Introduction
Patients may be admitted to the specialist cardiothoracic critical care unit from a variety of sources (Figure 1.1). In all elective admissions, and in the majority of emergency admissions, a clinical history will already have been elicited and a physical examination performed – often more than once. Most patients will already have undergone extensive investigation or therapeutic intervention, and the underlying diagnosis or diagnoses will have been established. Despite this seemingly ideal situation, the cardiothoracic intensivist should adopt an inquisitive attitude and use the so-called ‘history and physical examination’ to confirm previous findings, assess disease progression and exclude new pathology. Contrary to popular belief, this is often the most efficient and effective means of predicting and detecting significant comorbid conditions. Clinical investigations should therefore be considered an adjunct to, rather than a substitute for, basic medical assessment.
Figure 1.1 Cardiothoracic critical care admission sources.
In the critical care setting, particularly when a patient is physiologically unstable or has reduced consciousness, the conventional stepwise approach to the history and physical examination will usually require modification (Table 1.1). Indeed it may have to be conducted during or after initial resuscitation.
Table 1.1 Modification of conventional history and physical for use in critical care
Conventional | Critical care | |
---|---|---|
History |
|
|
Physical |
|
|
History
The Conscious Patient
All available sources of information should be drawn upon to construct as detailed a history as possible. Where the patient is conscious and able to respond to direct questioning, this important primary source of information should not be overlooked. Rather than using ‘open’ questions and expecting them to recount their entire current and past medical history in a concise fashion and in chronological order, it is often easier to ask the patient to confirm previously documented information and append newly acquired information as necessary. When faced with an acutely unwell and possibly deteriorating patient, the skilled intensivist needs to be able to quickly gather sufficient information to aid diagnosis and guide management. Of particular importance is the patient’s understanding of their medical condition, their insight into treatment options and prognosis, and their expectations. Corroborative history from family and carers is also invaluable, especially in the setting of acute delirium or dementia, where the patient’s own account may be unreliable. This information should be solicited and documented whenever possible.
Symptoms of cardiorespiratory disease (e.g. angina pectoris, dyspnoea, orthopnoea, syncope, palpitations, ankle swelling, etc.) (Table 1.2) should be actively sought, as should any recent progression in symptom severity. Symptoms should be described in terms of their nature (using the patient’s own words), onset, duration, progression, modifying factors and associations. The impact of symptoms on functional status should be documented using the New York Heart Association (NYHA) classification and the Canadian Cardiovascular Society (CCS) angina scale.
Table 1.2 Common symptoms associated with cardiorespiratory conditions
Cardiovascular | Respiratory |
---|---|
Syncope | Recent overseas travel |
Chest pain | Fever and/or rigors |
Fatigue or exercise intolerance | Facial or sinus pain |
Exertional dyspnoea | Chest pain |
Paroxysmal nocturnal dyspnoea | Cough |
Orthopnoea | Sputum production (volume, time course, purulence) |
Palpitations | Haemoptysis |
Intermittent claudication or ischaemic rest pain | Dyspnoea |
Stroke or transient ischaemic attack | Exercise intolerance |
Cough or sputum production | History of bird keeping, asbestos exposure, or other sources of occupational lung disease |
Peripheral oedema |
Enquiry into the patient’s past medical history should include coexisting conditions, previous hospital admissions, surgical procedures and complications, prolonged hospitalisation and unplanned admissions to a critical care unit. It is important to note the indication for any surgical procedure or therapeutic intervention (e.g. splenectomy, permanent pacemaker, angioplasty), the outcome of the procedure and any anaesthetic related morbidity. A history of difficult tracheal intubation is of particular note, both with respect to the unintubated patient who may require intervention during their stay, and the patient who is already intubated who will require extubation before discharge to the ward. Factors known to be associated with increased mortality and morbidity (e.g. congestive cardiac failure, peripheral vascular disease, renal insufficiency, arterial hypertension, pulmonary hypertension, diabetes mellitus, chronic pulmonary disease, neurological disease and previous cardiovascular surgery) should be documented.
Where the patient has been admitted following a diagnostic or therapeutic intervention (e.g. coronary angiography or angioplasty), a comprehensive medical and nursing ‘handover’ is essential. This is particularly important when the patient has been brought to hospital by emergency ambulance and taken directly to the angiography suite. Similarly, when a patient is transferred from another hospital for specialist cardiothoracic care (e.g. surgical repair of acute type A aortic dissection), a formal handover of clinical information and documentation is an absolute prerequisite for the transfer of clinical responsibility and for safe ongoing care. In many areas a formal handover document or aide memoire is used both to guide and to document the comprehensive handover of clinically relevant information.
It is essential to record current and recent prescription drug administration, including formulation, dosage and route of administration. In addition, the medication history should include drugs taken ‘as required’, proprietary or ‘over-the-counter’ medicines, complimentary or alternative therapies, and recreational drugs. This latter category should include alcohol and tobacco products. A history of allergic or other idiosyncratic reaction to a specific drug (e.g. suxamethonium) or class of drugs (e.g. penicillins) should be sought and documented.
Where adherence to a particular cultural or religious belief system (e.g. Jehovah’s Witnesses) has the potential to influence any aspect of critical care management, this should be comprehensively documented. In some instances it may be appropriate to explore and document a patient’s specific wishes in a number of hypothetical clinical scenarios, including limits of care. It is often preferable that limits of care be discussed with the patient and family early on in the critical care stay, rather than late in the course of the illness when the patient is in extremis. It is important that both the patient and the family have a realistic understanding of what intensive care can offer, rather than relying on preconceived ideas.
The Unconscious Patient
The unconscious, critically unwell patient represents a special challenge for any clinician. From a cardiothoracic point of view, such patients cover a wide range of potential presentations, including, but not limited to the following:
A patient transferred from the operating theatre or catheter laboratory following an invasive procedure;
A patient admitted following out-of-hospital cardiac arrest, via either the catheter laboratory or the emergency department;
A patient requiring ongoing organ support following an interventional cardiology or bronchoscopic intervention; and
A ward patient who has physiologically deteriorated and requires more advanced treatment modalities or resuscitation.
When reviewing an obtunded patient the clinician is deprived of many of the usual visual and auditory clues that guide patient assessment, forcing the use of alternative sources of information. Family members and carers are often the key source of information regarding recent symptoms, and it is often possible to establish the temporal course of the presenting complaint with thorough questioning. In many respects, it is often possible to obtain a full history, provided that the right questions are asked, and an open mind maintained.
