(1)
King’s College Hospital NHS Foundation Trust, King’s Health Partners, Denmark Hill, London, SE5 9RS, UK
Abstract
The early 1980s saw radical advances in perioperative monitoring. In a relatively short period of time, the “finger on the pulse”, manual measurement of blood pressure using Riva Rocci cuff, aneroid mercury column and listening for Korotkov sounds with a stethoscope and (if you were lucky) a primitive ECG machine were replaced from the early to mid 1980s with pulse oximetry, automated non-invasive BP, end tidal carbon dioxide and anaesthetic agent monitoring and sophisticated ECG technology. This was a major transition in monitoring technology but, since then, progress has been halted. From the late 1990s technology has become widely available to measure the extent of anaesthesia induced cortical suppression using EEG monitoring technology (e.g. BIS, Medtronic, USA), cerebral and tissue oxygenation using the Invos cerebral oximeter (Medtronic, USA) and various flow monitors using Doppler and arterial pulse waveform analysis from the radial or femoral artery lines. In 2011 NICE (National Institute for Health and Care Excellence, UK) guidelines were introduced advocating the use of flow monitoring and then in 2012 “depth of anaesthesia” in high-risk surgical patients. Although widely available this technology is still only used in a small percentage of patients in the UK. Recent evidence indicates that the introduction of flow monitoring alongside existing monitoring does not radically improve outcome in terms of reduction in complications and has no effect on 30 day and in-hospital mortality, which remains at around 5 % in the elderly, high risk surgical population. This is probably due to a fundamental misunderstanding of circulatory physiology which has led to protocols and interventions using flow monitoring which are inappropriate. Often they result in massive fluid and sodium overload. This is particularly a problem in the highest risk patients. The rapid rise in the number of such patients has I believe necessitated a radical re-evaluation of circulatory physiology under anaesthesia and its implication for management. Using the combination of these new monitoring technologies (flow, depth of anaesthesia and cerebral and tissue oximetry) in a multimodal monitoring strategy provides maximum benefit by reducing complications, the necessity for high dependency care and mortality. This combined strategy and its rationale is the subject of this chapter.
Keywords
MonitoringCardiac outputDepth of anaesthesiaCerebral oxygenationCirculation during anaesthesiaCrystalloidsColloidsOutcome following surgery13.1 Introduction
Why do we need to change our current perioperative monitoring strategy when it is clear from recent analyses that death in the immediate perioperative period is incredibly low? Anaesthesia is completely safe isn’t it? In a survey linked to the NAP 5 (National Audit Project) study of the Royal College of Anaesthetists in the United Kingdom on accidental awareness during general anaesthesia (AAGA) investigators made several pertinent comments [1]:
“The low mortality rate of GA (0.06 % or one in 1718) during surgery is notable”.
“Many patients are scared of anaesthesia and this figure can only be reassuring for them”.
“During the period of time when they are cared for by anaesthetists, the risk of death is low indeed”.
“This low mortality rate is in marked contrast to the report by EUSOS [2] of an overall 4 % or one in 25 mortality rate for in patient elective major surgery”
The implication then is that anaesthesia is completely safe and with a mortality rate of one in 2000 one might expect little to be gained from a new multimodal monitoring (MMM) strategy. However, there is a very important caveat which arises from the following statement made in the paper.
“These differences (in immediate and intermediate mortality) highlight the potential impact advances in perioperative care by anaesthetists, surgeons and intensivists might have on overall mortality rates after surgery”.
Patients thus die very rarely (0.05 %) on the operating table or in the first 1 or 2 days post-operatively but up to 4 % may die during a hospital admission [2]. It is thus for anaesthetists to recognise that deficiencies in intraoperative and immediate perioperative management may start the “time bomb ticking” for complications leading to mortality occurring later on during hospital admission. These deaths cannot simply be accounted entirely by surgical complications. The challenge we have today is to translate the very low immediate perioperative mortality indicated by the NAP5 study data to the whole perioperative period.
