques – experienced personnel, avoidance of haematoma formation around the insertion site.
3. Adoption of a care bundle; the Matching Michigan campaign has shown that CLABSI decreased by more than 60% over 2 years in ICUs in England after introduction of a care bundle.
4. Antibiotic-coated central venous catheters have been proposed and adopted with success across intensive care units worldwide. The catheters are impregnated with minocycline-rifampicin and are effective in inhibiting the development of biofilm of both Gram-negative and Gram-positive bacteria, with the exception of P. aeruginosa and Candida spp.
Table 12.3 shows possible systemic treatment for CLABSI.
Pathogen | First line antibiotic | Alternative treatment |
---|---|---|
|
|
|
|
|
|
|
|
|
Pseudomonas | Carbapenem or piperacillin-tazobactam |
|
Candida spp. | Echinocandins or fluconazole | Amphotericin |
Candida glabrata | Echinocandins or fluconazole at higher doses if susceptible strains | Amphotericin |
Mechanical Circulatory Support Device (MCSD) Infections
Infections in MCSD patients are difficult to treat and have been associated with poor prognosis and poor quality of life. Ventricular assist devices differ from other cardiac devices by the fact that they have a percutaneous drive-line, which has been termed the ‘Achilles’ heel’ of these devices. The most recent INTERMACS report (March 2016) has shown that major infections in MCSD are the third highest cause of death with an overall death toll of 9% (June 2006 to March 2016), surpassed only by neurological dysfunction and multiorgan failure.
The results of a retrospective analysis of all VAD patients from the Mayo Clinic between 2005 and 2011 are shown in Table 12.4.
Table 12.4 Retrospective analysis of all VAD patients from the Mayo Clinic between 2005 and 2011
Infection type | Cases per 100 patient-years of LVAD support (95% CI) |
---|---|
All | 32.8 (26.7–39.9) |
Endocarditis | 1.6 (0.5–3.8) |
Pump and/or cannula infection | 4.9 (2.7–8.0) |
|
|
Pocket infection | 2.3 (0.9–4.7) |
Driveline infection | 15 (10.9–20) |
Permanent pacemaker/CRT-D infection | 1.6 (0.5–3.8) |
Mediastinitis, VAD related | 2 (0.7–4.2) |
The salient points of international standards for the management of MCSD infections as valid in June 2016 are presented below.
1. Bacterial infections:
(a) Gram-positive cocci are the most prevalent pathogens, followed by Gram-negative rods.
(b) Nosocomial pathogens – Pseudomonas, Serratia and Enterobacter – account for the second most common cause after Staphylococcus spp.
(c) The duration of antimicrobial treatment is not clearly established; most centres recommend courses of at least 2 weeks for superficial driveline infections and at least 4 weeks for deep-seated infections.
(d) Due to the wide variety of possible scenarios, there are currently no international guidelines on the treatment of bacterial infections associated with MCSD. The antibiotic agents should be administered after consultation with the local microbiology department, infectious diseases specialist and the transplant or heart failure specialist teams.
2. Fungal infections:
(a) The prevalence of fungal infections has increased over the years.
(b) Most fungal infections are caused by Candida spp., with a few other case reports of Aspergillus spp. and other yeasts.
(c) No evidence has demonstrated that the routine use of antifungal prophylaxis decreases the rate of fungal infections in MCSD patients; however, preimplantation prophylaxis should be considered in high-risk patients.
(d) Candida spp. pump or cannula infection should be treated with 8–12 weeks of amphotericin, echinocandin intravenously or high dose fluconazole if susceptible Candida strains.
(e) Deep-seated pocket or driveline infection should be treated with 8–12 weeks of echinocandin or amphotericin intravenously followed by prolonged suppressive oral therapy.
(f) Superficial driveline infections should be treated with 2 weeks of azole therapy.
Antifungals in the ICU
Fungal infections are associated with high morbidity and mortality in the critically ill patient and lead to very high healthcare costs. The prevalence of fungal infections in intensive care is increasing due to the increasing complexity of medical and surgical ICU patients, the presence of medium- and long-term devices, such as ECMO and VAD cannulae, and the use of broad spectrum antibiotics.
Although all species of yeasts can be encountered, Candida and Aspergillus spp. are by far the most prevalent in cardiothoracic ICU patients, so we will refer to treatment for these two pathogens in the discussion below.
1. Candida spp:
(a) The 2012 European guidelines recommend that fungicidal agents – echinocandins or lipid-based polyenes – be used for the initial treatment of invasive Candida infection; once the species of Candida and the sensitivities are identified, treatment can be de-escalated to azoles for susceptible strains.
(b) Polyenes are preferred to other classes for the treatment of deep-seated infections, such as mediastinitis or endocarditis.
2. Aspergillus:
(a) Caspofungin is the only echinocandin approved for the treatment of invasive aspergillosis; however, it is an inferior choice to voriconazole and amphotericin.
(b) Voriconazole is recommended as first line agent for aspergillosis; notably, it interacts with tacrolimus and cyclosporine by interfering with the hepatic metabolism pathways; the therapeutic levels of the immunosuppressants should be monitored and higher doses may be required.
(c) Lipid-based polyenes (amphotericin B) are recommended as second line therapy for aspergillosis, where voriconazole is contraindicated.
Antibiotic Resistance and the Development of New Agents
The last decade has seen worrying levels of increased antimicrobial resistance, prompting the Centre for Disease Control in the USA and similar organisations worldwide to declare the current situation an emergency. The Review of Antimicrobial Resistance has recently published a report outlining the problem and proposing several strategies of tackling the issue of the increase in antimicrobial resistance.
Which are the microorganisms causing these issues and where are they most likely to be encountered?
The Centre for Disease Control has published a list of the current microorganism resistance threats. We present the ones most likely to be encountered in a cardiothoracic critical care unit in Table 12.5.
Table 12.5 Microorganisms showing resistance
Urgent threats |
|
Serious threats |
|
Concerning threats | Vancomycin-resistant S. aureus (VRSA) |
There have been volumes written on how to best tackle the emergence of these infections in the ICU. The key message from organisations all over the world is to implement antibiotic stewardship programmes and improve the education of the ‘jobbing’ intensivists in the pharmacology of antimicrobials.
Antimicrobial stewardship is a bundle of interventions designed to optimise and measure the appropriate use of antimicrobials based on pharmacodynamics and pharmacokinetic data available, local ecology and patient and unit characteristics. A well-run stewardship programme is a two-stage process.
(a) Identification of patients with infection;
(b) Empirical antibiotics according to local policy and microbiological advice;
(c) Optimised dosing according to pharmacokinetics(PK)/ pharmacodynamics(PD).