A thorough review of the medical record is also invaluable when the patient is not able to speak for him or herself. Written correspondence from other clinicians (e.g. surgeons, cardiologists, respiratory physicians, general practitioners) will answer many questions regarding the course of illness leading up to admission. Where questions remain unanswered regarding the previous clinical course, direct communication with these sources is encouraged, not only to obtain further information, but as a matter of courtesy regarding the condition of their patient.
Physical Examination
The Conscious Patient
Whilst a comprehensive physical examination is sometimes not possible given the limitations that reception and resuscitation of the critically ill patient places on assessment, a full physical examination should nevertheless be attempted. There is also a frequent temptation for the clinician to rely on the battery of monitors that an intensive care admission entails, especially during daily review of the patient, or to perform an investigation rather than seek clinical findings. This is a fallacy, as even an abbreviated physical examination during a ward round may reveal a finding (e.g. bronchial breathing) that may take hours to manifest as worsening hypoxia or increasing oxygen requirement, permitting early investigation and intervention.
General Inspection
This should initially be undertaken from the end of the bed, so as to better appreciate the overall Gestalt. The initial focus should be on the patient. Central or peripheral cyanosis may be evident in the setting of hypoxaemia or shunting. The patient posture provides many important clues, especially when assessing respiratory effort. Pursed lip breathing to increase end-expiratory pressure and a ‘tripod’ position with the shoulders rotated forward and the hands on the lower extremity to engage the accessory muscles are evidence of respiratory distress. Attention should then be turned to the various drug infusions being administered, the relevant concentration, rate and route of administration. Peripheral and central venous access should be noted and recorded including available lumens for other medications and the size of each catheter if the administration of volume is required. Invasive monitoring (e.g. arterial line, pulmonary artery catheter), circulatory support devices (intra-aortic balloon pump, ventricular assist device and extracorporeal circuits) and renal replacement therapy should also be evaluated. An indwelling urinary catheter may be present, and if so, the volume and concentration of urine in the drainage bag should be noted.
The postoperative cardiac or thoracic surgical patient will have a variable number of mediastinal and pleural drains in situ. The volume of blood in these should be recorded so that an accurate estimation of any ongoing blood loss can be made. An air leak may also be present when pleural drains are on suction, and the magnitude and respiratory phase of this should be judged. Where epicardial pacing wires are present, their function should be confirmed. If in use, external pacing should be converted from fixed rate mode to demand mode, with an appropriate backup rate.
The Hands and Arms
Examination of the hands reveals much about the circulatory state of the patient. Cold and shut down extremities with delayed capillary return may suggest a high degree of systemic vascular resistance, usually because of hypovolaemia or low cardiac output state, or alternatively, an acutely ischaemic limb. In contrast, warm peripheries suggest a normal or high cardiac output state. Finger clubbing may be indicative of chronic cardiorespiratory disease, notably congenital, cyanotic heart disease, non-small-cell lung cancer and suppurative lung conditions such as cystic fibrosis or bronchiectasis.
The peripheral pulses can give clues to the presence of significant valvulopathy (e.g. the ‘water hammer’ pulse of severe aortic regurgitation) and regional perfusion abnormalities, particularly in aortic dissection (i.e. radioradial and radiofemoral delay). Inspection of the palmar creases was popularised for the estimation of plasma haemoglobin concentration, but has subsequently proven to be unreliable. Rarely, the immunological and embolic phenomena of infective endocarditis (Janeway lesions and Osler’s nodes) may be evident.
The Neck
Neck examination in the critical care environment is often difficult, due to the presence of indwelling jugular venous catheters. In the event that the neck is unencumbered, examination of the jugular venous waveform can be used to assess right atrial filling and compliance, atrioventricular dissociation (cannon a-waves) and torrential tricuspid regurgitation (massive cv-waves). These abnormalities are also visible on the central venous waveform if invasive monitoring is present.
If pericardial tamponade is suspected, an early sign of compromise is an increase in right atrial pressure on deep inspiration (Kussmaul’s sign). If the patient’s trachea is not intubated, it is wise to conduct an airway assessment at this juncture if not already performed. Auscultation of the carotid arteries may reveal bruits consistent with turbulent flow if there is a substantial atheroma burden, or the referred murmur of aortic stenosis.
The Praecordium
As for other parts of the body, and as alluded to in the starting quotation, examination of the chest should follow the traditional route of observation, palpation, percussion and auscultation. Ideally, both the anterior and posterior chest should be examined, as the lower lobes of the lung (particularly on the left) can take up much of the posterior aspect, preventing examination of the other lobes if the anterior chest is not examined. The often high volume of ambient noise in the critical care environment may make ausculation challenging. Subtle abnormalities may be missed, and seemingly positive findings may be misinterpreted. It is wise to correlate findings not consistent with the overall condition of the patient with appropriate investigation. Radiological investigation and bedside modalities such as ultrasound and transthoracic echocardiography are valuable for this purpose.
The Abdomen
Initially, at least, the abdomen is rarely a focus in the cardiothoracic critical care unit. Interest in this region is limited largely to distension and the presence of bowel sounds or the absence thereof. However, small or large bowel ischaemia is a not uncommon phenomenon following cardiac surgery, as a result of a low cardiac output state, embolic phenomenon or use of intra-aortic balloon counterpulsation. A high index of suspicion is required for this condition, particularly in the setting of an unexplained lactataemia and worsening acidosis, despite the presence of a seemingly adequate cardiac output. The opportunity should be taken at this point to assess the back of the patient for sacral oedema, as this is the most dependent point in the semirecumbent patient, and is an important finding when assessing volume status.
The Legs
An assessment of the legs completes the examination. Like the hands, the lower extremities reveal much about perfusion status, thus capillary refill and skin temperature should be assessed. The posterior tibial and dorsalis pedis pulses should be sought, particularly if the femoral vessels have been used for arterial access. Whilst a rare event, acute lower limb ischaemia is a recognised complication of a wide range of invasive devices, in particular peripheral venoarterial ECMO cannulae and the intra-aortic balloon pump. It is important to note that even if a distal reperfusion line is incorporated into an ECMO circuit, distal pulses may be absent. However, the limb should feel warm and well perfused or, at the very least, similar to its counterpart.
Deep venous thrombosis is a common complication in many postoperative patients, and whilst at least half of these are completely asymptomatic, the remainder may exhibit the classical signs of calf tenderness, swelling, distended superficial veins and warmth. The traditional test for this condition, Homan’s sign (rapid passive dorsiflexion of the ankle with the aim of causing pain in the calf), is no longer recommended because of the risk of clot fragmentation and acute pulmonary embolism.
Finally, the presence and extent of any peripheral oedema should be assessed. Oedema is, by definition, an excess of interstitial fluid, and therefore must be the result of a derangement of the Starling forces across the microcirculation (increased capillary hydrostatic pressure, decreased plasma colloid oncotic pressure, increased capillary permeability or deranged lymphatic drainage).