13.2 The Increasingly High-Risk Surgical Population
A recent review highlighted the type of patients and surgery who constitute a high-risk population [3]. The elderly patient with one or more comorbidities of the cardiovascular, respiratory or renal systems [4] is now the largest category of high risk patient. The increasing burden of obesity and diabetes must also be taken into account and may affect a younger population. It is estimated that one million of the estimated three million operations carried out in the UK in 2013 were on patients over 65 [1]. In a study in the USA the elderly underwent surgery four times more often than the young and in a recent Australian and New Zealand study, 5 % of patients over 70 died within 30 days of surgery [5]. The “Reason” study investigators commented that “strategies are needed to reduce complications and mortality in older surgical patients”. Given the 4 % mortality in the EUSOS study we might conclude that strategies are required for younger and fitter patients too [2]! Strategies and protocols need to be guided by monitors not “recipe books” and we need to individualise patient care [6]. Anaesthetists must recognise that deficiencies in intraoperative management are deleterious for long-term patient outcome.
13.3 Monitoring Technology
The late 70s and early 80s saw a dramatic advance in monitoring technology in the perioperative period. The “finger on the pulse” was replaced by the pulse oximeter and it also became possible to routinely monitor end tidal carbon dioxide and inhalation agent concentration. In addition, manual intermittent blood pressure using a conventional arm cuff or oscillotonometer was replaced by automated non-invasive BP machines. ECG monitoring advanced with the appearance of memory scopes and automated analysis of ST segment depression. This represented an enormous advance in a very short period of time. However, since from around 1984 until 2011 there have been no major advances in routine perioperative monitoring. Indeed, assessment of patient status still relies solely on finger sPO2, blood pressure monitoring and the presence of ischaemia indicated on a bipolar, three lead ECG system not really designed to pick this up. In addition, in the UK even if more sophisticated monitoring was available it was usually only placed in the operating room and not available in the induction room, still beloved by 80 % of anaesthetists [1]. Most hospitals in Europe and the USA do not have a rigorous protocol of haemodynamic management even for high risk patients [7].
13.4 The Implications of Poor Blood Pressure Control During Anaesthesia
Most anaesthetists will try and maintain mean arterial pressure (MAP) within about 20 % of the pre-induction value. What is the evidence that low MAP is harmful? A recent large retrospective study from the Cleveland clinic found that “a mean arterial pressure less than 55 mmHg predicted adverse cardiac and renal related outcomes; however, the limitations of retrospective analyses must be considered. Notably, any amount of time at a MAP less than 55 mmHg was associated with adverse outcomes [8]”. No room for complacency! They also go on to say that “further research is required to determine whether interventions to prevent and rapidly treat intraoperative hypotension ameliorate the risk of acute kidney injury and myocardial injury in patients having non-cardiac surgery”. Although this seems a reasonable statement it may lead to further problems as correction of MAP by vasoconstrictors may result in a decrease in flow and oxygen delivery, further compromising oxygenation [9]. So, it is crucial that we know what causes the fall in MAP on induction and during maintenance of anaesthesia in moderate and high-risk patients in order to make appropriate interventions (see later).
13.5 Interventions Used by Anaesthetists to Maintain MAP During Anaesthesia
Most anaesthetists will use fluids and/or vasoactive drugs to correct changes in MAP without consideration of the “depth of anaesthesia” or degree of cortical suppression induced by the anaesthetics used.
13.5.1 Using Fluids
As far as fluids are concerned current practice throughout the world is still influenced by the ground breaking paper from Shires et al from Dallas published in 1961 in the Annals of Surgery [10]. The investigators used a triple indicator dilution technique which suggested that there was a profound loss of functional extracellular fluid during major surgery, later nicknamed “third space”, which could amount during surgery to up to 15 ml.kg−1 body weight hr−1. Given that some patients might therefore need 1 L or more per hour of extracellular fluid equivalent replacement they proposed the introduction of a lactated version of Ringer’s solution, a balanced salt solution which contains a more physiological concentration of chloride in contradistinction to so-called “normal saline”, 0.9 % NaCl. It literally opened the floodgates as anaesthetists could point to excessive “third space loss” as the cause of intra-operative hypotension in major surgery and were given carte blanche to use excessive amounts of fluid such as lactated Ringer’s (Hartmann’s) in trying to reverse the fall in blood pressure.
It is clear that the original authors very quickly became concerned that excessive fluids were now been given rather than the fluid restrictive practice which had existed prior to the paper of 1961. Moore and Shires in 1967 stated [11] “the objective of (perioperative) care is restoration to normal physiology and normal function of organs, with a normal blood volume and functional body water and electrolytes. This can never be achieved by inundation (with fluids)”. It is clear that giving massive volumes of balanced salt solutions will not achieve anything resembling normal physiology! The dangers of excessive fluids and volume overload with high CVP have recently been re-iterated by Marik. e.g. pulmonary oedema and increased extra-vascular lung water, impaired oxygenation, altered pulmonary and chest wall mechanics, increased work of breathing, myocardial oedema etc. The list is long [12].