(a) Review within 72 hours with laboratory results and clinical progress;
(b) Switch to narrow spectrum antibiotics (if broad spectrum antibiotics started initially);
(c) Stop antibiotics if the pathology is unlikely to be infection.
In Spain, a country with a high prevalence of multidrug resistant organisms (MDR), a recently implemented ‘zero resistance’ programme recommends that antimicrobials against MDR should only be administered in patients with septic shock and a high risk of MDR based on risk factors or knowledge of local ecology.
The following are risk factors for carriage of MDR.
1. Hospital admission for more than 5 days in the last 3 months.
2. Institutionalised patients (prison, healthcare, social centre).
3. Known previous colonisation or infection with MDR.
4. Antibiotic course of at least a week in the previous month, especially with one or more of the following:
(a) Third or fourth generation cephalosporins;
(b) Fluoroquinolones;
(c) Carbapenems.
5. End-stage renal disease or renal-replacement therapy.
6. Comorbid conditions associated with colonisation or infection with MDR, such as:
(a) Cystic fibrosis;
(b) Bronchiectasis;
(c) Chronic skin ulcers.
Even though these risk factors were highlighted in the context of the Spanish healthcare system, they are internationally recognisable as the situations which should alert most clinicians to the possibility of the patient being colonised by or having an infection with a MDR pathogen.
The arsenal available to combat these infections is, as explained earlier, getting more and more limited. Commonly used antibiotics to treat potential MDRs are complemented by some new preparations and combinations. Table 12.6 lists some of the most commonly available.
Table 12.6 Most commonly available new preparations against MDRs
Antibiotic | Active against | No activity | Remarks |
---|---|---|---|
Colistin (polymyxin E) |
|
|
|
Tigecycline |
|
| Can be used for deep-seated infections, note poor lung penetration of this antibiotic |
Carbapenems |
| Strains of Gram-positive cocci with reduced penicillin sensitivity | Emerging strains of carbapenemase-producing microorganisms (KPO) |
Glycopeptides (vancomycin, teicoplanin) |
| Gram-negative bacteria | |
Daptomycin |
| Gram-negative bacteria | Inactivated by alveolar surfactant – ineffective in pneumonias |
Linezolid |
| Gram-negative bacteria |
|
Fosfomycin |
| Some Pseudomonas and E. coli strains | High potential for development of resistance under prolonged therapy |
Ceftolozane/tazobactam | Indications:
| ||
Tedizolid |
|
|
|
Avibactam |
| ||
Plazomicin |
| Phase 3 clinical trials | |
Echinocandins |
|
Learning Points
The most common approach to empirical antibiotic treatment in severe infections in the critically ill is to ‘start broad and narrow down’.
Consider the most likely source of sepsis when deciding which antibiotics to start.
The rate of discovery of new antibiotics is lower than the rate of development of multidrug resistant strains in pathogens.
In the current context of drug resistant pathogens, antibiotic stewardship is a must.
In certain cases, be mindful of the pharmacokinetic and pharmacodynamics changes which occur due to critical illness pathophysiology.
Further Reading
MCQs
1. A 19 year old girl is admitted to the ICU following double lung transplant for cystic fibrosis. She was known to be previously colonised with multidrug resistant Pseudomonas. After initial satisfactory progress, the patient develops severe pneumonia on day 3 postoperatively. Talking to the anaesthetist for the case, it was a difficult intubation with a small double lumen tube and incomplete tracheobronchial toilet may have been performed. Suspecting that this is possible contamination with the patient’s previous flora, choose which antibiotic you would consider starting.
2. A 57 year old male presents to the A&E department of a peripheral hospital with a history of 3 weeks of fever, chills and malaise. In the last few hours, though, he is increasingly dyspnoeic and has started to cough pink, frothy sputum. His medical history is unremarkable apart from an aortic valve replacement for a stenotic bicuspid aortic valve 3 years previously. The cardiology registrar does a ‘quick TTE’ and, despite the poor windows, detects free aortic regurgitation and a rocking valve. What would you do next?
Send the patient urgently to the regional cardiac surgical centre and give a dose of intravenous flucloxacillin and gentamicin, with instructions to be continued for 6 weeks or until microbiological advice
Keep the patient in overnight, start a vasoconstrictor to help increase the perfusion pressure to the brain and start piperacillin/tazobactam and gentamicin intravenously
Send the patient urgently to the regional cardiac surgical centre and give a dose of intravenous vancomycin with gentamicin and rifampicin, with instructions to be continued for 6 weeks or until microbiological advice
Intubate the patient prior to transfer and give fluids to compensate for increased capillary permeability with sepsis; start a course of rifampicin
Keep the patient in overnight, start a diuretic, give fluid boluses and a vasoconstrictor to maintain MAP > 65 mmHg; start vancomycin with ciprofloxacin and fluconazole
3. An acceptable initial choice of antibiotics for presumed ventilator associated pneumonia in a non-penicillin-allergic patient who is otherwise progressing well would be:
4. A 36 year old woman with a LVAD for postpartum cardiomyopathy is referred to the parent cardiac centre with fever and chills. The device has been alarming increased power for the last 24 hours. She has a history of frequent driveline infections with MSSA and C. albicans. What is the choice of antibiotics if we presume that LVAD infection is a possible diagnosis?
5. The risk of CLABSI is highest with:
Long-term catheter insertion in the radiology department
Exercise answers are available on p.467. Alternatively, take the test online at www.cambridge.org/CardiothoracicMCQ
Introduction
Along with cardiothoracic operating departments, cardiothoracic intensive care units are some of the greatest consumers of blood products in the world; so for example UK cardiac surgical centres use approximately 10% of the national supply. Furthermore, use of blood products is currently on the increase due to a combination of factors, including advancing patient age, increasing burden of comorbidities, expanding use of antiplatelet therapy and greater surgical complexity. Importantly, transfusion of blood products is associated with increased morbidity and mortality thus it is imperative to employ strategies such as patient blood management (PBM) to avoid unnecessary transfusion and conserve precious resources.
Blood Products
More than 2 million donations are made each year in the UK to National Health Service Blood and Transfusion (NHSBT). From these donations, approximately 2,000,000 units of red cells, 300,000 units of platelets, 350,000 units of FFP and 126,000 units of cryoprecipitate are prepared. The current commonly prescribed blood products in the UK are listed in Table 13.1.