The Unconscious Patient
As for history, the physical examination of the unconscious individual is hindered by the lack of patient participation. Nevertheless, such an examination should always be undertaken with the same care as if the patient were awake and fully conscious. The presence of the mechanical ventilator at the bedside increases the ambient noise level, further impairing the ability of the clinician to auscultate. Heavy sedation or neuromuscular blockade naturally prevents patient movement, and simple tasks such as leaning the patient forward to auscultate the chest are impossible. Nevertheless, an attempt should be made, as much valuable information can still be gained, particularly with respect to tissue perfusion.
As for physical examination in the conscious patient, assessment of the unconscious patient should follow in the same stepwise fashion. Many of the same clinical features may be found on careful inspection. Caution should be taken during joint manipulation and palpation. The patient will not be able to report discomfort or resist painful movements, and tissue damage may result if the examiner is overly rough. Examination of the abdomen in the unconscious patient is considerably confounded, especially in the setting of neuromuscular blockade. This is because the early features of gut ischaemia or peritonism will be absent, partly because the patient is unable to report discomfort, but also because of a lack of abdominal muscle tone. As a result, the early features of guarding and tenderness to percussion (formerly tested as ‘rebound tenderness’) are absent. Consequently, abdominal distension, free gas under the diaphragm and a rising lactate in the setting of apparently adequate cardiac output may be the only features of a major intra-abdominal pathology. A high index of suspicion must be maintained as a result.
Daily assessment of the unconscious patient should also include a brief neurological examination. Naturally, a full assessment of muscle power cannot be undertaken, but a brief examination for features of an upper motor neurone lesion such as hypertonia, hyperreflexia and clonus (in the absence of neuromuscular blockade) can be quickly and easily performed. As described in detail in Chapter 16, on sedation and analgesia, the indications for deep sedation and neuromuscular blockade are becoming fewer as cardiothoracic critical care evolves, and targeted sedation and sedation breaks are increasingly de rigueur. Conscious state can be graded with any number of specialist sedation scores, which are beyond the scope of this chapter – however, response to approach, voice and pain should be assessed. A variety of different techniques have been described to evaluate response to painful stimulus. However, firm pressure over the superior orbital notch is usually the most unambiguous means of assessing global response, as peripheral stimulation may not give an accurate assessment of localisation if a hemiplegia is present. Likewise, withdrawal to stimulation, decerebrate and decorticate posturing can be difficult to assess when the focus point is on the hand or foot.
Conclusions
The presence of advanced monitoring modalities and ready access to bedside investigations, combined with difficult examination conditions, are all powerful motivators to de-emphasise the traditional focus on history and examination. Whilst cardiothoracic critical care requires a different skill set to that of the emergency department or ward, the temptation to forgo the basic diagnostic process must be resisted, as history and examination findings serve to clarify the clinical scenario and highlight evolving problems that monitoring may not detect for some time. Furthermore, history and physical examination allow appropriate targeting of investigations, minimising patient discomfort and unnecessary cost to the health system.
Learning Points
The cardiothoracic intensivist should adopt an inquisitive approach to history and physical examination so as to confirm previous findings, assess disease progression and exclude new pathology.
It can be challenging to elicit a history and undertake a physical examination in the critically ill patient and it may be necessary to modify the conventional stepwise approach.
Advanced monitoring modalities are prone to artefact and incorrect interpretation and should not be seen as a substitute for a thorough history and physical examination.
On admission/discharge from cardiothoracic critical care a formal handover of clinical information is an absolute prerequisite for continuity of care.
It may be necessary to explore and document a patient’s specific wishes in a number of hypothetical clinical scenarios so as to inform decision making should the patient deteriorate.
Further Reading
Introduction
Electrocardiography (ECG) has been the main heart investigation method for most of the twentieth century and still plays a pivotal role in diagnostics. ECGs can provide a wealth of information about cardiac function and can often show early signs of systemic abnormalities as well. In order to accurately interpret an ECG it is important to first ensure its correct acquisition. The process involves recording small electrical changes on the skin that occur as a result of cardiac muscle depolarisation. Ten electrodes are used to record the heart’s electrical activity in 12 different orientations, which encompass the 12 ‘leads’ of the ECG. It is important therefore to ensure that these 10 electrodes are consistently applied in order to accurately assess for any abnormalities. In addition, account must be made of sources of interference.
Recording the ECG
As posture may affect the appearance of the ECG it is preferably performed in the supine position where practicable. Skin preparation is important to reduce artefacts and may include hair removal and/or skin cleansing.
Limb Electrodes
Moving the limb electrodes away from the distal limbs may affect the ECG appearance and it is therefore preferable that these are placed just proximal to the wrists and ankles in order to produce consistent results. Limb electrodes are often colour coded to aid placement.
Right arm (RA, red) proximal to right wrist
Left arm (LA, yellow) proximal to left wrist
Left leg (LL, green) proximal to left ankle
Right leg (RL, black) proximal to right ankle
Precordial (Chest) Electrodes
Most errors occur in placement of the chest electrodes, especially V1 and V2, which may be placed too high. This can have a significant effect on the resultant ECG. Correct anatomical positioning must be adhered to, with the centre of the electrode aligned with the correct location.
V1 (C1) fourth intercostal space, right sternal edge
V2 (C2) fourth intercostal space, left sternal edge
V3 (C3) midway between V2 and V4
V4 (C4) fifth intercostal space, midclavicular line
V5 (C5) horizontal level with V4, anterior axillary line
V6 (C6) horizontal level with V4, midaxillary line
After placement of V1 and V2, V4 is located in the fifth intercostal space, midclavicular line. V3 is then placed directly in between V2 and V4. V5 and V6 are located at the same horizontal level as V4, perpendicular to the midclavicular line.
Filter Settings and Calibration
Most filter settings are set by default but it is recommended that the low frequency filter is set on or below 0.05 Hz. This filter will account for respiration. If it is set too high, it will distort the ST segment. This may also reduce the accuracy of detecting myocardial ischaemia based on ST segment shifts. The high filter setting should be set on or above 100 Hz. This filter setting should account for the artefact created by muscle tremor. The mains filter (50 Hz) is normally set to ‘Off’.
A standard ECG has a voltage calibration of 10 mm/mV and is recorded with a paper speed of 25 mm/s. This results in 10 second recording. Each small square is equal to 40 ms and each large square (5 small squares) is equal to 200 ms.