Interestingly, recent papers and reviews have confirmed that “there is no convincing evidence supporting the existence of the non-anatomical third space loss neither in haemorrhagic shock nor in surgery of any kind” [13–15]. This highlights another problem. Third space loss does exist in conditions such as septic shock, anaphylaxis and burn injury and it is important to distinguish fluid and flow requirements in elective surgery in contradistinction to haemodynamic changes in the intensive care unit where sepsis may well be a serious problem. Reviews of fluid management often mix up these two completely different scenarios and thus cause unnecessary confusion.
It is thus disappointing to see a large scale prospective trial of restrictive versus liberal fluids during surgery, the Relief study, which will be running until November 2018 where large volumes of fluid are given in the liberal group. Intervention patients may receive 4 days Na+ requirement in a 2-h procedure! In 2016 patients are still receiving too much fluid and Na+ in the perioperative period with implications for poor outcome (see later).
13.5.2 Using Vasoactive Agents
It is easy to restore MAP to “normal” using vasoactive agents such as metaraminol, phenylephrine or even noradrenaline [16]. However, MAP may then be maintained at the expense of cardiac output as shown in Fig. 13.1 which demonstrates that reliance on MAP alone may be associated with profound falls in cardiac output (CO) and oxygen delivery (DO2) and subsequent build-up of oxygen debt (vide infra). It is crucial to know the cause of the fall in MAP before using vasoactive compounds. This may be a real problem when the flow monitor does not accurately track changes in flow with changes in vasomotor tone [17]. This may lead in some cases to a fall sense of security.


Fig. 13.1
LiDCOviewPro Case analysis using LiDCOview pro software (www.lidco.com). The top horizontal trace in red shows nominal cardiac output (nCO). The next trace in green shows systemic vascular resistance (SVR). The third trace black hatching is blood pressure (BP) from an arterial line, mean pressure (MAP) is indicated by the red line. The bottom trace shows stroke volume (SV) in blue and heart rate (HR) in purple. The numbers on the BP segment indicate interventions. 1 indicates induction of anaesthesia and 2 is intubation. Various vasoactive compounds were given during the 10 h. procedure but it can be seen that nCO is maintained at only 30 % of starting value (2.2 vs. 6.6 lpm) for the majority of the operation. However, MAP and HR are maintained at normal values and would not suggest any problem. The massive reduction in oxygen delivery (DO2) and build-up of oxygen debt thus occurred without any indication from conventional monitoring of BP and HR
13.6 Cardiac Output Changes Following Induction and Maintenance of Anaesthesia
13.6.1 Introduction
Most anaesthetists opine that the fall in MAP following induction is mainly due to peripheral vasodilation with a fall in systemic vascular resistance (SVR) but little change in CO and oxygen delivery DO2 and incorrectly assume that a simple vasoconstrictor such as metaraminol will both correct MAP whilst maintaining flow (see above). The first measurement of CO during surgery using the dye dilution technique was carried out in 1960 and showed that the CO dropped by 20 % after induction [18]. However, it was only following the advent of continuous CO monitoring technology, particularly using analysis of the radial artery waveform (see later) that it was possible to follow the rapid changes of pressure and flow following induction of anaesthesia. Kamenik and Petrun, using the LiDCO rapid (LiDCO, UK), were able to demonstrate that the fall in MAP following BIS (Medtronic, USA) targeted induction using either propofol or etomidate was mainly due to a fall in CO and not due to a fall in SVR [19]. The fall may be as much as 40 % in elderly, high-risk patients. Knowledge of this potentially dramatic fall is essential to allow appropriate intraoperative fluid and vasoactive drug management of these patients.