Table 13.1 Comparison of blood products and components currently in use in the UK
Commercial product | Protein | Platelet | Fibrinogen | II | V | VII | VIII | vWF | IX | X | XI | XII | XIII | Protein C | Protein S | Antithrombin | Heparin | Red cell stroma | Blood group specific | White cell contaminant | Clinically important subtypes | Donor exposure | Pathogen inactivation or reduction | |||
---|---|---|---|---|---|---|---|---|---|---|---|---|---|---|---|---|---|---|---|---|---|---|---|---|---|---|
Blood components | Packed red cells | Haemoglobin <40 g/l | trace | trace | trace | trace | trace | trace | trace | trace | trace | trace | trace | trace | trace | trace | trace | No | Yes | Yes | <5106/unit | washed, CMV, negative, irradiated | 1 | Leukodepletion (UK) | ||
Platelets | >240 × 109/l | trace | trace | trace | trace | trace | trace | trace | trace | trace | trace | trace | trace | trace | trace | No | trace | Yes | <5106/unit** | pool, single donor apheresis, HLA matched | 1 to 6 | Leukodepletion (UK) | ||||
FFP | >50 g/l | nil | 0.9 g/l | variable | variable | variable | >70 U | variable | variable | variable | variable | variable | variable * | variable * | variable * | variable * | No | trace** | Yes | <5106/unit** | 1 | Leukodepletion (UK) | ||||
Cryoprecipitate | nil | >0.7 g/pool | variable | variable | variable | 350 U/pool | variable | variable | variable | variable | variable | variable | variable | variable | variable | No | trace** | Yes | <5106/unit** | 5 | Leukodepletion (UK) | |||||
Methylene blue FFP | >50 g/l | nil | 0.7 g/l | variable | variable | variable | 50 U | variable | variable | variable | variable | variable | variable | variable | variable | variable | No | trace** | Yes | <5106/unit** | 1 | Methylene blue | ||||
Methylene blue cryo | nil | 0.7 g/pool (80% of untreated) | variable | variable | variable | 350 U/pool (80% of untreated) | variable | variable | variable | variable | variable | variable | variable | variable | variable | No | trace** | Yes | <5106/unit** | Methylene blue | ||||||
Blood products | Fibrinogen concentrate | Riastap ® | Albumin | nil | 20 g/l | No | nil | No | No | >20,000 | Pasteurisation | |||||||||||||||
Prothrombin complex | Beriplex ® | 6–14 g/l | nil | NA | 20–60** | NA | 10–25** | NA | NA | 20–31*** | 22–60*** | NA | NA | 15–45 | 12–38 | Yes | Yes | nil | No | No | >20,000 | Filtration, pasteurisation | ||||
Octaplex ® | 1.3–4.1 g/l | nil | NA | 20–76*** | NA | 18–48** | NA | NA | 50*** | 36–60*** | NA | 26–62** | 24–64** | NA | Yes | nil | No | No | >20,000 | Filtration, pasteurisation | ||||||
SD FFP | 45–70 g/l | nil | NA | NA | 31 | NA | 28 | NA | NA | NA | NA | NA | NA | 50 | NA | No | nil | Yes | No | >20,000 | Solvent detergent |
* Assumed to be 100% activity but donors will vary 70–100%.
** Prior to freezing.
*** Per ml reconstituted.
Beriplex ® and Riastap ® are trademarks of CSL Behring.
Octaplex ® is a ® trademark of Octapharma.
Blood components are not blood group specific due to pooling of donors in the production process. This means equilibration of interindividual variation from donor to donor and the dilution of hazardous antibodies such as antineutrophil antibodies. It is important to note that some products have traces of heparin and may be potentially contraindicated in heparin induced thrombocytopenia. Red cells are considered suitable in the UK for transfusion for up to 35 days post donation and are stored at 4 oC ±2 oC, FFP and cryoprecipitate are usable for up to 2 years and are stored at −30 oC. Platelets have a shelf life of 7 days if stored at room temperature with agitation.
To reduce the risk of transmission of infection, all donors complete a health questionnaire prior to donation to identify those that may be unsuitable. Any donations taken are then tested for a range of infections including HIV 1 and 2, syphilis, HTLV1 and 2, hepatitis B and hepatitis C. As of April 2016, NHSBT also commenced screening for hepatitis E negative blood for transfusion to some patients who are immunosuppressed due to haematological malignancy or who are scheduled for or have undergone solid organ or bone marrow transplantation. Several initiatives are also in place to reduce the risk of transmission of Variant Creutzfeldt-Jakob disease including leukodepletion (1999) and the use of non-UK methylene blue treated plasma components or commercial solvent/detergent fresh frozen plasma (SD FFP) treated pooled plasma for patients born after 1995.
Red Blood Cells
It has been shown that isovolaemic haemodilution of normal volunteers and non-anaemic patients is tolerated to a haemoglobin of 50 g/l. Studies that have assessed patients who refuse blood found that the degree of preoperative anaemia affected the risk of death but did not have an effect on mortality. Hebert showed in a randomised controlled trial (TRICC) that a restrictive (70 g/l) transfusion strategy was superior to a liberal (90 g/l) one in ICU patients. However, some doubt has been cast on this in the setting of cardiothoracic surgery after a recent study reported improved outcomes with a liberal strategy. In the TITRe2 trial, patients undergoing elective cardiac surgery were randomised between 90 g/l and 75 g/l as the transfusion threshold. Ischaemic cerebral events, myocardial infarction, infection or acute renal failure within 3 months of surgery were non-significantly different. This leads to transfusion rates of 92.7% versus 53.4%. Strikingly, the mortality was different, 2.6% versus 4.2%, in the two groups with a hazard ratio of 1.67 (1.00–2.67) (p = 0.045) favouring the higher threshold.
Accepted side effects of transfusion are infection, immune reactions, volume overload, immune suppression, and red cell and HLA allo-immunisation. General recommendations agree that a Hb of <70 g/l is an indication for transfusion; however when the Hb is >70 g/l the circumstances of the patient need to be taken into account and the risk/balance of transfusion assessed. In patients with a Hb of 80–100 g/l, the transfusion decision should be individualised and generally a transfusion is not indicated with a Hb of >100 g/l. However, these recommendations do not include patients with coronary artery disease.
Platelets
We usually apply the threshold of platelets <100 × 109/l in the immediate postoperative setting. Controversies exist for patients with concomitant antiplatelet therapy. Patients exposed to antiplatelet therapy take several days to recover normal platelet function: clopidogrel (7 days), aspirin (7 days), ticagrelor (5 days) and prasugrel (7 days). Cangrelor is an interesting alternative ADP-inhibitor that allows rapid platelet function recovery within a few hours of stopping administration. Despite the recent development of near patient platelet function testing, the problem remains that the platelet function testing becomes abnormal with reducing platelet count (around 75 × 109/l for the Multiplate). Moreover, the treatment of confirmed or assumed platelet dysfunction is platelet transfusion. The British Commission for Standards in Haematology have published guidance for platelet transfusion. The evidence for the use of platelet transfusion is marred by the impact of ongoing antiplatelet therapy on both the patient’s own and transfused platelets. The general advice is to discontinue antiplatelet therapy where possible, taking into account the time required to normalise platelet function. Platelet transfusion in patients requiring antiplatelet therapy may increase the risk of atherosclerotic complications while not positively affecting platelet function.
