Abstract
Lung cancer is among one of the most commonly diagnosed malignancies and is the leading cause of cancer-related mortality in both men and women globally, with an estimated 1.8 million deaths annually. Moreover, it is also the leading cause of cancer related deaths in the United States (U.S.), with an estimated 127,000 deaths annually. Approximately 50% of patients who undergo chest Computed Tomography (CT) are found to have a pulmonary nodule (PN), albeit 95% of these PNs are subsequently found to be benign. Further complicating the challenge of timely detection of lung cancer, is made more difficult by the fact that most patients are totally asymptomatic in early stage of disease.
However, given that sponsored studies by National Cancer Institute (NCI) and other organizations showed a 20% reduction in lung cancer specific mortality with low dose CT scanning in patients at risk, it is reasonable to assume that clinicians will confront this clinical scenario more frequently. Consequently, due to these significant findings, the United States Preventive Services Task Force (USPSTF) recommended annual screening of high-risk patients. Therefore, as result of these recommendations, 240,000 new lung cancers were diagnosed in the U.S. in 2020, with an estimated 238,000 new cases in 2023. Given the multitude of challenges, the practice guidelines and recommendations for the management of these PNs are often tailored to available resources and trained personnel familiar with the various techniques and technologies.
This review will discuss the evolution of various advancements when tissue biopsy is required: from sputum cytology, nonguided bronchoscopy, percutaneous CT guided biopsy, guided advanced bronchoscopic techniques such as endobronchial ultrasound (EBUS), radial Endobronchial Ultrasound (rEBUS) to the latest advancement of robotic-assisted bronchoscopy (RAB). Furthermore, as many of the aforementioned techniques require anesthesia, as integral members of the multidisciplinary team, anesthesiologists are often in the unique position of facilitating diagnosis and subsequent treatment by other subspecialists when providing anesthetic care for these patients with PNs.
Additionally, the common anesthetic considerations and implications for the preoperative, intraoperative, and postoperative periods will be elucidated further, with special emphasis on the unique considerations for RABs. Combined hybrid procedures with RAB, EBUS and surgery will also be reviewed, as they offer potential reduction in time of diagnosis to definitive treatment. Lastly, the strategies employed to mitigate some of the commonly encountered challenges faced by anesthesiologists when caring for these patients will also be reviewed.
1
Introduction
When clinicians are confronted with the common radiographic finding of a PN, the initial goal is to rule out malignancy from a benign process such as prior infections, scar tissue or other benign conditions. Depending on the patient’s risks, health status and smoking history, current practice guidelines recommend three distinct pathways for diagnosis and management of PNs: close monitoring with serial CTs, obtaining tissue biopsy for establishing pathologic diagnosis, and removal by surgery [ ]. The main objective being to promptly diagnose malignant disease processes thereby facilitating timely surgical resection, all the meanwhile avoiding unnecessary invasive testing, treatment and surgery in those patients with benign conditions.
1.1
Sputum cytology
Historically, there have been tremendous advancements made over the last five decades when tissue specimen confirmation is needed to make the pathologic diagnosis. In previous decades, sputum cytology specimens have been utilized in diagnosis of malignant lesions, however studies have reported a wide range of sensitivities for sputum cytology with sensitivity ranges from 0.42 to 0.97 [ , ]. Gilbert et al. reported that there are a variety of methodological reasons for the different ranges reported for sensitivity of sputum cytology [ ]. For example, the number of sputum samples, specimen adequacy and location of suspected lesion play a strong role in sensitivity of this technique in the diagnosis of malignancy. Despite demonstrating higher sensitivity for central lesions, the sensitivity of sputum cytology remains suboptimal for peripheral PNs.
1.2
Flexible bronchoscopy
Similar to sputum cytology, wide range of sensitivities have been reported for flexible bronchoscopy and its associated procedures such as biopsy, cytobrushing, washing, transbronchial needle aspiration, bronchoalveolar lavage, endobronchial needle aspiration. For example, Wagner et al. reported an overall sensitivity of 0.67 for central or endobronchial lesions [ ]. Whereas, others have reported a sensitivity for malignancy for conventional bronchoscopy ranging from 0.34 to 0.63 for peripheral PNs [ ]. Likewise, other reviews have demonstrated sensitivities not only change for location of lesion, with central lesions having higher sensitivity than peripheral, but also size of lesion; with lesion diameters greater than >2 cm having higher sensitivities (0.62) versus significantly lower sensitivities for lesions smaller than <2 cm (0.33) [ ].