The 12 ‘Lead’ ECG
The 12 ‘leads’ of an ECG correspond to 12 vectors along which depolarisation of cardiac tissue is recorded. Each is created by measuring the electrical potential between two points. In each case one of the ten electrodes is the positive pole. There are three bipolar limb leads where another electrode is the negative pole. The negative pole for the unipolar limb leads and the precordial leads is a composite pole (VW) called Wilson’s central terminus, which is created by averaging the potential recorded by RA, LA and LL electrodes:
VW = 1/3 (RA + LA + LL).
The six limb leads view the heart in the coronal (vertical) plane while the six precordial leads view the heart in a perpendicular transverse (horizontal) plane.
Bipolar Limb Leads
Unipolar Augmented Limb Leads
Lead aVR is the potential difference between RA and Vw,
aVR = 3/2 (RA – VW).
Lead aVL is the potential difference between LA and Vw,
aVL = 3/2 (LA – VW).
Lead aVF is the potential difference between LL and Vw,
aVF = 3/2 (LL – VW).
The vectors created by the bipolar and augmented limb leads together form the hexaxial reference system (see Figure 2.1).
Figure 2.1 Hexaxial reference system.
Precordial Leads
For each of the precordial leads the positive pole is the corresponding electrode and the negative pole is VW.
ECG Arrangement
A standard ECG records a 2.5 second tracing of each lead arranged in a grid of four columns and three rows. A marker depicts the change from one lead to the next in each row and can be confused with part of the ECG. The first column contains leads I, II and III, the second column aVR, aVL and aVF, the third column V1, V2 and V3 and the final column contains leads V4, V5 and V6. A fourth row is often provided as a continuous tracing to aid determination of rhythm.
Although each lead records electrical activity of the heart from a different angle, contiguous leads are associated with different anatomical regions.
Inferior leads II, III and aVF
Anterior leads V3 and V4
Septal leads V1 and V2
Lateral leads I, aVL, V5 and V6
Interpretation of the ECG
Waves and Intervals
P Wave
The P wave (see Figure 2.2) represents depolarisation of the right and left atria. In normal sinus rhythm, its origins are from the sinus node, which is normally located posteriorly high in the right atrium. Therefore, the P wave is positive (upright) in all leads except aVR, and can be positive or biphasic in V1. It typically has duration <80 ms.
In right atrial enlargement, the P wave is tall and peaked, and is prolonged and bifid in left atrial enlargement.
PR Interval
The PR interval is measured from the beginning of the P wave to the beginning of the QRS complex and represents the time taken for atrial depolarisation and conduction through the AV node. A normal PR interval varies between 120 and 200 ms.
If the PR interval is shorter than 120 ms then conduction is bypassing the AV node (see Wolf–Parkinson–White syndrome). Prolongation of the PR interval indicates 1° AV block. Depression of the short isoelectric segment between the end of the P wave and the beginning of the QRS complex can indicate pericarditis.
QRS Complex
Q, R and S waves form the QRS complex, which represents ventricular depolarisation. If the first deflection is negative it is termed the Q wave, otherwise an initial positive deflection is an R wave. A final negative deflection is the S wave. Normally the whole QRS complex is <120 ms in duration. Q waves represent left to right depolarisation of the interventricular septum and are typically seen in left sided leads (I, aVL, V5 and V6). Small Q waves can be seen in most leads except V1, V2 and V3.
Large Q waves are pathological if >40 ms wide, and >2 mm deep or >25% of the height of the QRS complex. This can represent myocardial infarction, cardiomyopathy or electrode malposition.
If the QRS complex is particularly tall this may indicate left ventricular hypertrophy. A number of criteria have been developed to aid diagnosis, none of which are perfect. Most commonly used is the Sokolow–Lyon index:
S wave in V1 + R wave in V5 or V6 (whichever largest) > 3.5 mV.
There is a large S wave in lead V1, which gradually becomes smaller (absent) by lead V6. Its presence or absence is rarely of clinical significance.
J Point
The J point is the junction between the QRS complex and the subsequent ST segment. Elevation or abnormalities of the J point are frequently seen and their significance is debated. Elevation of the J point is observed in hypothermia.
ST Segment
The ST segment is the section between the end of the QRS complex (J point) and the T wave. It is typically isoelectric and represents the period of time when the ventricles remain depolarised prior to repolarisation. If the ST segment is down sloping or depressed, this may indicate myocardial ischaemia. ST segment elevation, taken as more than 1 mm, 80 ms following the J point, may indicate myocardial infarction but has a false positive rate between 15 and 20% (even slightly higher in women). Abnormalities of the ST segment can also occur in pericarditis and left ventricular hypertrophy.
T Wave
The T wave represents ventricular repolarisation. It is typically positive in all leads except aVR and V1. A variety of situations can result in T wave abnormalities, which are often non-specific. Myocardial ischaemia and left ventricular hypertrophy can result in T wave inversion. Metabolic abnormalities can also manifest as T wave abnormalities.
U Wave
The U wave is poorly understood but may represent repolarisation of the interventricular septum or papillary muscles and therefore can be observed on the normal ECG, although their absence is not pathological. Prominent U waves are observed in hypokalaemia and hypothyroidism.
QT Interval
The QT interval is measured from the beginning of the QRS complex to the end of the T wave. There are several issues regarding its measurement, not least determining where the T wave ends. The best lead to measure the QT interval is lead V3 or II. Several consecutive beats should be measured with the longest value taken. Prominent U waves are not normally included in the calculation and the end of the T wave is then assumed to be where the downslope of the T wave would cross the isoelectric line. If depolarisation is prolonged, the difference between the measured QRS complex and 120 ms should be subtracted from the measured QT interval.
Because of the close relationship of the QT interval and heart rate (the QT shortens with increasing heart rate), a correction factor must be applied. Although many methods have been developed to calculate a corrected QT (QTc), the one most widely used is Bazett’s formula:
QTc = QT / √RR interval.
The RR interval is measured in seconds such that at a heart rate of 60 bpm the RR interval is 1, and QTc = QT. The QTc is typically less than 440 ms. Gender, however, also has an effect on QTc and therefore a value of less than 460 ms is acceptable for women.
An abnormally prolonged QTc can predispose to malignant ventricular arrhythmias. A long QT can be the result of a genetic abnormality or due to a variety of medications.
Figure 2.2 PQRST intervals.
Rate and Rhythm
Heart Rate
The heart rate is most accurately obtained by dividing the cycle length (RR interval) into 60,000. However, this is more easily achieved by dividing the number of large squares on a standard ECG between successive QRS complexes into 300. These formulae work well provided the rhythm is regular. However, if the rhythm is irregular then we can take advantage of the fact that the ECG is recorded over 10 seconds. Counting the number of QRS complexes across the ECG and multiplying by 6 will give an estimate of the heart rate.