During the intensive care stay there is controversy about the optimal platelet count for preventing bleeding with a number of invasive procedures. The insertion or removal of epidural catheters is considered safe with platelet counts >(80–100) × 109/l. The majority of procedures, such as line insertions or chest drain removals, are safe with platelet counts over 50 × 109/l provided concomitant anticoagulation has been corrected or stopped. Our practice, with the exception of epidurals and lumbar punctures, is to transfuse platelets for counts <50 × 109/l prior to intervention and to not transfuse platelets for counts of >80 × 109/l. Between 50 and 80 × 109/l we will reserve a unit of platelets for 8 hours for the patient in case of excessive bleeding or if the clinician requests these.
In patients on ECMO the agreed platelet threshold is currently debated. We accept 100 × 109/l in a bleeding patient but have had individual patients where we tried to achieve higher platelet counts due to concomitant intracranial bleeding with a continued need for heparin anticoagulation. Others advocate thresholds as low as 20 × 109/l. The coagulation system in adults and children is fundamentally different and therefore it is not likely that the same threshold can be applied for both groups of patients.
In individual patients who develop thrombocytopenia while on ECMO, a number of possible causes such as drugs, mechanical factors or immune mechanisms have to be considered. Where there is an associated bleeding pattern this is important and the need for ongoing anticoagulation needs to be reviewed. Where reduced anticoagulation therapy can be compensated with measures such as increasing the flow of the ECMO circuit, this is likely to be more effective than a small increment in platelet count. Any transfused platelets will be subjected to the same causes of thrombocytopenia as the patient, and particularly in moderate thrombocytopenia are unlikely to have a prolonged effect.
We assume that a unit of platelets will contain a minimum of 250 × 109/l per adult therapeutic dose. In a healthy adult we assume a daily platelet production of 10 × 109/l. A platelet transfusion is clinically most effective in a patient with thrombocytopenia. The platelet recovery following a fixed dose infusion of platelets is highly variable and is dependent on the original reason for thrombocytopenia in the patient. It is bound to be optimal in a patient with lack of production but far less satisfactory in a patient with platelet destruction. There are a number of specific cardiothoracic interventions that will reduce the platelet recovery in a given patient such as extracorporeal membrane oxygenation (ECMO) and intra-aortic balloon pump (IABP).
Human platelet concentrates express very few AB antigens and are suspended in less than 50 ml of plasma. For this reason ABO groups can be overridden in case of an emergency. Platelets do however express HLA class I antigens, which can lead to antibody formation causing platelet refractoriness (defined as repeated platelet increments of <5 × 109/l). Red cells are more potent HLA type sensitisers than platelet transfusions and multiparous women are particularly at risk. The UK national blood service is able to provide HLA class I typing and antibody screening with a view to providing HLA selected/matched platelets. These products are considerably more expensive than standard platelets but may lead to better increment and thus overall reduction in platelet usage. All HLA matched platelets must be irradiated.
Cryoprecipitate and Fibrinogen Concentrate
Fibrinogen is a critical haemostatic factor for the development of effective local clotting in all surgical patients, and in particular for the management of perioperative bleeding in cardiac surgical patients. Normal fibrinogen levels are 200–400 mg/dl in the non-parturient, but may be >400 mg/dl during the third trimester of pregnancy. The optimal transfusion threshold for fibrinogen replacement is unknown. There is no RCT evidence for the transfusion threshold of 1 g/dl but it has been accepted in clinical practice. More recently, higher thresholds for fibrinogen replacement have been advocated with thresholds of up to 2 g/dl. The massive transfusion guidelines published by the BCSH advise use of a cut-off of 1.5 g/dl as the threshold, as do the NICE transfusion guidelines of November 2015.
An adult dose of cryoprecipitate (10 units or 2 pools) is equivalent to 2–4 g of fibrinogen concentrate. Based on the relative content of fibrinogen per ml, fibrinogen concentrate should be superior to cryoprecipitate in restoring low fibrinogen levels. However, cryoprecipitate also contains factors such as vWF and factor XIII which may be of therapeutic benefit. Importantly, a therapeutic dose of 4 g of fibrinogen is four times as expensive as cryoprecipitate.
Ranucci et al. recently reported a prospective, randomised, blind study of 116 patients undergoing high risk cardiac surgery with an expected cardiopulmonary bypass duration of >90 minutes. Patients received fibrinogen concentrates after protamine administration and prothrombin complex concentrates (PCCs) if bleeding persisted. Patients receiving fibrinogen had a significantly lower rate of any allogeneic blood product transfusions, including PRBCs and FFP. Postoperative bleeding was also reduced in the fibrinogen treated patients (median, 300 ml; interquartile range, 200 to 400 ml) compared with the control patients (median, 355 ml; interquartile range, 250 to 600 ml). However, the recently published REPLACE-trial comparing the early aggressive use of fibrinogen concentrate in patients undergoing aortic surgery with placebo, on the background of a defined transfusion algorithm with platelets and FFP, did not demonstrate clinical benefit.
Fresh Frozen Plasma and Prothrombin Complex Concentrate
Fresh frozen plasma may increase clotting factor activity by up to 30% with administration of 15 ml/kg. The absolute increment depends on the baseline factor activity in 40 ml/kg of patient plasma and may be considerably smaller if the clotting factor levels are nearly normal. There may be considerable variation in the concentration of individual factors such as factor XI or IX between donors. In some congenital coagulation factor deficiencies, it may therefore be advisable to use pooled products to minimise these effects between donors.
Prothrombin complex is a pooled plasma product that contains factors II, VII, IX and X as well as Protein C and S. It is licensed for use in acquired deficiency of the vitamin K dependent clotting factors. The most recent guidelines (2015) on the treatment of massive haemorrhage explicitly advise against the use of prothrombin complex in massive transfusion outside studies. The main advantage of using PCC over FFP is the reduced volume and easier administration. An FFP equivalent dose is 15 U/kg, and there are published dosing recommendations for warfarin reversal based on baseline INR with up to 50 U/kg. The available PCCs also contain traces of heparin so are contraindicated for the reversal of warfarin in patients with HIT.