However, studies such as the NLST and NELSON trial have reported that most PNs detected (80%) were located in the periphery of the lung [ , ]. The peripheral location presents a unique set of challenges for clinicians when utilizing conventional bronchoscopy: how to safely navigate, confirm and acquire samples from these small PNs. Furthermore, leaving some type of dye or fiducial marker represents yet another challenge when attempting to identify these PNs later intraoperatively during surgical resection given that conventional bronchoscopes diameters will not allow reaching these PNs, as they are only able to reach the sixth-generation bronchi [ ]. Lastly, the flexibility of conventional bronchoscopes is a significant limitation not only for peripheral lesions but also apical ones as well, especially when biopsy instrumentation is passed in the working channel, thus making maneuverability around acute angles and sharp turns more challenging.
1.3
Transthoracic needle aspiration
Likewise, transthoracic needle aspiration (TTNA) can be done with the radiologic assistance of CT, fluoroscopy and or ultrasound. The TTNA approach has been reported to have the highest sensitivity, with some authors reporting sensitivity as high as 0.96, while metanalysis have shown a range of 0.88–0.92 [ , ]. However, the main drawback continues to remain the high risk of pneumothorax, with ranges reported from 20% to 25% for all types of biopsies [ , , ]. Furthermore, despite demonstrating a high sensitivity for cancer diagnosis, TTNA procedures are associated with a high rate of pneumothorax, 25.3% when core biopsy is done and 18.8% when fine needle aspiration is performed. Of these, up to 11.9% of the cases required a chest tube placement [ ]. Moreover, although the TTNA approach allows biopsies of lesions where both conventional and advanced guided bronchoscopy are unable to reach due to the lack of airway passage to the location of the desired lesion, it is still not feasible to biopsy lesions bilaterally. Similarly, high risk patients such as those with prior radiation, surgery and or lesions located next to vasculature present additional clinical challenges for physicians when lesions are in these precarious anatomical areas.
1.4
Advanced guided bronchoscopy
In the last two decades, given the miniaturization of scopes, catheters and advancements in computer aided software, innovative technologies and techniques have emerged that facilitate guidance in the tracheobronchial anatomy, which are particularly useful in navigating to PNs. Electromagnetic navigation bronchoscopy (ENB) and virtual bronchoscopy navigation (VBN) can aid the bronchoscopist to reach a peripheral or apical lesion analogous to the manner the global positioning system helps the driver navigate a car. Typically, a thin cut CT scan (1.0 mm) scan done at end of full inspiration is used to create three-dimensional (3D) virtual reconstruction images of the tracheobronchial anatomy, helping create a map for the desired peripheral lesion.
Both ENB and VBN navigation systems are used primarily to navigate to peripheral PNs, but they can also be used to sample either mediastinal or hilar lymph nodes. However, the rEBUS approach creates images of the tissues adjacent to the tip of the 360-degree ultrasound probe, in this manner pulmonologists can identify the location of peripheral lesions by comparing the images of normal lung and cancer tissue created by the rEBUS probe. Once the lesion is identified, small biopsy forceps, needle and bronchial brushes can be advanced in the guiding sheath to obtain samples. Given the size limitation of the guiding sheath, multiple biopsy specimens are needed to get an adequate sample. Although the complication rates such as bleeding and pneumothorax are significantly lower (reported range of 0–3.6%) than TTNA and these procedures are well tolerated, their sensitivities for lung cancer are not as high TTNA, with recent metanalyses reporting sensitivities of 0.72 for rEBUS and 0.77 for ENB [ ].
The newest advanced bronchoscopy is the RAB, which has attempted to improve the sensitivity for the diagnosis of malignant lesions, since the aforementioned diagnostic bronchoscopic technologies have suboptimal sensitivities for diagnosis of malignant lesions given their inherent technical limitations. The fundamental components of the RABs technologies include some type of image-based airway navigation, real-time airway visualization, intraprocedural confirmation of imaging, and lastly sample-acquisition tools. Both Food and Drug Administration (FDA) approved RAB systems, the Ion Robotic-Assisted Endoluminal Platform (Intuitive Surgical Inc., February 2019) and the Monarch Robotic-Assisted Bronchoscopy Platform (Auris Health Inc., March 2018) were introduced specifically to improve the precision during sampling by simultaneously enhancing navigation, confirmation and acquisition. Fielding et al. reported that the utilization of RAB gave an overall diagnostic yield of 79%, with a malignancy-specific diagnostic yield of 88% [ ]. Similarly, the multicenter prospective evaluation of the Ion Endoluminal System (PRECIsE) trial demonstrated a diagnostic yield of 96.7% with no adverse events of hemorrhage or pneumothorax with RAB [ ].