13.6.2 Why Does Cardiac Output Fall on Induction and During Maintenance of Anaesthesia?
To understand what happens to the circulation during anaesthesia induction we need to go back more than 50 years to a series of papers by Professor Sharpey-Schafer at St Thomas’s Hospital Medical School in London [20–22]. He studied venous tone in patients and volunteers who were healthy, anaemic, hypertensive, following Valsalva manoeuvre, ganglion blocking drugs and blood donation. His comment that “it is clear that the usual clinical practice of following such cases (hypotension with ganglion blocking drugs, then being introduced for the treatment of hypertension [23]) by the measurement of arterial pressure by the antiquated cuff method can be of limited value”. He appreciated that the changes in MAP caused by ganglion blocking drugs especially when the patient was raised to the upright position were occurring too quickly to be picked up by conventional methods of measuring MAP in use at the time, such as Korotkoff sounds and the mercury manometer. Interestingly, hypotensive anaesthesia had been introduced into clinical practice by Enderby and colleagues at East Grinstead, Sussex, UK to allow more accurate plastic surgery to be carried out on airmen who had received facial burns during the Second World War. Enderby also noted the restrictions imposed on accurately measuring MAP during these cases and introduced the mechanical von Recklinghausen and Boulitte oscillotonometers into clinical anaesthetic practice in the UK. These he found more accurate at low pressures than the conventional blood pressure cuff [24]. This also allowed remote measurement of blood pressure and removed the necessity of placing the stethoscope over the brachial artery. It is noteworthy that modern automatic oscillo(tono)meters are now the most common way of measuring blood pressure during anaesthesia. Sharpey-Schafer’s conclusion was that “it appears that the veins and not the arteries are the major factor in causing hypotension with ganglion blocking drugs when assuming the upright position”. Is the same true with anaesthetic drugs? Isolated hepatic portal veins and aorta taken from the rat were used to investigate the direct action of propofol. It caused a dose related decrease of tone in both types of blood vessel but concentrations required to produce this effect on veins were significantly lower than those required to produce similar changes in the isolated artery preparation. The authors concluded that this direct action may contribute towards the hypotensive effects of propofol [25]. Venodilation with propofol resulted in an increase in venous capacitance in dogs and there was no change in SVR to account for the change in MAP. They concluded that anaesthesia with propofol is accompanied by decreased CO secondary to venodilation and reduction in preload. Cardiac output and MAP are preserved as long as preload is maintained [26]. It was also noted by Sharpey-Schafer that underlying venous tone is markedly increased in patients with heart failure and anaemia, particularly pertinent to the high-risk and elderly patient population. It is therefore reasonable to conclude that these patients are more susceptible to the venodilation induced by propofol and probably other anaesthetics.
Venodilation is thus a key feature of anaesthesia which has been very little recognised [27]. Following venodilation the fall in MAP is due not only to falling preload, stroke volume (SV) and CO with a consequent reduction in DO2 but also due to a shift of volume out of the arterial tree into the dilated venous compartment [27]. The question is whether we should maintain venous capacitance with fluids (as in the dog study above) or by administration of a venoconstrictor. Our experience with the venoconstrictor, phenylephrine, the effect monitored by the LiDCOrapid (see later) commenced in low-dose pre-induction can maintain venous tone, cardiac output and MAP without unnecessary fluid administration to maintain venous capacitance [28].
Crucially important is the fact that measuring CO with whatever technology after induction of anaesthesia may greatly underestimate the true resting CO and therefore any intervention which is supposedly designed to “optimise stroke volume” (whatever that means) and maintain DO2 will be flawed and may result in unnecessary excess fluid and Na+ administration to the detriment of outcome in high-risk patients [12].
13.7 Recommendation to Add Flow Monitoring
Although flow monitoring technology had been around for many years mainly using the pulmonary artery thermodilution catheter (PAC) this technology came to be considered too invasive for routine use and some studies seemed to question its usefulness in high-risk surgery [29]. In addition, it only provided intermittent values for flow. Doppler technology, providing continuous measurement of flow velocity, from which was calculated stroke volume and CO, was first employed in perioperative management in the 1990s [30] but was popularised by the introduction of the Deltex oesophageal Doppler in the early 2000s (Deltex, UK). Three major trials published between 2002 and 2006 suggested benefits to higher risk abdominal surgery patients by modest reductions in hospital length of stay [31–33]. However, adoption of this technology had been extremely limited and it was left to the National Institute of Health and Care Excellence (NICE) in the UK to recommend this technology for a distinct group of high-risk surgical patients undergoing particular surgical operations in 2011 [34]. Even then, the guidance was questioned and argued over in editorials [35, 36]. In the intervening period many other continuous flow monitoring technologies had been developed by various companies, usually using analysis of the arterial waveform either from the descending aorta via a catheter placed in the femoral artery (PiCCO, Pulsion, Germany) or from the radial artery (LiDCO, UK and Flotrac, Edwards Lab, USA). Both PiCCO and LiDCO technologies allowed for accurate calibration either using a trans-pulmonary thermodilution technique as with PiCCO or a lithium dilution technique with LiDCO. It was not surprising that these companies protested that a single flow monitoring technology had been advocated by NICE and thus in 2012 the advice was extended to any flow monitoring technology which in the European Union had a CE mark. (It is important to point out that the CE mark does not guarantee that the device measures flow accurately only that it is been manufactured to a certain standard).