Recombinant Activated Factor VIIa
Recombinant FVIIa is used off-label as a prohaemostatic agent for life threatening hemorrhage. Its mechanism of action is thought to be related to binding with tissue factor (TF) expressed at the site of vascular injury to locally produce thrombin and amplify haemostatic activation. Controlled clinical trials report the incidence of thrombotic complications among patients who received rFVIIa to be relatively low and similar to that among patients who received placebo. In the most recent cardiac surgical study, patients bleeding postoperatively >200 ml/hour were randomised to placebo, 40 µg/kg rFVIIa or 80 µg/kg rFVIIa. Primary endpoints were the number of patients suffering critical serious adverse events. Secondary endpoints included rates of reoperation, blood loss and transfusions. Although more adverse events occurred in the rFVIIa groups, they did not reach statistical significance. However, after randomisation, significantly fewer patients in the rFVIIa group underwent a reoperation because of bleeding or needed allogeneic transfusions.
Bleeding and Coagulopathy
About 50% of cardiac surgical patients receive red cells, 30% of which also receive blood product combinations including platelets or plasma. Established risk factors for transfusion include preoperative anaemia, low body mass index, complexity and urgency of surgery.
The cell based model of haemostasis is complex and not easily reproducible in vitro (Figure 13.1). Haemostasis is initiated following tethering of platelets to a site of vascular injury through vWF and GPIb/V/IX. The platelet membrane undergoes activation and mediators are released. Traces of tissue factor stabilise the factor VIIa, which in turn activates traces of IXa, Xa and thrombin. This sets off an autofeedback mechanism that propagates self-activation and results in fibrin polymerisation, followed by thrombin inactivation.
Figure 13.1 Cell based model of haemostasis.
Contact activation occurs with activation of the four contact factors: K, PK, HK and XII. This leads to activation of the intrinsic pathway and activated complement. Both the activation of the complement pathways and the formation of thrombin activate platelets and lead to platelet aggregation and fragmentation. Platelet activation, in turn, activates endothelial cells and causes release of tPA platelet activation.
K kallikrein, PK prekallikrein, HK high-molecular-kininogen, F factor, a activated form, f fragmented form, PG prostaglandin, NO nitrous oxide, TXA2 thromboxane A2, OR oxygen radicals, 5HT serotonin, IL1 interleukin 1, PAF platelet activating factor, tPA tissue plasminogen activator.
The effects of CPB on haemostasis are also complex and multifactorial. During surgery the majority of the surgical field is bypassed by the circulating blood; the patient’s blood is haemodiluted with crystalloid and albumin. Hypothermia in combination with partial activation of the clotting cascade prolongs the clotting reaction further. Primary haemostasis is impaired by platelet consumption and degranulation. Platelets may also have been exposed to antiplatelet agents in the immediate preoperative phase, which will have reduced platelet activation further. High doses of unfractionated heparin inhibit the majority of the serin proteases in an antithrombin dependent manner. If administered in high doses, the half-life of heparin is prolonged, ranging between 30 and 90 minutes. Elimination is via renal and hepatic mechanisms in addition to poorly delineated pathways involving the macrophages and endothelial cells. Sequestration sites can be a source of heparin rebound, which is sometimes observed in patients who have received exceedingly high doses of heparin during CPB for up to 12 hours. Tissue debris is aspirated by cardiotomy suckers from the surgical field and mixed with the other blood and recirculated, adding a source of extrinsic clotting factor activation.
Diagnostic Testing
The majority of cardiac patients have postoperative thrombocytopenia and the clotting factors, if measured, can be as low as 25–50% of baseline immediately after separating from CPB. A gradual recovery occurs over the next 2–4 days. No single laboratory test has been shown to be predictive of postoperative clinical bleeding and unselected correction of platelet count or fibrinogen has had only disappointing results.
Preoperative Screening
It is currently accepted practice to screen aPTT and PT preoperatively along with full blood count (FBC). The reason for screening PT and aPTT is an anachronism dating back to when warfarin and unfractionated heparin were the main anticoagulants in use. The FBC is more important than ever in that it allows screening for preoperative anaemia or thrombocytopenia, which may be amenable to preoperative intervention. Generally we accept platelet counts >90 × 109/l, aPTT <38 s and PT <18 s, assuming the patient is not on anticoagulants. A bleeding score is more useful to identify patients with a potential undiagnosed bleeding disorder.
Blood Tests Used To Assess and Direct Treatment of Post CPB Coagulopathy
The majority of centres now use a combination of laboratory and point-of-care assays in combination with clinical assessment to guide transfusion of products according to predefined algorithms (see Figure 13.2); for further details see Chapter 36.
Figure 13.2 Part of the rationale for use of blood products is the use of preagreed transfusion algorithms to restore near normal haemostasis.
Laboratory Based Tests
The conventional laboratory tests in use are the PT/INR, aPTT, Clauss fibrinogen and thrombin time. Others include anti-Xa level. FBC is also essential. Generally it is assumed that thrombocytopenia will respond clinically to platelet transfusions up to 100 ×109/l. PT >20 s and aPTT >48 s correct with FFP. These screening tests tend to become prolonged when the levels of some individual factors have decreased to 40% or less.
The main drawback of using laboratory based assays is the slower turnaround time, which may be as long as 40 minutes. Furthermore, these assays are sensitive to high heparin levels. Thrombin time allows the detection of heparin and interpretation of prolonged clotting tests in complex patients. It is more sensitive to heparin than aPTT, and a TT value >25 s suggests heparin contamination. In the context of recently discontinued warfarin therapy, mildly prolonged aPTT and PT may hint at potentially correctable deficits in a patient with protracted bleeding. Dabigatran or argatroban may similarly potentially produce falsely low fibrinogen results.
Point-of-Care (POC) Testing
POC testing is firmly establishing itself in the setting of cardiothoracic surgery. The benefit of these tests is the rapid turnaround and the ability to detect hypofibrinogenaemia at the POC. The drawback is the relative insensitivity to some of the more subtle imbalances of coagulation such as warfarin or heparin therapy for which laboratory based assays have been validated and have a long track record.
The two dominant technologies are thromboelastography (TEG) and rotational thromboelastometry (ROTEM). A third, the Sonoclot, was evaluated by NICE in 2014 but was not deemed suitable to be used outside a clinical trial. All the viscoelastic assays benefit from a number of activator kits which usually include a PTT like reagent with kaolin or celite, a tissue factor based activator (EXTEM or fastTEG), a fibrinogen activator (functional fibrinogen, or FIBTEM) and a heparinase containing Celite or kaolin assay. Manufacturers are developing a cartridge based solution that allows the simultaneous assessment of several of the assays at once in varying combinations to improve reliability.
The TEG is able to assess platelet function in addition to the above tests by making the reaction dependent on ADP platelet activation or arachidonic acid based activation. Another potentially useful test is the Multiplate platelet function assay. This utilises electric impedance to detect platelet function/aggregation in whole blood. Results are available in under 10 minutes but one of the problems with the test is the interference from low platelet counts, which may be a secondary reason for a lack of detectable platelet function perioperatively.