Rhythm
The presence of P waves on their own does not indicate sinus rhythm. It is important to ensure that their morphology (as discussed earlier) is consistent with an origin from the sinus node. A disparate morphology might indicate an ectopic atrial origin and prompt closer scrutiny. If the P wave is not clear on the surface ECG then, post cardiac surgery, the temporary atrial pacemaker wires can be used to create an atrial electrocardiogram (AEG). This is recorded by connecting the atrial pacing wires to the left and right arm leads, or to one of the precordial chest leads, and may be useful in differentiating various tachy and brady arrhythmias.
Axis and QRS Transition
The mean frontal axis (Figure 2.3) of the heart provides an average electrical axis (vector) of ventricular depolarisation. Any deviation from a normal axis may indicate pathology. This may be in the form of structural abnormalities (i.e. increased muscle mass – left ventricular hypertrophy or loss of muscle mass – following myocardial infarction) or electrical abnormalities (i.e. accessory AV connections or bundle branch block). A normal QRS axis is usually between −30° and +90°.
The mean frontal axis provides a mean electrical ventricular depolarisation vector in two dimensions only. The QRS transition provides an indication of the ventricular depolarisation in the third dimension (z axis). The QRS transition is determined from the precordial leads. The QRS complex in lead V1 is usually negative and gradually becomes more positive through V6. The transition zone is where the QRS changes from being predominantly negative to being predominantly positive. This usually occurs at V3 or V4.
Calculating the QRS Axis
To estimate the QRS axis we focus solely on the limb leads (not V1–V6) and use the fact that depolarisation towards any lead will provide a positive deflection in that lead.
If lead I is positive, the axis must lie between −90° and +90°. If lead II is negative, then the axis is between −30° and −240°. Together the axis must be between −30° and −90°. This is left axis deviation.
If lead I is negative, the axis must lie between −90° and −270°. If lead aVF is positive, the axis must be between 0° and 180°. Together the axis must be between −90° and 180°. This is right axis deviation.
If lead I is negative and lead aVF is negative, then the axis lies between −90° and 180°. This is an indeterminate axis.
Figure 2.3 Mean frontal axis.
Arrhythmias
Conduction Abnormalities
Bundle Branch Block
Electrical impulses transmitted through the AV node and bundle of His are conducted to the ventricular myocytes via the left and right bundles.
The right bundle remains sheathed in connective tissue within the septum until it reaches the base of the papillary muscle when it divides into multiple fibres connecting with the Purkinje fibres. The left bundle however divides immediately into anterior and posterior fascicles supplying Purkinje fibres within the left ventricle.
Right bundle branch block (RBBB) occurs when there is loss of conduction through the right bundle branch. The left ventricle is excited normally by the left bundle branch but the right ventricle is depolarised via conduction through the myocardium from the left ventricle. As a result, ventricular depolarisation is prolonged and there is an extra deflection indicated by rapid left ventricular depolarisation followed by slower right ventricular depolarisation. A QRS duration >100 ms indicates incomplete block and a duration >120 ms indicates complete block. A terminal R wave is observed in lead V1 (rsRʹ) and a slurred S wave in leads I and V6. The T wave should be deflected opposite the terminal deflection of the QRS complex. Right bundle branch block can be observed in normal individuals without cardiac disease.
Left bundle branch block (LBBB) is demonstrated by a QRS >120 ms, a QS or rS complex in lead V1 and a notched R wave in lead V6. Involvement of only the left anterior fascicle results in left axis deviation and less marked widening of the QRS complex. This is called left anterior hemiblock (LAHB). A qR complex is observed in lateral leads I and aVL, and rS pattern in the inferior leads II, III and aVF. Due to its broad nature and dual blood supply, left posterior hemiblock (LPHB) is much less common. It is characterised by right axis deviation, a rS complex in lateral leads and qR complex in inferior leads.
AV Block
When conduction between the atria and ventricles is impaired, AV block is said to be present. If the PR interval is prolonged beyond 200 ms but each P wave remains associated with a single QRS complex, 1° AV block is present.
Progressive beat-to-beat prolongation of the PR interval with the final beat not conducting to the ventricles is Mobitz 1 2° AV block (Wenckebach phenomenon). However, if the PR interval remains unchanged prior to loss of conduction to the ventricles then Mobitz 2 2° AV block is present.
When there is complete dissociation between atrial and ventricular depolarisation (i.e. no association between P waves and QRS complexes) 3° AV block is present.
Tachyarrhythmias
Supraventricular Arrhythmias
Atrial flutter: Atrial flutter refers to any macro re-entrant rhythm within the atria. Typical atrial flutter is a right atrial rhythm where depolarisation occurs continuously, usually in a counterclockwise fashion, around the tricuspid valve. The AV node will conduct at a variable rate depending on other factors such as concomitant medication etc. The resultant ECG demonstrates a continuously cycling baseline between QRS complexes. These ‘flutter’ waves have a slow phase and a fast phase, are negative in the inferior leads, positive in lead V1 and isoelectric in lead I which is perpendicular to the re-entrant circuit.
Atrial fibrillation (AF): Although theories regarding the exact mechanisms responsible for atrial fibrillation abound, it is still poorly understood. In essence, however, there are multiple re-entrant circuits and wavelets that are constantly colliding and interrupting each other. The AV node is continuously receiving impulse, which again will be conducted at a variable rate. The ECG will demonstrate a chaotic baseline, constantly changing in frequency and amplitude.
AV node re-entrant tachycardia (AVNRT): The AV node receives multiple inputs within the transition zone around the compact AV node. These inputs may have different properties with regards to conduction velocities and refractory periods. This discordance can result in a re-entrant rhythm between the different connections. The ECG depicts a regular narrow complex tachycardia with no discernable P waves as both atria and ventricles are depolarised simultaneously.
AV re-entrant tachycardia (AVRT): Additional accessory electrical connections between the atria and ventricles can result in pre-excitation. The relatively quick conduction over the accessory connection results in the ventricle beginning to depolarise from an area other than those directly supplied by the bundle branches. This ventricular depolarisation is fused with depolarisation from the normal conduction system as signals catch up through the AV node. The slurred onset of the resultant QRS complex is termed a ‘delta’ wave and represents pre-excitation.
Under certain circumstances, impulse can continuously cycle between the AV node and accessory connection. If the AV node is the anterograde limb of the circuit, the resultant arrhythmia is orthodromic AVRT. Rarely, the accessory connection is the anterograde limb and the resultant arrhythmia is antidromic AVRT. The ECG of orthodromic AVRT will show a narrow complex tachycardia (no pre-excitation evident) with inverted P wave discernible shortly after the QRS complex. Antidromic AVRT will show a maximally pre-excited QRS complex.