It is now possible using relatively non-invasive technology to measure flow accurately and continuously throughout the perioperative period.
13.8 How Did the Addition of Flow Monitoring Affect Anaesthetic Practice and Patient Outcome?
13.8.1 Protocols and Interventions
Although flow monitoring was advocated in high-risk patients by NICE it was not clear what protocol would or should be used by anaesthetists. The principle of goal directed therapy (GDT), see later, had been introduced to try and achieve population-based DO2 targets and the figure of 600 ml of oxygen delivery per m−2 Body Surface Area (BSA) is often quoted. However, although this target is usually used in the immediate postoperative period to reduce repay oxygen debt and reduce complications [37] it is less frequently used during high-risk surgery [38] as will be discussed in the next section.
13.8.2 Flow Time Corrected (FTc) and Stroke Volume “Optimisation” Strategies
The original Doppler studies suggested that flow time, corrected for heart rate, (Ftc) maintained at about 350 msec seemed to indicate that patients were optimally filled [31]. This important parameter was discarded following the introduction of SV optimisation or maximisation strategies (see Fig. 13.2). This was based on the opinion that poor outcome was a result of inadequate tissue perfusion and giving additional fluids to improve perfusion would result in a better outcome. Thus if the patient was fluid responsive it was assumed that they needed fluids. Thus 200–300 ml. boluses of colloid (3–4 ml.kg−1) are given over a period of less than 5 min and repeated until additional boluses no longer produced a greater than 10 % increase in stroke volume. (A 10 % threshold is chosen as a 300 ml. fluid challenge will produce haemodilution and around a 5 % reduction in oxygen carrying capacity and this would need to be offset by at least a 5 % increase in SV for the fluid challenge to increase DO2). It is clear that just because the patient may respond to fluids with an increase in SV does not necessarily mean that they need fluids (see also next section). In addition, since the Doppler is placed post induction none of the studies appreciated the extent of the fall in CO and DO2 post induction.


Fig. 13.2
Fluid management using the Oesophageal Doppler algorithm suggested by the Deltex Medical Company, Chichester, UK (Reproduced with permission from Bidd et al. [60])
13.8.3 Systolic Pressure and Stroke Volume Variation as Predictors of Fluid Responsiveness
Another parameter available from monitors showing the dynamic pressure waveform from an arterial line is pulse pressure variation (PPV) [39]. Fluid depleted patients show high values of PPV and this can be used as an indication to give fluids. In addition, if flow is calculated from the pressure waveform (see above) stroke volume variation (SVV) can also be used as an indication of fluid responsiveness. These parameters are only available following intubation and institution of intermittent positive pressure ventilation (IPPV) using a tidal volume of at least 8 ml.kg−1 in a patient with a closed chest and in sinus rhythm. A SVV of between 5 and 10 % suggested adequate filling whereas a figure above 13 % suggested fluid depletion [39, 40]. As will be seen later excessive quantities of unnecessary fluid and Na+ have deleterious implications for both the peripheral circulation and the cardiovascular system.
13.9 Does the Current Practice of Adding Flow Monitoring to Existing Monitoring Actually Improve Outcome?
Treating individual patients using population-based estimates of DO2, “maximising” or “optimising” SV and trying to achieve certain targets of PPV or SVV may not produce the benefits expected. In a randomised controlled trial of intraoperative goal directed fluid therapy in aerobically fit and unfit patients having major colorectal surgery it was concluded that “SV optimisation conferred no additional benefit and in aerobically fit patients had a detrimental effect on the primary outcome in terms of increased patient length of stay”. This study used a SV maximisation strategy and also included replacement of “third space loss” at 10 ml.kg−1.h−141. Another study from Brazil using a calibrated LiDCO plus monitor tried to achieve GDT targets of 600 ml.m−2 BSA DO2 using fluids and inotropes (dobutamine) in a high-risk surgical population randomised to two baseline fluid groups i.e. a restrictive group received 4 ml.kg−1.hr−1 of lactated Ringer’s per hour versus 12 in the standard group. Although DO2 targets were better achieved in the standard group the outcome was better in the restricted group [38]. It is also clear that SV maximisation using the Doppler or LiDCO may not produce any additional benefit over a simple restrictive fluid strategy [41].