Blood Conservation
PBM is an international initiative based on the Australian experience of using a multimodal approach to optimise red cell mass, minimise blood loss and harness the physiological tolerance of anaemia, so as to reduce the need for transfusion, improve patient outcomes and ensure that blood products are available for those patients that absolutely require transfusion. The UK Department of Health has published guidelines which can be downloaded freely and encompass a range of interventions beginning with rapid diagnosis of preoperative anaemia and correction of reversible causes when possible prior to surgery, the use of cell salvage and adjuncts such as fibrin glue, along with targeted reversal of anticoagulants and algorithms to correct coagulopathy, so as to minimise perioperative blood loss, and use of a restrictive policy of diagnostic testing to reduce the impact of repeated blood sampling on postoperative haemoglobin.
Future Outlook: Anticoagulant Reversal with Beriplex, Idaricuzimab and Adexanet Alfa
Four new oral anticoagulants have come on the market over the past 5 years. While the adoption was slow initially, now patients present for urgent or elective surgery while on these drugs. There are set recommendations by the manufacturers with regard to pausing treatment prior to surgery based on renal function and risk of surgery. Tranexamic acid and correcting other correctable factors like thrombocytopenia may also help.
Prothrombin complex has become the reversal agent of choice of the novel anticoagulants with doses of 25–50 IU/kg (albeit not licensed). This has now been superseded for dabigatran by idaricuzimab, a monoclonal antibody for dabigatran. We are expecting that adexanet alfa (a dummy factor Xa molecule) will receive a license in the near future for Xa inhibitors for specific reversal.
Learning Points
Blood products and components vary in their indications, and internationally and randomised control trials to determine their optimal use are lacking.
Recombinant products are only available for a minority of indications such as congenital factor VIII deficiency.
Recombinant factor VIIa was inferior to best conventional management in cardiothoracic surgery.
Point-of-care testing has advantages over laboratory testing of turnaround time and global nature. Laboratory assays by comparison have a defined advantage in their reproducibility and use in transfusion algorithms.
Prothrombin complex has become the unlicensed reversal agent of choice for direct oral anticoagulants (DOACs). Specific reversal agents will probably be available in the near future.
Further Reading
MCQsWhich of the following statements is true?
1. UK Plasma:
Is being used to manufacture plasma derived single factor concentrates where so far there is no recombinant option
2. Point-of-care testing:
3. Laboratory based testing:
4. Blood products:
5. Transfusion associated risks:
Patients who refuse blood on grounds of lack of safety of the blood supply should be offered concomitant antiretrovirals
A liberal transfusion threshold >90 g/l was superior to 75 g/l in the T2 study
Exercise answers are available on p.468. Alternatively, take the test online at www.cambridge.org/CardiothoracicMCQ
Introduction
Intravenous fluid administration is performed in critically ill patients to maintain fluid volume homeostasis and to achieve many other goals. Intensivists and perioperative physicians who care for the cardiothoracic critically ill patient must appreciate that fluids are ‘drugs’ and therefore it is necesssary to understand their physiology and pharmacology. Appropriate fluid resuscitation is often the first intervention for the haemodynamically unstable patient. There is ongoing debate regarding whether the fluid chosen for these patients should be a crystalloid solution (i.e. isotonic saline, Ringer’s lactate) or a colloid containing solution (i.e. albumin solution, hyperoncotic starch). Large international surveys have shown that choice of fluid is mostly a matter of institutional preference rather than due to specific procedural/patient related factors.
Pathophysiology
There are several determinants in assessing the need for intravascular volume replacement in the context of cardiac surgery: (i) blood loss, (ii) increasing vascular capacitance as often occurs with patient rewarming, (iii) third space fluid losses secondary to cardiopulmonary bypass (CPB) induced systemic inflammation, and (iv) increased cardiac preload requirements in the setting of transient cardiac ischaemia – reperfusion injury, myocardial stunning and reduced ventricular compliance.
Total body water for the average 70 kg adult male is about 45 l of which 30 l (65%) is intracellular and 15 l (35%) is extracellular fluid. The extracellular space can be subdivided into interstitial space (10l) and intravascular space (5 l). All three compartments also contain electrolytes, including ions and larger molecules. The cellular membranes maintain potential differences via a complex differential concentration between intracellular (potassium) and extracellular (sodium) ions. In addition, proteins and large molecules maintain the colloid distributions between intracellular, interstitial and intravascular spaces.
The fate of any intravenous fluid given into the vascular compartment depends on many factors including the electrolyte and colloid (if any) compositions, the patient’s intravascular volume status, the total volume of fluid administered, and the integrity of endothelial glycocalyx. The endothelial glycocalyx modulates vascular permeability and inflammation and according to the Starling model it is a key determinant of fluid disposition. The primary forces defining transcapillary fluid movement and the counterbalancing process of fluid movement into the vascular space in a normal and damaged vasculature are shown in Figure 14.1. It has been shown that CPB is associated with loss of glycocalyx integrity and microvascular dysfunction. Interestingly, significant shedding of the glycocalyx also occurs during off-CPB procedures. In critical illness, including post-CPB/postoperative states, endothelial glycocalyx denudation causes high transcapillary movement of fluid leading to tissue oedema and inadequate intravascular volume expansion after intravenous fluids are administered.
Figure 14.1 The opposing forces defining the steady state net flow of fluid from the capillary into the interstitial space are defined by the hydrostatic pressure differences between the capillary lumen pressure (Pc) and interstitial pressure (Pi) as opposed by the filtration coefficient (Kf) which itself is a function of the vascular endothelial cell integrity and the intraluminal glycocalyx. This net efflux of fluid out of the capillary into the interstitium is blunted by an opposing oncotic pressure gradient moving fluid in the opposite direction because capillary oncotic pressure (πc) is greater than interstitial oncotic pressure (πi). And like hydrostatic pressure dependent flow, oncotic dependent flow is blunted by the reflection coefficient (σ), which like Kf is a function of the glycocalyx and vascular endothelial integrity. Under normal conditions (left side), both Kf and σ are high, minimising fluid flux resulting in a slight loss of plasma into the interstitium which is removed by lymphatic flow. However, if the vascular endothelium and glycocalyx are damaged (right side), oncotic pressure gradients play a minimal role because a large amount of protein-rich plasma translocates into the interstitial space, minimising the oncotic pressure gradient, whereas the constant Pc promotes massive fluid loss and interstitial oedema.