Subjects with pre-excitation and symptoms that may be related to it (palpitations, breathlessness, presyncope and syncope) are said to have Wolff–Parkinson–White syndrome (WPW).
Ventricular Arrhythmias
A broad complex tachycardia is ventricular tachycardia (VT) until proved otherwise. Patients should be assessed and treated promptly within current guidelines. Distinguishing between ventricular tachycardia and supraventricular tachycardia with aberrant ventricular depolarisation can be difficult. Clearly dissociated P waves, fusion beats (partial ventricular depolarisation over the AV node) and capture beats (complete ventricular depolarisation over the AV node) are all good indicators of VT but are frequently absent. Other features, which are suggestive of VT are:
Learning Points
Accurate electrode placement is essential to ECG interpretation.
Care should be taken to examine all leads when making measurements.
QRS axis and transition can be used together to indicate underlying ventricular abnormalities.
Narrow complex tachycardias can often be distinguished by their ECG features.
Broad complex tachycardias should be considered to be ventricular tachycardia until proved otherwise.
Further Reading
MCQs
1. Electrode V2 is positioned at the following position:
2. The QTc attempts to correct the QT interval to a heart rate of:
3. A normal QRS axis lies between:
4. A gradual beat-to-beat lengthening of the PR interval followed by a dropped beat is known as:
5. An ECG with a continuously cycling regular baseline between QRS complexes is likely to be showing:
Introduction
Since its introduction into the intensive care environment in the early 1980s, echocardiography has been recognised as an invaluable tool. Transthoracic echocardiography (TTE) provides a non-invasive, portable imaging modality, which allows for rapid diagnosis and cardiovascular monitoring in the critically ill. Transoesophageal echocardiography (TOE) produces better resolution images and is often utilised as an adjunct, or when TTE image quality is inadequate.
Focused echocardiography provides a goal directed ultrasound examination to address specific diagnostic and monitoring questions.
Indications for Echocardiography
In the critically ill patient echocardiography has been shown to provide supplemental information to physical examination and other monitoring modalities. It may be performed for diagnostic reasons, as a haemodynamic monitor, to assess volaemic status, or for procedural guidance.
Focused Scanning
A focused TTE evaluation comprises four views of the heart: parasternal long-axis and short-axis, apical four-chamber and subcostal views (Figure 3.1). Each view provides basic information on biventricular function, volaemic status, valvular function, and the presence of pleural or pericardial collections. When integrated with clinical examination and other haemodynamic parameters, point-of-care echocardiography provides a bedside tool for diagnosis and monitoring of cardiovascular pathophysiology. Multiple focused cardiac scanning protocols are now in use across the globe (e.g. FICE, FEEL, FATE) and extended algorithms have been developed to allow basic assessment of valvular pathology and quantification of ventricular function. Focused scanning requires not only the development of skills to obtain adequate ultrasound images, but also the knowledge to interpret the findings and the experience to request a comprehensive study when indicated. Consequently, training and accreditation programmes are now established to ensure competency and focused echo is a skill to be possessed by all critical care physicians.
Figure 3.1 Basic focused transthoracic echocardiography views. PLAX, parasternal long-axis; PSAX, parasternal short-axis; A4C, apical four-chamber; SC, subcostal.
Left Ventricle
Left ventricular (LV) systolic function (LVSF) is frequently altered in the critically ill, either as a cause of decompensation, or as a consequence of critical illness: the incidence of LVSF in septic shock, for example, may be as high as 60%.
Advances in perioperative management and myocardial protection have seen a reduction in the incidence of postcardiotomy cardiogenic shock, but it may still occur in up to 6% of cardiac surgical procedures, and is associated with high mortality. Early postoperative detection or exclusion of LV dysfunction is paramount to initiate prompt and appropriate therapy.
Although some studies have reported good correlation between qualitative and quantitative assessment, objective quantification of LVSF is recommended and allows for interobserver comparisons.
Fractional shortening (FS), derived from M-mode linear measurements, is a quick and reproducible measure of LVSF. However, it is only representative of a single dimension, and in the presence of regional wall motion abnormalities (RWMA) may give an inaccurate measure of global LVSF.
Ejection fraction (EF) is calculated from estimates of LV end-diastolic volume (LVEDV) and end-systolic volume (LVESV). EF derived from the FS, using the Teichholz method, is limited by its assumptions of the geometric LV shape, and is no longer recommended. The modified Simpson rule involves tracing the LV cavity in the four-chamber and two-chamber views at end-diastole and end-systole to estimate LVEDV and LVESV (Figure 3.2). Three-dimensional echocardiography has also been shown to produce accurate and reproducible measures of LV volumes. EF is calculated as:
EF = (LVEDV – LVESV) / LVEDV.
Figure 3.2 TOE midoesophageal four-chamber (a) and two-chamber (b) views, showing biplane method (modified Simpson’s rule) to derive left ventricular ejection fraction.
The presence of RWMA before and after cardiac surgery is not uncommon, but the detection of new defects warrants further investigation. After coronary artery bypass grafting (CABG) surgery, a new RWMA suggests myocardial ischaemia or possibly infarction from multiple causes including a compromised coronary artery bypass graft occlusion, coronary vasospasm, inadvertent left circumflex artery ligation during mitral valve (MV) surgery (presenting with LV lateral wall akinesia), coronary ostial compromise after aortic root surgery and others. The 17-segment LV model is typically used and each segment is scored as follows:
1 normokinesia, normal regional wall motion
2 hypokinesia, reduced regional wall motion
3 akinesia, no movement of one or more segments
4 dyskinesia, paradoxical movement of one or more segments in relation to other LV areas.
However, RWMA may occur in the absence of significant coronary artery disease: postoperative epicardial pacing induces abnormal motion of the interventricular septum (IVS) and posterior LV; stress induced (Takotsubo) cardiomyopathy classically presents with apical akinesia and ballooning; the inferior and inferolateral LV walls are most often affected in myocarditis.
The assessment of LV diastolic function should form an integral part of a routine examination, especially in patients presenting with heart failure. In fact, up to 50% of patients with CHF have isolated LV diastolic dysfunction (DD) in the presence of a normal LVEF. In addition, LVDD may play an important role in a subset of patients difficult to wean from mechanical ventilation.
Patients with dynamic LV outflow tract (LVOT) obstruction exhibit hypotension, a low cardiac index and high LV filling pressures, but deteriorate with inotropic administration. Typically seen in hypertrophic obstructive cardiomyopathy (HOCM) and post-MV repair surgery, it may also occur in severe hypovolaemia. Echocardiography is indispensable in making the correct diagnosis, allowing expeditious treatment revision to vasopressor therapy, volume loading and cessation of inotropic support. Echocardiography findings include the presence of a significant gradient in the LVOT, systolic anterior motion (SAM) of the MV and mitral regurgitation (MR).