An additional potential advantage is the seamless integration of these robotic platforms with EBUS, rEBUS, cone beam Computed Tomography (CBCT), and even the da Vinci Surgical System (Intuitive Surgical Inc.). Likewise, the utilization of adjunct techniques such as rEBUS and CBCT can further increase sensitivity by enhancing confirmation of the PN. Moreover, if a patient has a positive confirmation for malignancy, staging can be done simultaneously via EBUS and with the usage of RAB in select patients, the localization of these PNs can be facilitated by marking dyes or fiducial markers. Thereafter, in some instances, appropriately selected patients are provided definitive surgical resection in hybrid suites or operating room in a single setting anesthetic.
1.5
Robotic bronchoscopy
Optimizing the diagnostic yield of PN biopsy without sacrificing safety is critical as innovation in navigational bronchoscopy continues to evolve. RAB is the most recent in the evolution of modalities utilized for obtaining PN biopsies. The two commercially available platforms differ in some key areas, notably the technology utilized to target the lesion. Both Monarch and Ion platforms afford superior maneuverability within smaller peripheral and apical airways, further reach, superior stability, and enhanced tissue acquisition. Both systems have similar limitations despite differing navigational technology systems [ , ].
1.6
Monarch robotic system
The Monarch Robotic System consists of a bronchoscope system, cart, and tower. The bronchoscope system is a telescoping design with an outer sheath (6.0 mm outer diameter) and an inner bronchoscope (4.2 mm outer diameter) with a 2.1 mm inner diameter working channel. Maneuverability and articulation are improved with the inner bronchoscope while the outer bronchoscope enhances stability. A distinguishing feature of the Monarch platform is the ability to manipulate the outer sheath and inner scope independently. The “leapfrog technique” utilizes the intersection of the inner scope and outer sheath as a fulcrum to increase the flexibility while maintaining control over the robotic scope. The robotic scope can be navigated through acute angles commonly seen in the upper lobes and superior segments by driving the flexible scope into an airway followed by advancing the sheath over the scope in an alternating pattern. Also notable with the Monarch platform is the ability to maintain continuous vision throughout the procedure including tissue sample acquisition. This is especially useful when attempting to traverse tortuous distal airways [ ].
The Monarch Robotic System uses a proprietary navigation algorithm to fuse optical pattern recognition with electromagnetic positioning and robotic insertion data to calculate where the scope tip should be positioned within the lung to target the PN. After obtaining a preprocedural thin cut CT, the patient lies within an electromagnetic field which affords the ability to utilize reference sensors and the electromagnetic field generator built into the robot to merge the virtual CT with the patient’s real time anatomy. A virtual 3D reconstruction is generated which displays pathways to the target. The bronchoscope is advanced along the mapped pathway by the operator using a handheld controller. Navigation is aided by continuous ENB system feedback on distance to target and bronchoscope tip location. Once the PN is within reach, fluoroscopy or rEBUS can be performed to confirm navigation to the target. Tissue samples are obtained by passing sampling tools through the working channel [ , , ].
The REACH trail was the first assessment of the Monarch system in 2018. Chen et al. used human cadavers to compare the Monarch to a conventional flexible bronchoscope of the same diameter (4.2 mm). The Monarch system was noted to navigate further into distal airways based on depth as well as airway generation (9th vs. 6th). The study attributed this to the enhanced structural support impeding instrument prolapse and improved maneuverability leading to more success traversing acute airway angulations [ ].
The clinical implications of the Monarch system were further studied in the ACCESS study. Chen et al. reported a 97% diagnostic yield after artificial peripheral tumor targets were injected into cadaveric lungs [ ]. However, results of this study cannot be extrapolated fully to live patients given that CT Body Divergence (CTBD); which is essentially the difference between the predicted location of the target PN by the respective virtual navigation system compared to the actual location during bronchoscopy, is not comparable between cadavers and live patients.