Fluids in the Cardiothoracic ICU
Colloids Versus Crystalloids
There is a lack of prospective data with special regard to ‘ideal’ fluid in the cardiac surgical patient population. Colloid fluids would be expected to produce a much larger volume expanding effect. Usual teaching suggests that the colloid to crystalloid volume expansion efficacy is 1:3 (300% greater efficacy for colloids), whereas the colloid to crystalloid ratio for volume expansion in adequately powered and well-designed randomised controlled trials (RCTs) was 1:1.2–1:1.4 (approximately 30% greater efficacy for colloids). The contents of solutes in colloid and crystalloid solutions are presented in Tables 14.1 and 14.2.
Table 14.1 Composition of currently available crystalloid solutions
Fluid/solute |
|
|
|
|
|
|
|
|
|
|
---|---|---|---|---|---|---|---|---|---|---|
Plasma | 142 | 4 | 103 | 5 | 3 | 290 | 2 | — | — | 1 |
| 154 | — | 154 | — | — | 230 | — | — | — | 50 |
| 77 | — | 77 | — | — | 203 | — | — | — | 50 |
NaCl 0.9% | 154 | — | 154 | — | — | 308 | — | — | — | — |
Lactated Ringer’s | 130 | 4 | 109 | 3 | — | 273 | 28 | — | — | — |
Dextrose 5% | — | — | — | — | — | 252 | — | — | — | 50 |
Plasmalyte 148 | 140 | 5 | 98 | — | 3 | 294 | — | 23 | 27 | — |
Normasol | 140 | 5 | 98 | — | 3 | 294 | — | 23 | 27 | — |
Table 14.2 Composition of currently available colloid solutions
Fluid/solute |
| pH |
|
|
|
|
---|---|---|---|---|---|---|
Gelofusine | 274 | 7.4 | 154 | <0.4 | <0.4 | 125 |
Hetastarch | 308 | 4.0–5.5 | 154 | — | — | 154 |
Haemaccel | 301 | 7.4 | 145 | 5 | 6.25 | 145 |
Pentastarch | 326 | 5.0 | 154 | — | — | 154 |
Albumin 4.5% | 7.4 | <160 | <2 | — | 136 |
It would stand to reason that early during elective cardiac surgery colloid fluids may have a better volume expansion effect than crystalloids as capillary pressures are likely to be normal and loading with crystalloids would produce a dilutional effect on plasma oncotic pressure leading to tissue oedema and a reduced volume expansion effect.
Crystalloids are preferred over colloid containing solutions for the management of patients with severe hypovolaemia not due to bleeding. Saline solutions seem to be as effective as other crystalloid solutions and colloid containing solutions, and are much less expensive. It is recommended that hyperoncotic starch solutions are avoided as they increase the risk of acute kidney injury, need for renal replacement therapy (RRT) and mortality. They may also lead to hypernatraemia, hyperchloraemia and acidosis. There was a black box warning from FDA for heat starch.
In a 9 year multicentre open label trial (CRISTAL), 2857 patients with hypovolaemic shock, due to any cause, were randomly assigned to fluid resuscitation with colloid or crystalloid. There was no difference in 28 day mortality or need for RRT between the two arms. Patients treated with colloids had more mechanical ventilation free days (13.5 versus 14.6 days) and vasopressor therapy (15.2 versus 16.2 days), as well as a lower 90 day mortality (31% versus 34%). However, the open label design and heterogeneity of fluids that were compared between the groups limit confidence in the apparent benefit of colloid solutions in this population.
Albumin
In theory, albumin has two possible advantages over crystalloid solutions: (i) more rapid plasma volume expansion, since the colloid solution remains intravascularly (in contrast to saline, three quarters of whichenters the interstitium) and (ii) lesser risk of pulmonary oedema, since dilutional hypoalbuminaemia will not occur. Well-designed RCTs and meta-analyses have failed to demonstrate benefits from the use of albumin. However, in some cardiothoracic intensive care units albumin remains the colloid of choice for patients undergoing lung transplantation and pulmonary endarterectomy when volume expansion is needed in the immediate postoperative period, in an attempt to reduce the risk of early reperfusion lung injury.
Hydroxyethyl Starch (HES)
Use of hyperoncotic starch solutions has been associated with increased incidence of acute kidney injury (AKI) and high mortality in some studies. In a RCT, 7000 patients were randomised to receive 6% HES or normal saline for all fluid resuscitation until ICU discharge. There was an increased incidence of AKI requiring RRT in the HES group compared with the saline group (5% versus 5.8%). Two meta-analyses, one of which excluded seven trials that were retracted due to scientific misconduct of one investigator, found that compared to conventional fluid resuscitation regimens, HES was associated with increased risk of mortality and need for RRT. HES associated AKI appears to be related to pinocytosis of metabolites into renal proximal tubular cells after glomerular filtration. HES has also been associated with increased risk of bleeding, which is probably due to impaired fibrin polymerisation and decreased factor VIII, vWF and XIII levels and not associated with haemodilution. Given the fact that the cardiac surgical patient population is at risk of AKI and coagulopathy anyway, the use of HES in the setting of cardiac surgery is not recommended.
Crystalloids
One of the most commonly used crystalloid solutions is 0.9% sodium chloride or ‘normal’ saline, which is anything but ‘normal’ or ‘physiological’ as it is slightly hypertonic and contains equal amounts of sodium and chloride making it hypernatraemic and very hyperchloraemic relative to plasma. Administration of high volume ‘normal’ saline will result in hypernatraemia, hyperchloraemic acidaemia leading to renal vasoconstriction and reduction in renal blood flow. This has led to suggestions that physiologically buffered fluids with a chemical composition that approximates extracellular fluid (e.g. Ringer’s lactate solution, Hartman’s solution, Plasma-lyte 148, Accusol) are used instead of ‘isotonic’ saline for large volume resuscitation. A recent RCT which enrolled healthy volunteers, and large observational series, have demonstrated that adverse effects associated with saline use were not evident when physiologically buffered fluids were used.
Hypertonic Solutions
Hypertonic solutions typically used in cardiac surgery include 2–7% saline. They have been used in an attempt to minimise volume overload and produce cellular dehydration in the context of cerebral oedema. The majority of the trials of hypertonic solutions have been in patients with traumatic brain injury. The results cannot be extrapolated for the general cardiac surgical population. However, cardiac surgical patients with cerebral oedema may benefit from hypertonic saline solutions on an individual basis. Studies examining use of hypertonic solutions did not examine their effect on renal function and reported a high incidence of hypernatraemia.
Fluid Balance Management
Positive fluid balance has been associated with worse outcomes in some but not all critically ill patient populations. A recent observational study that included a large cohort of medical, surgical and cardiothoracic ICU patients showed that positive fluid balance at the time of ICU discharge was associated with increased risk of death, after adjusting for markers of illness severity and chronic medical conditions, particularly in patients with underlying heart or kidney disease.