13.10 Fundamental Misconceptions of Circulatory Physiology During Anaesthesia
I have already considered the importance of distinguishing between venodilation and vasodilation and implications for management. Other factors have come to light recently which will have profound implications for fluid management during major surgery in high risk patients. These are discussed in more detail in a recent review [27].
13.10.1 The Endothelial Glycocalyx Layer
The clinical implications and physiological importance of the endothelial glycocalyx layer (EGL) have been pointed out in three recent reviews and have altered our understanding and management of fluids during surgery [42–44]. The identification of the EGL, a fragile, sieve like barrier between the plasma and the endothelial cells, requires a fundamental re-evaluation of fluid transfer into and out of the interstitial space from arteriolar to venular end as proposed by the Starling equation. It now appears that gross fluid transfer is much less than previously suggested. Fluid crossing the EGL and into the interstitium returns to the circulation predominantly by the lymphatics. The EGL is the true effective barrier between the circulation and the interstitial fluid. As it is fragile it is easily damaged by ischaemia, sepsis, burn injury, anaphylaxis and pertinent to this discussion, hypervolaemia. Excess fluids and Na+ to replace non-existent “third space loss” may not only damage the EGL directly by compression and distortion but also lead to an increase in atrial natriuretic peptide (ANP) which itself damages the layer. Albumin appears to be protective and sits within the sieve like membrane partially preventing excess fluid loss. Excess fluid administration in the perioperative period leads to a fall in albumin which again damages the EGL. The implication is that “third space loss” may actually be precipitated by excess fluids rather than they being a replacement for third space loss. Maintaining pre-induction values of CO and DO2 (e.g. by using colloids/blood to replace obvious losses to maintain circulating volume) rather than trying to maximise them to nominal population based targets may be the more appropriate strategy. This will also help to minimise unnecessary intraoperative fluids and Na+ which may be deleterious in the high risk patient.
13.10.2 Cardiac Output and the Peripheral Circulation
In experiments in the 1950s Guyton showed that the need of the body for oxygen is the real regulator of cardiac output [45]. In other words, CO is determined by the metabolic needs of the tissues and not the other way round. In general, tissues (except skin and kidney) regulate their own flow according to their metabolic demands. Summation of these tissue flows determines venous return and venous tone determines how much of that venous return actually gets back to the heart as preload. Venous tone is decreased by anaesthesia and small doses of venoconstrictors prevent or substantially reduce the venodilation (see earlier). The heart plays a permissive role and does not regulate its own output, so it is not appropriate to use a SV optimisation or maximisation strategy or try to achieve a perioperative increase in global blood flow as an intervention strategy in the elective patient. It seems much more appropriate to just maintain pre-induction CO and DO2.
13.11 Does The Use of Flow Monitoring and Maximising Stroke Volume and Oxygen Delivery Improve Outcome and Reduce Mortality?
This is clearly a very important question as if our current strategies are not improving outcome to the extent we desire then we must consider a new strategy based on a clearer understanding of circulatory physiology, as indicated above [27].
In a recent Cochrane collaboration review entitled “Perioperative increase in global blood flow to explicit defined goals and outcomes after surgery”, the investigators noted that using this strategy there was no decrease in mortality and length of stay was decreased on average by only 1 day [46]. There were reductions in complications which accounted for the reduction in length of stay. However, the investigators concluded “the balance of current evidence does not support widespread implementation of this approach to reduce mortality but does suggest complications and duration of hospital stay are reduced”. This was hardly a ringing endorsement of this strategy and worryingly there was no mortality reduction. In addition, the latest large-scale prospective randomised trial of the effect of perioperative, CO guided haemodynamic therapy on outcomes following major gastrointestinal surgery, the so-called Optimise trial, did not reduce a composite outcome of complications and 30 day mortality [47]. A recent editorial by two authors of the Challand et al. study [48] mentioned earlier concluded that SV optimisation/maximisation using the algorithm shown in Fig. 13.2 provides “no marginal benefit in aerobically fit patients having elective surgery within a contemporary enhanced recovery pathway” [49].