Chaddha et al. studied 167 lesions in 165 patients, of which a significant percentage were small peripheral lesions. The average lesion size was 25 mm (10–40 mm) with 71.3% of lesions smaller than 30 mm. The majority of the lesions were peripherally located in the outer third of the lung (70.7%). The navigation success was reported to be 88.6% (148/167) of lesions as confirmed by rEBUS or fluoroscopy. Notably, tissue biopsy was successfully obtained in 97.6% (161/165) patients. This retrospective post-market study reported an overall diagnostic yield of 69.1–77%. Complications of pneumothorax and significant airway bleeding were noted to be 3.6% (6/165) and 2.4% (4/165) respectively. Of the 6 patients who got a pneumothorax, 4 required tube thoracostomy [ ].
The BENEFIT study was a prospective multicenter trial that assessed 54 patients at 5 different institutions. The median lesion size was 23 mm. Navigation success as defined by rEBUS confirmation was noted to be 96% (51/53) of cases. The overall diagnostic yield was estimated to be 74.1% (70% for eccentric and 80.6% for concentric views) Pneumothorax was noted in 2/53 patients (3.7%) and one of those patients required chest tube insertion [ ].
Agrawal conducted a retrospective cohort study of 124 patients. The aim of this study was to elucidate factors associated with the diagnostic accuracy of the Monarch system. In this study, they reported navigation success as measured by rEBUS to be 82% with a diagnostic yield of 77% after 12 months of follow up. Notably this study found similar diagnostic yields for both eccentric (84%) and concentric (85%) views. Median lesion size was 20.5 mm [ ].
1.7
Ion Robotic system
The Ion system consists of the system cart and controller, a laptop with PlanPoint software, and instruments including a flexible, fully articulating catheter, a peripheral vision probe, and flexion biopsy needles. The Ion System has a single ultra-thin, flexible catheter (3.5 mm outer diameter) and a 2.0 mm working channel with a vision probe that is passed through the working channel. The catheter is controlled by a trackball and scroll wheel and can articulate 180° in any direction. Unlike the Monarch system, which uses ENB technology, the Ion Robotic system utilizes shape sensing technology for target navigation. A virtual (3D) airway is constructed by PlanPoint software utilizing a preprocedural thin cut chest CT. At the outset of the procedure, the virtual model is merged with real time patient airway anatomy using the shape sensing catheter. Similarly, a pathway to target is mapped using this information determining an optimal direction given anatomy present and location of target. The vision probe is a key component, as it allows the operator to obtain a bronchoscopy view necessary to confirm the virtual navigation strategy. As with the Monarch platform, once the target is reached rEBUS, fluoroscopy, or CBCT is used to confirm successful target navigation prior to tissue acquisition. The Ion catheter is fixed in position while the vision probe is removed [ ]. A unique feature of the Ion platform is the endoluminal compass which is present on the 3D reconstructed virtual image. This feature can be an invaluable asset to the operator as it can assist in planning how to make the necessary adjustments to traverse the narrowest stretches of the airway. Additionally, a function called “preview path” affords the operator the ability to decouple the virtual image from live view. This can be useful both to virtually preview the path ahead anticipating any sharp or acute angles as well as when the operator encounters significant CTBD. In this scenario, the operator can use the preview function to recalibrate the virtual image with the live image at each branch point [ ].
Yarmus et al. conducted the PRECISION trial in 2020 which compared the Ion robotic system to ultrathin bronchoscopy with rEBUS and ENB with respect to navigating to and biopsing 20 pseudotumor peripherally located lesions in human cadavers. The average diameter was 16.5 mm. Navigational success was noted to be 80% (18/20) with the Ion robotic system as compared to 45% (9/20) and 25% (5/20) with ENB respectively. As with the results of the ACCESS study, the results of this cadaver trial may overestimate the success rates of robotic technology given the fact that CTBD is of much greater significance when dealing with live, breathing tissue [ ].
A retrospective single center trial of 130 patients at a tertiary cancer institution reported navigational success of 98.7%. The median lesion size was 18 mm with 39% of lesions in the periphery. The overall diagnostic yield was 81.7% (93% for concentric and 78.8% for eccentric view. Of note, the Ion system was reported to have a diagnostic yield of 69.5% in lesions <20mmand 70.9% yield for peripheral lesions. Both of these findings are a significant improvement upon what has previously been reported in the literature. Overall complication rate was reported to be 3% (4/130). Two patients had a pneumothorax (1.5%) with one of the two requiring an intervention. The other adverse events included one event of post procedural aspiration pneumonia and one event of hypoxemia that were both managed conservatively [ ].