A secondary analysis of the FACTT trial demonstrated that patients with ARDS who developed AKI had a higher mortality (independent of a conservative or liberal fluid administration strategy). This study demonstrated some evidence of causality, as diuretic use was associated with a protective effect, potentially due to its effect on fluid balance. When the diuretic effect was adjusted for fluid balance, the protective effect was attenuated, thus potentially suggesting that the modulation of fluid balance promoted the benefit.
Intravenous fluid administration is a common intervention used for both the prevention and treatment of AKI. The pathogenesis of AKI in cardiac surgical patients is multifactorial, not only due to perturbed haemodynamics but also the result of direct cellular injury as well as indirect injury from inflammation and microcirculatory changes. AKI with oliguria as well as fluid resuscitation often results in accumulation of excess total body fluid. This fluid accumulates in all tissues of the body, and through the interstitial space, as well as remaining within the vascular space, resulting in increased venous pressure. The presence of oliguria is associated with a poor prognosis; however, it remains unclear whether this is due to severity of injury or due to fluid overload. Most studies to date have been conducted in the general ICU population. Similar findings have been reproduced in the cardiac surgery population, as early administration of fluid can lead to AKI. In a prospective observational cohort of 100 patients undergoing cardiac surgery, those patients in the quartile receiving the highest volume of fluid suffered the highest degree of AKI. This study was limited by the small number of patients included.
It has been demonstrated that positive fluid balance in the first 3 postoperative days is associated with primary graft dysfunction after lung transplantation. Patients undergoing pulmonary endarterectomy constitute a unique patient population at risk of development of ARDS due to high permeability lung injury. Therefore restrictive fluid management strategies and adequate diuresis in the first 48 hours (aiming for 2 l negative fluid balance) is of paramount importance. Negative fluid balance should also be maintained during the first week after lung resection surgery. Fluids should be limited because lung resection decreases the pulmonary vascular bed and overhydration will result in impaired gas exchange, need for prolonged mechanical ventilation, prolonged length of stay and high mortality.
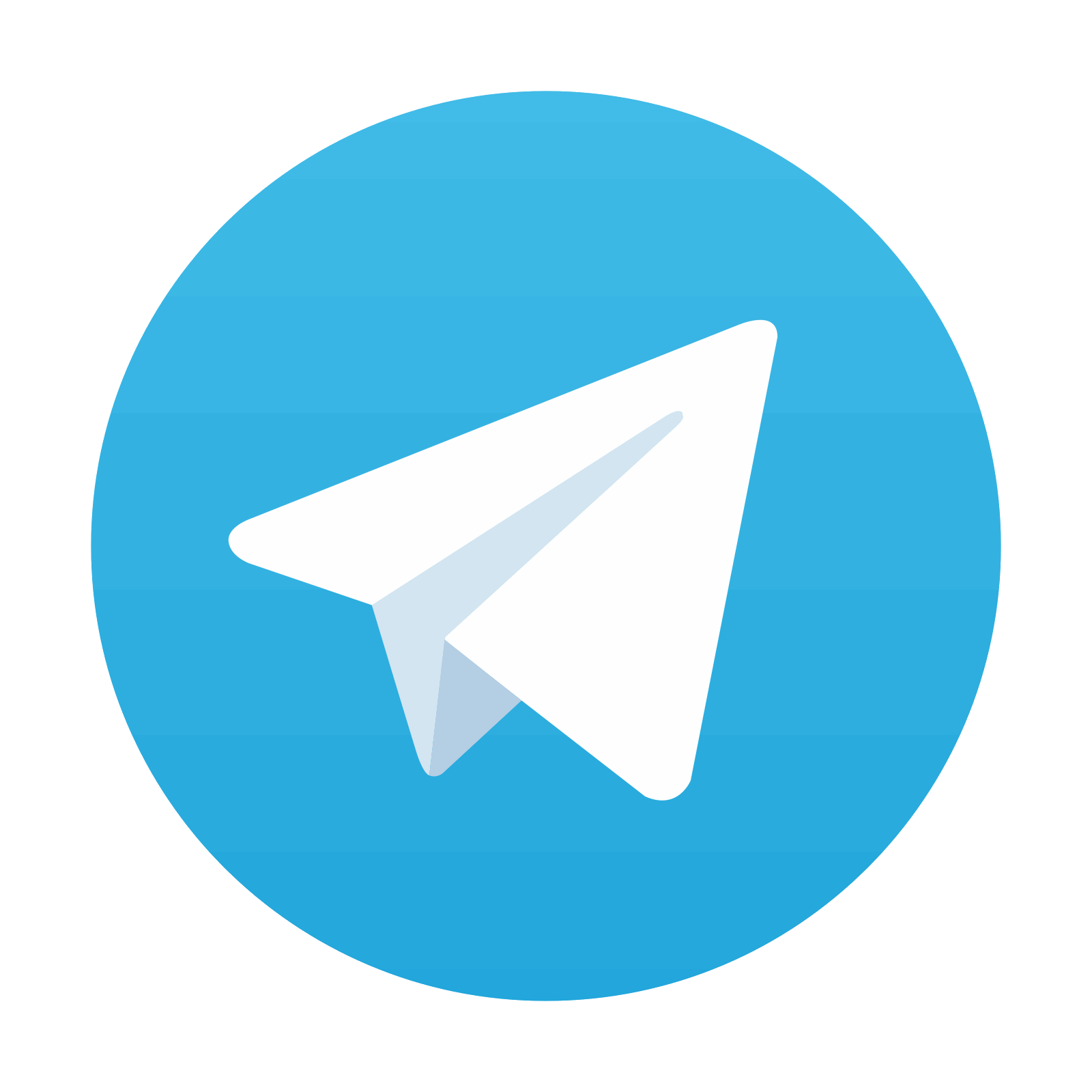
Stay updated, free articles. Join our Telegram channel

Full access? Get Clinical Tree
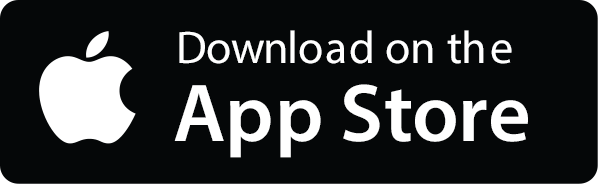
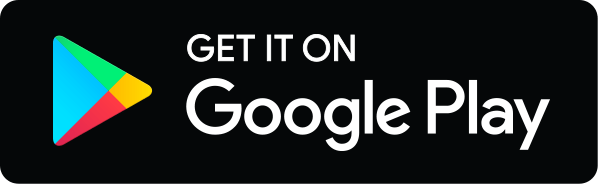