13.12 Why Does Flow Monitoring Using Current Strategies Not Produce the Expected Benefits, Especially Reduction in Mortality?
Earlier reviews of outcome using flow monitoring technology appeared to suggest that mortality was reduced in high risk patients [50] especially in studies where mortality in the control group exceeded 10 %. However, the introduction of the enhanced recovery after surgery program (ERAS) has meant that overall surgical care has improved in the last 10 years or so [51]. This would likely produce an improvement in outcome and a reduction in mortality independent of any effect of flow monitoring. Any benefit expected from flow monitoring is also likely to be reduced due to misunderstanding of circulatory physiology, the role of the heart in the circulation and third space loss alluded to above. Patients may receive too much fluid and Na+ and this may be deleterious in these patients and offset any benefits from improved care such as ERAS.
13.13 Conclusion on Goal Directed Therapy and Outcome
It is clear that there has not been as much benefit as expected in particular there has been no effect on mortality reduction versus current practice and mortality remains unacceptably high. What is the way forward?
13.14 The Role of Individualised Haemodynamic Therapy
In a review of perioperative haemodynamic therapy the authors stated that “once an individualised approach will be identified, the terms of liberal, restrictive and supranormal values could eventually be replaced by adequate haemodynamic support that fits every patient’s own needs” [6]. The question is, can we identify a better individualised approach to further improve outcomes and in particular reduce mortality in this high risk patient group?
The goal of twenty-first-century individualised intraoperative management of the high-risk surgical patient should be directed towards avoiding the build-up of oxygen debt. This can only be achieved by using multi-modal monitoring (MMM) to assess and maintain DO2 using a reliable flow monitor from pre-induction in the elective patient and then maintained as close to the pre-induction level as possible during surgery and into the immediate postoperative period.
DO 2 is the product of arterial oxygen content (CaO 2 ) multiplied by cardiac output.
CaO2 is the product of Hb concentration in g.L−1 multiplied by arterial oxygen saturation (sPO2) as a percentage divided by 100 multiplied by 1.34, giving the number of mls of oxygen carried by 1 g of Hb when fully saturated. If “normal” CO is 5 l.min−1 then DO2 is:
in the average 70 kg patient.

So, clearly we need to track CaO2 as well as CO throughout the perioperative period to be able to maintain DO2. In patients who are experiencing blood loss, frequent measurements of Hb are clearly necessary. To be able to maintain CO and DO2 during surgery, but at the same time prevent excessive or inadequate levels of anaesthesia, we need to have some monitor to assess depth of anaesthesia. The latter term is better expressed as adequate cortical suppression [52, 53], maintained at an appropriate level in response to the intensity of surgery throughout the procedure. Since our main strategy is to maintain DO2 and tissue oxygenation, a rational monitor to use alongside flow monitoring and depth of anaesthesia monitoring is a cerebral/tissue oximeter (see later) [54]. These three components of flow, depth of anaesthesia and cerebral/tissue oxygenation form the three strands of the MMM strategy.
13.15 Oxygen Debt and Why It Is Bad for High-Risk Patients
The concept of build-up of oxygen debt and contribution to poor outcome in high-risk surgical patients was first evaluated by Shoemaker et al in the late 80s and early 90s [55–58]. He measured oxygen consumption (VO2) in his high-risk patients at pre-induction and then throughout anaesthesia using a PAC. The difference between VO2 intra-operatively (less 15 % which Shoemaker considered was the likely reduction in VO2 due to the effects of anaesthesia) and that obtained from the pre-induction reading constituted oxygen debt and clearly the greater the difference and the longer it went on for, the greater the oxygen debt would be at the end of the procedure.