Right Ventricle
Acute right ventricular (RV) failure after cardiac surgery carries a poor prognosis. It occurs frequently after heart transplantation and LV assist device (LVAD) implantation. RV dysfunction is a well-recognised complication of acute respiratory distress syndrome (ARDS) and RV-protective ventilation strategies have emerged to impact on RV function.
In the presence of tricuspid regurgitation (TR), RV systolic pressure can be reliably estimated by applying the simplified Bernoulli equation to the peak TR jet velocity.
Due to its geometry, echocardiographic quantification of RV systolic function is challenging. Longitudinal measures of function, such as tricuspid annular plane systolic excursion (TAPSE) and tissue Doppler derived tricuspid lateral annular systolic velocity (Sʹ), provide excellent measures of RV function. They are easily obtainable by TTE, but with TOE, values can be underestimated due to linear malalignment. Their use in the perioperative setting is less robust: opening of the pericardium is accompanied by a significant decline in TAPSE and Sʹ without an associated decrease in global RV function. This makes preoperative and early postoperative comparisons unreliable. RV fractional area change (FAC) provides an accurate measure of global RV function (Figure 3.3), correlates with RVEF derived from magnetic resonance imaging and is an independent predictor of mortality. Global longitudinal strain and three-dimensional imaging offer alternative measures of RV performance.
Figure 3.3 TOE midoesophageal four-chamber right ventricular views in end-diastole (a) and end-systole (b), used to calculate right ventricular fractional area change.
Valvular Heart Disease
In the early postoperative period, echocardiography is used to assess adequacy of valve repair, competence of valve replacement, detection of significant paravalvular leaks, recognition of patient-prosthesis mismatch, and iatrogenic valve injury.
Valvulopathies responsible for acute deterioration can be postischaemic, infective and traumatic in origin. Acute myocardial infarction (MI) may be complicated by papillary muscle rupture and severe MR. Infective endocarditis (IE) is a life threatening condition. TOE is recommended in patients with high clinical suspicion of IE. It is essential to determine the size and precise location of vegetations, extent of leaflet destruction and valvular dysfunction, the presence of abscess cavities or fistulae, and dehiscence of prosthetic valves (Figure 3.4). Echocardiography findings are crucial in predicting embolic risk and establishing the timing of surgical intervention.
Figure 3.4 TOE midoesophageal long axis view, showing vegetations (arrows) on the aortic valve (AV). LA, left atrium; LV, left ventricle.
Pericardium
Cardiac tamponade is a clinical diagnosis comprising haemodynamic instability associated with equalisation of diastolic filling pressures and large respiratory fluctuation in arterial pressure (pulsus paradoxus). After cardiac surgery, however, classic signs of cardiac tamponade are often mild and atypical presentations are not uncommon. Localised pericardial collections and thrombus formation may cause isolated left-sided compression, with normal right-sided pressures. A high index of suspicion is required postcardiac surgery and TTE or TOE often provides rapid confirmation of diagnosis (Figure 3.5). Loculated and posterior collections may necessitate TOE. In patients with significant pulmonary hypertension, RV diastolic collapse may be absent. In haemodynamically stable patients, echocardiography may be utilised to monitor the progression of a pericardial effusion.
Figure 3.5 TTE subcostal view, showing a large pericardial effusion (arrows). LV, left ventricle; RV, right ventricle.
Constrictive pericarditis (CP) results in impaired diastolic filling due to a rigid, non-compliant pericardium. Patients present with chest pain, dyspnoea and peripheral oedema. Although usually idiopathic in origin, it can occur post-MI and postcardiac surgery. It is important to differentiate it from restrictive cardiomyopathy (RCM).
Echocardiographic features to distinguish CP from RCM include a hyperechogenic pericardium, dynamic changes in LV diastolic filling velocity with respiration and preservation of myocardial relaxation velocities.
Aorta
Aortic dissection typically presents with chest pain and symptomology overlaps that of acute MI, however, it may be asymptomatic perioperatively. Contrast computed tomography (CT) and cardiac magnetic resonance (CMR) usually confirms the diagnosis, but TOE may assist in demonstrating complications, including aortic regurgitation (AR), pericardial effusion, pleural effusion and RWMA secondary to coronary involvement of the dissection flap. In particular, TOE examination of the mechanism of AR is essential to determine the surgical plan.
In the haemodynamically unstable postoperative patient, an echocardiographic study must include assessment of the aorta: the incidence of intraoperative iatrogenic type A dissection is approximately 0.2%.
Intracardiac Shunts
Previously undiagnosed interatrial shunts may present with hypoxaemia or paradoxical emboli, particularly in patients subjected to increased positive airway pressures. Right-to-left shunting via a persistent foramen ovale is observed in up to 20% of patients with ARDS and refractory hypoxaemia.
Post-MI cardiogenic shock may be precipitated by ventricular septal defect that often requires placement of an intra-aortic balloon pump and urgent surgical correction. Echocardiographic examination must exclude this rare but life threatening complication in patients with haemodynamic instability after an MI involving the interventricular septum.
Intracardiac Masses
Cardioembolism accounts for 15–30% of ischaemic strokes. Echocardiography represents the mainstay in evaluation for left-sided cardiac masses. In atrial fibrillation, thrombus typically develops in the left atrial appendage; thrombus also occurs in areas of LV akinaesia or aneurysm, and on pacemaker wires or indwelling catheters in the right side of the heart (Figure 3.6). Other potential embolic sources include tumours and vegetations.
Figure 3.6 TOE midoesophageal bicaval view, showing thrombus (arrows) attached to a central venous catheter (CVC), in the right atrium (RA). LA, left atrium; SVC, superior vena cava.
Pulmonary Embolism
Although CT remains the gold standard for the diagnosis of pulmonary embolism (PE), echocardiography may be of value for the patient too unstable to transfer to the radiology suite. RV dilatation, RV dysfunction (McConnell’s sign), peak TR jet velocity >2.7 m/s and pulmonary artery acceleration time <80 ms all support the diagnosis of PE.
Cardiac Arrest
A focused TTE exam may be of value in the rapid identification of reversible causes of cardiac arrest. To avoid interruptions in chest compressions, a subcostal view is obtained during the 10-second pulse check. Readily detectable reversible causes include hypovolaemia, tamponade, tension pneumothorax, myocardial ischaemia and signs of PE.