1.8
Radial EBUS
Radial EBUS is routinely used as the confirmation of target prior to tissue acquisition. Prior to the advent of robotic platforms, the literature consistently reported a large discrepancy between diagnostic yields of concentric versus eccentric views. In a meta-analysis studying rEBUS for PN sampling, the overall diagnostic yield of 7872 lesions was noted to be 70.6%. The difference between concentric (79% yield) and eccentric (52% yield) was significant. When used in combination with the Monarch platform, no significant difference is noted between diagnostic yields of concentric and eccentric views, highlighting the utility of the robotic platform [ ]. One possible explanation for the improvement in diagnostic accuracy in patients with eccentric rEBUS view is the fact that the robotic platform allows for enhanced peripheral visualization and the ability to navigate under direct guidance [ ].
1.9
Robotic bronchoscopy limitations
Both the Monarch and Ion Robotic systems rely in part on a preprocedural CT image to guide navigation to target lesion. This thin cut CT image is obtained at full inspiration which explains why there is divergence between what is seen on CT image and what is seen in a real-life patient. Under general anesthesia, patients have smaller lung volumes, leading to atelectasis. Given this atelectasis, CTBD continues to be an ongoing challenge when attempting to accurately obtain biopsy of these PNs.
Chen et al. described that when comparing a full inspiration CT scan to an end-expiration tidal volume CT scan, the target location differed by 17.6 mm. When sampling such small targets, such a position alteration is significant as the margin for error in these cases is miniscule [ ].
When atelectasis is present during the procedure and no atelectasis was on the baseline CT scan, it is not accounted for in the 3D reconstruction, making the pathway to the target less accurate. This is especially problematic when the target lesion is in the lower lobes where atelectasis tends to be most prevalent and there is more diaphragmatic excursion. Reisenauer et al. described a median CTBD of 10 mm in upper lobes as compared to 21 mm in lower lobes when using the Ion Robotic system. This effect can be mitigated by the confirmatory rEBUS, fluoroscopy, CBCT prior to tissue acquisition to confirm target location [ ]. It is important to note that collapse of distal airways can result in vision loss and impaired robotic navigation, thus adversely impacting yield sensitivity [ ].
1.10
Preoperative anesthetic considerations
Patients scheduled for pulmonary procedures present distinct challenges for anesthesiologists. CT guided TTNA, EBUS, and RAB require a thorough preoperative assessment to determine the patient’s suitability for the procedure, particularly when combined with lung resection surgery. The primary focus of this assessment should encompass an evaluation of the patient’s functional capacity, the presence of adverse effects stemming from prior oncological interventions, identification of paraneoplastic syndromes, and the overall optimization of the patient’s condition.
EBUS and TTNA procedures have rapidly proliferated, however, standardized guidelines for streamlining the preoperative assessment process for anesthesiologists are still lacking. These procedures are typically performed on an outpatient basis, with anesthesia administered at varying depths, including moderate sedation, deep sedation, or general anesthesia. Notably, it is imperative to emphasize that studies have demonstrated that diagnostic efficacy of EBUS remains largely unaffected by anesthetic method [ ]. Consequently, this study and others have demonstrated the most suitable anesthetic regimen should not only be contingent upon individual patient characteristics and procedural specifics but also adhere to intuitional protocols and norms [ ].
While some patients may undergo these procedures without an extensive preoperative evaluation due to their relatively brief anticipated anesthesia duration and low procedural risk, a retrospective analysis involving over 500 patients subjected to EBUS under general anesthesia revealed that pre-existing comorbidities exhibited a stronger correlation with postoperative respiratory complications than the specific choice of anesthesia [ , ]. Thus, it is vitally important to meticulously review recent laboratory tests, electrocardiograms, and imaging studies prior to administering anesthesia. For instance, the presence of hyponatremia could potentially signify Syndrome of Inappropriate Antidiuretic Hormone Secretion (SIADH), or treatment-related adrenal insufficiency linked to immunotherapy, underscoring the critical importance of a comprehensive preoperative assessment.