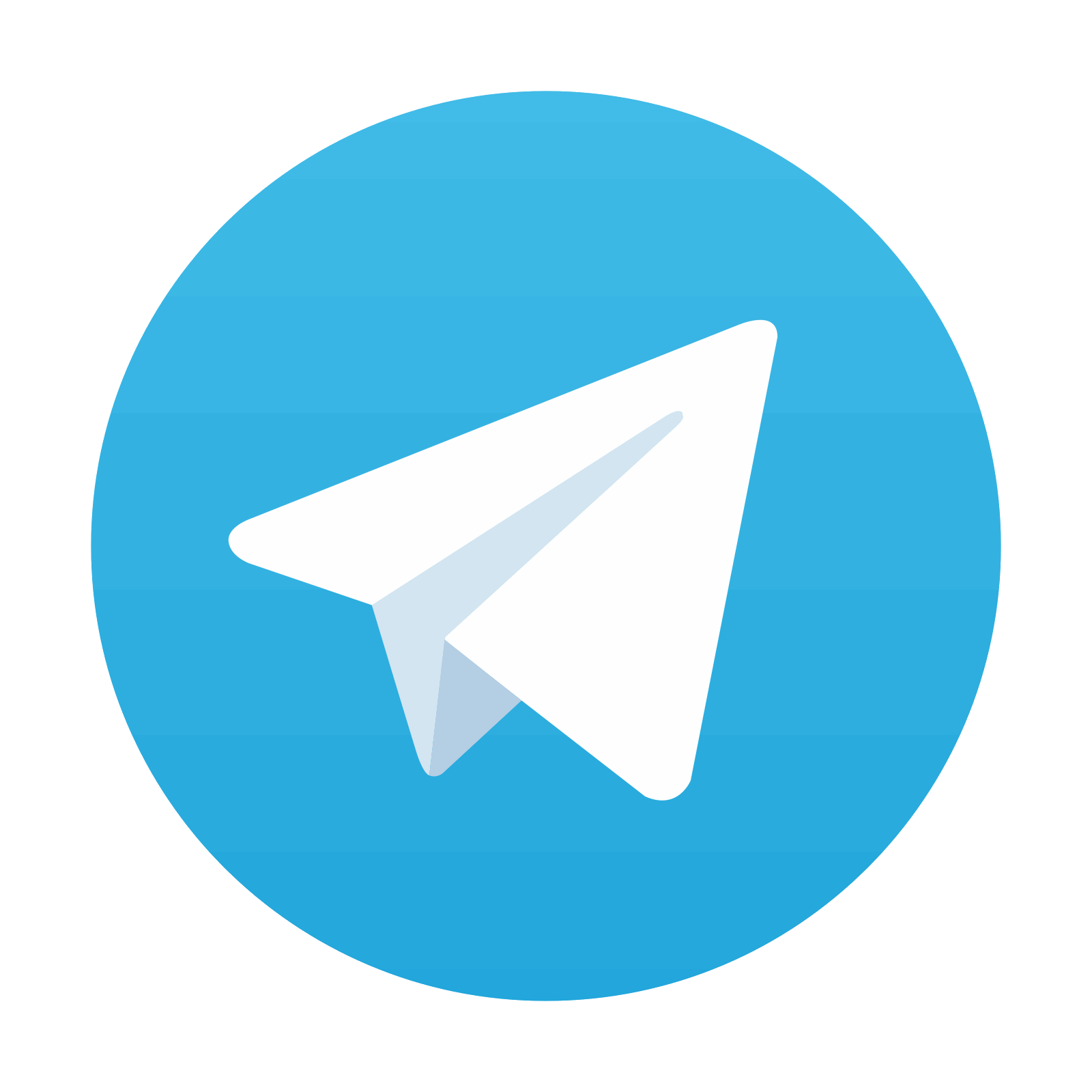
Stay updated, free articles. Join our Telegram channel

Full access? Get Clinical Tree
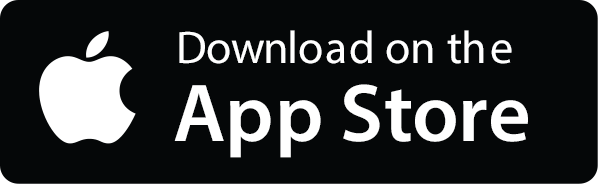
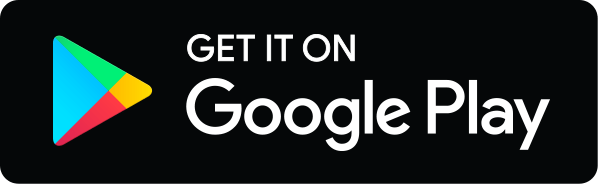