Haemodynamic Monitoring
Inferior vena cava (IVC) diameter and its respiratory variation as a marker of fluid responsiveness has been well characterised. Initially described in spontaneously breathing patients, its extension to the ventilated population remains debatable. The effect of positive pressure tidal volumes and pulmonary compliance on IVC variability makes interpretation problematic. Even with spontaneous ventilation, increased work of breathing with the generation of extreme negative intrathoracic pressures IVC collapsibility can be misleading. IVC collapsibility and distensibility indices have been developed to improve reliability in determining fluid responsiveness. The superior vena cava (SVC), an intrathoracic structure, offers a more reliable parameter of fluid responsiveness in the ventilated patient, but requires TOE for visualisation.
LV end-diastolic area (EDA) can be easily measured in short-axis views of the heart. Reduced LVEDA is suggestive of hypovolaemia and may predict fluid responsiveness. However, in the presence of RV failure, the LV will appear underfilled, and inspection of the LV in isolation may result in inappropriate fluid administration. Measurement of LVOT velocity-time integral (VTI) provides a surrogate for LV stroke volume. Respiratory variation in LVOT VTI has been used to guide fluid responsiveness and to calculate the cardiac output.
Procedural Guidance
Ultrasound plays a pivotal role in the field of mechanical circulatory support. Intra-aortic balloon pump insertion and positioning can be optimised by TOE, and immediate aortic complications readily detected. Echocardiography can be used to guide cannulation of extracorporeal life support (ECLS), to manage patients on ECLS and to assess suitability for weaning from support. Pericardiocentesis should be performed under echocardiography guidance.
Summary
The role of echocardiography in the critical care setting is expanding. As with any monitoring tool, physicians need to be aware of its limitations and integrate the findings with the clinical context. Comprehensive training, accreditation and maintenance of skills are essential to ensure appropriate use and interpretation.
Learning Points
Echocardiography is pivotal in the evaluation of the unstable patient.
Focused echo is a skill to be possessed by all critical care physicians.
Comprehensive training is essential to ensure appropriate use.
Objective quantification of ventricular function is recommended.
Echocardiography has an increasing role to play in patients on ECLS.
Further Reading
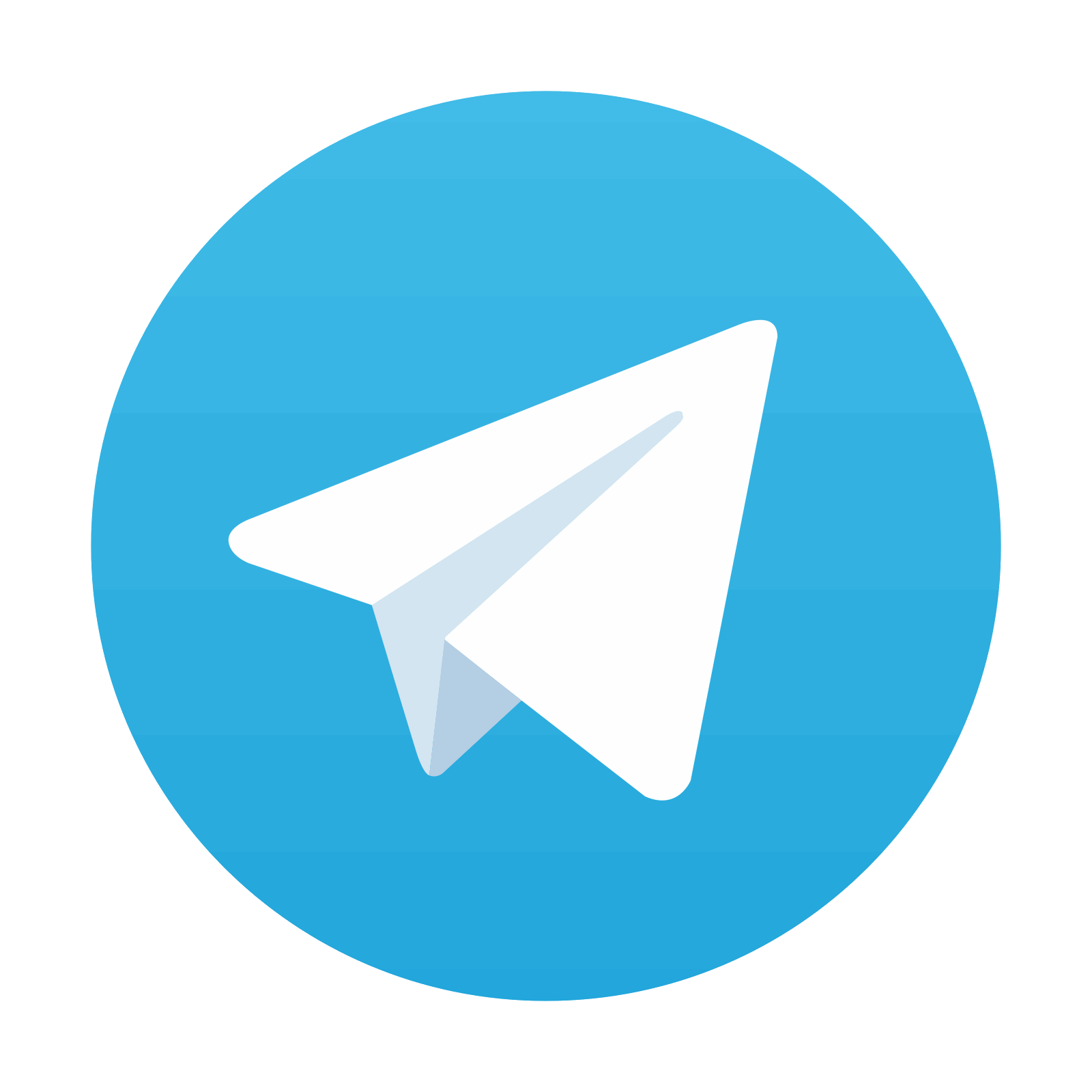
Stay updated, free articles. Join our Telegram channel

Full access? Get Clinical Tree
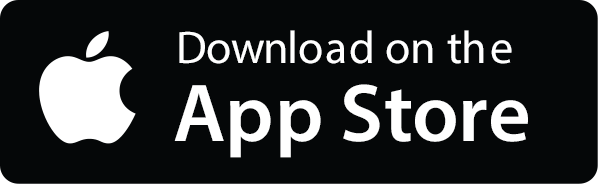
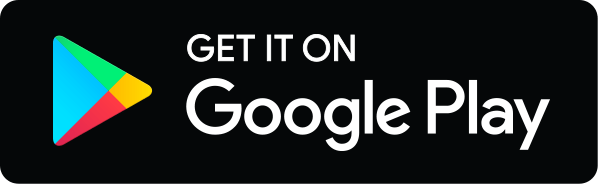