Additionally, in higher-resource centers, anesthesiologists must assess patients for their readiness to undergo combined procedures under the same anesthetic as a select few centers are beginning to offer this combined hybrid approach. Combined procedures typically include a diagnostic modality such as RAB or EBUS followed by therapeutic lung resection. Lung resection can be performed via Robotic Assisted Thoracic Surgery (RATS), Video Assisted Thoracoscopic Surgery (VATS), or open technique. RAB is especially helpful in localizing small PNs that may not be palpable to the surgical hand. This combined hybrid approach is thought to ease patient anxiety, decrease delays to definitive treatment, and potentially lead to less resource utilization and costs [ ]. The challenge for the anesthesiologist is to ensure proper patient selection as these patients will undergo one lung ventilation (OLV) and laryngeal mask airway (LMA) or single lumen endotracheal tube (SLT) to double lumen endotracheal tube (DLT) exchange to facilitate the combined procedure. Hence, along with a workup of existing comorbidities, the preoperative assessment must include a thorough airway assessment with cardiac and pulmonary function testing to decrease the risk of perioperative complications.
In summation, the management of patients undergoing pulmonary procedures necessitates a thorough preoperative evaluation process tailored to individual patient considerations and procedural prerequisites, especially when lung resection surgery is also planned as a combined approach. This evaluation encompasses an assessment of the patient’s functional capacity, the implications of previous therapeutic interventions, and the presence of underlying comorbidities. Cardiac and pulmonary function testing should also be performed in higher risk patients going for combined procedures to ensure the safest and most efficacious administration of anesthesia.
1.11
Intraoperative anesthetic considerations
The preparation of anesthesiologists for EBUS, TTNA procedures should account for the ability to promptly manage adverse events, not only limited to complications such as hypoxia, hypotension, bleeding, and pneumothorax. Each adverse event carries its own unique set of considerations, which must align with the specific procedure being performed. For instance, patients undergoing TTNA face a heightened risk of pneumothorax compared to RAB, primarily because the proceduralist must traverse the pleura to perform the lung biopsy [ , ]. Likewise, a patent undergoing a core biopsy that has had prior radiation and or the desired pulmonary nodule is adjacent to larger pulmonary vasculature invariably carries a much higher risk of bleeding. Conversely, RAB requires anesthesiologists to consider the impact of their ventilatory strategy on the patient’s hemodynamics, the development of hypoxia, and the diagnostic yield of the procedure.
Furthermore, patients undergoing transthoracic or endobronchial procedures, regardless of the chosen anesthetic, should receive standard ASA monitors with intravenous access sufficient for resuscitation in case of unexpected hemorrhage or hemodynamic shifts. The placement of an arterial line should be based on the patient’s co-morbidities, clinical status, or when a lung resection is planned during the same procedure. General anesthesia necessitates a large-diameter endotracheal tube (8.5 mm or larger) to accommodate a therapeutic bronchoscope. The placement of an endotracheal tube can be accomplished through direct laryngoscopy, video laryngoscopy, or a flexible bronchoscope. EBUS is typically performed with a laryngeal mask airway, allowing evaluation of the glottis and upper trachea. However, an endotracheal tube can also be used, especially in patients with a difficult airway. As expected with a larger endotracheal tube or the use of a supraglottic airway, vocal cord injury, edema, or trauma to surrounding structures can occur with airway manipulation.
Given the shared airway during these procedures, anesthesiologists must maintain vigilance and establish open communication with the proceduralist to expedite necessary interventions and mitigate procedure-related complications. Additionally, patients under general anesthesia with an endotracheal tube for RAB may require transitioning to a LMA if a staging EBUS is performed based on biopsy results. Alternatively, patients undergoing combined procedures with lung resection may require an LMA or SLT to DLT exchange. This presents a unique challenge for airway management, especially in patients with a known difficult airway. Of note, the utility of a bronchial blocker (BB) may be limited depending on the patient’s airway anatomy and procedural laterality. Specifically, although placement of a right-sided BB is easy, maintenance in correct position can be challenging given the short length of the right mainstem bronchus and manipulation by the proceduralist or surgeon intraoperatively. Ultimately, careful planning and preoperative discussions with the proceduralist are essential to minimize the risk of airway complications and to effectively carry out procedural goals.
1.12
Sedation strategies
The choice of sedation for endobronchial procedures should factor in the patient’s comorbidities and procedural requirements. A retrospective study comparing midazolam versus midazolam/propofol for EBUS in 274 patients showed similar complication rates and diagnostic sensitivity [ ]. This underscores the need for a tailored sedation approach that also considers institutional culture and resources. In procedures requiring general anesthesia, total intravenous anesthesia (TIVA) or a combined technique is preferred over the use of a volatile anesthetic alone. This minimizes the risk of awareness under anesthesia due to leaks in the anesthesia circuit from bronchoscopy or airway instrumentation, which can lead to unpredictable volatile anesthetic concentrations. TIVA can be provided with propofol alone or in combination with dexmedetomidine, ketamine, fentanyl, and/or remifentanil. Bispectral Index (BIS) can guide anesthetic depth, particularly when muscle relaxation is employed. The decision to administer paralysis should consider patient safety and the impact of atelectasis and respirations on motion artifact and CTBD [ ].
1.13
Ventilation strategies
While providing sedation or ventilatory support during general anesthesia, atelectasis significantly affects the diagnostic sensitivity of endobronchial procedures. Atelectasis can increase CTBD during navigation and create a “tumor-like” appearance during acquisition, resulting in non-diagnostic biopsies [ , ]. Atelectasis is more likely to occur in dependent lung zones, obese patients, and during prolonged anesthetics [ ]. Patients are also prone to atelectasis immediately following intubation and during intraprocedural breath holds. Conventional interventions to address atelectasis, such as delivering adequate tidal volumes during ventilation, applying positive end-expiratory pressure (PEEP), and performing recruitment maneuvers, are fundamental components of the Lung Navigation Ventilation Protocol (LNVP) and the Ventilatory Strategy to Prevent Atelectasis (VESPA). Both protocols were established to mitigate the impact of atelectasis on diagnostic outcomes during endobronchial procedures [ , ].
Moreover, in patients undergoing combined procedures with lung resection, lung isolation can be obtained with a DLT or BB. Lung ventilation during lung isolation should follow conventional parameters with either volume control or pressure control ventilation to achieve tidal volumes 6–8 ml/kg of predicted body weight (PBW), PEEP 5–10 cm H20, driving pressure less than 14 cm H20, and plateau pressure less than 25 cm H20 to decrease the risk of barotrauma [ ]. Permissive hypercapnia is generally tolerated up to a pH of 7.25 [ ]. Fraction of inspiratory oxygen should be titrated down from 100% as tolerated during OLV to minimize the risk of oxygen toxicity and attenuate the impact of absorption atelectasis. Due to mediastinal shifts, ventilation to perfusion (V/Q) mismatch, and hypoventilation that may also lead to atelectasis, providers may need to occasionally provide recruitment maneuvers during OLV to increase transpulmonary pressures.
Positioning is another crucial consideration when assessing the extent of atelectasis during endobronchial procedures and its impact on diagnostic outcomes. Therefore, RAB is typically performed under general anesthesia with an endotracheal tube before transitioning to an LMA for EBUS. Atelectasis is more prone to develop in the posterior and dependent regions of the lung during general anesthesia. Lin et al. described lateral decubitus positioning with the lesion of interest in the non-dependent lung to reduce atelectasis in posterior peripheral nodules. None of the eleven patients included in their study developed atelectasis in the lung of interest during their procedure, and there were no complications [ , ]. Although the results of this study have limited statistical power, this technique has been adopted in clinical practice. Additionally, prone positioning has been shown to decrease atelectasis, but its clinical use has not been as widely accepted due to safety concerns related to airway management [ ].
1.14
Other considerations
Radiation exposure during robotic bronchoscopy cannot be avoided when CBCT is used to enhance navigation and acquisition. The effective dose of CBCT is approximately equivalent to a low dose CT Chest however, it can increase with the number of image acquisitions. Typically, 1–3 image acquisitions are performed per procedure [ ]. Hence, it is imperative to wear a thyroid shield, eye protection, and a lead apron. Without the appropriate protective equipment, you may be asked to briefly step out of the room during image acquisition, which may be further complicated by the need to provide a breath hold to improve image quality. Clear communication with the proceduralist is critical to avoid inadvertent exposures and optimize patient safety.
1.15
Post procedural considerations
Regardless of sedation choice, patients should be transported to the post anesthesia recovery room with supplemental oxygen and a chest x-ray should be obtained to rule out pneumothorax or pulmonary complications. Patients may require additional opioids, a lidocaine patch, or other non-opioid analgesics to alleviate post operative pain or coughing. Ice chips can also serve as a useful adjunct to relieve post procedural discomfort from coughing. TIVA decreases the chance of patients experiencing postoperative nausea and vomiting (PONV) but antiemetics can be given for prophylaxis intraoperatively or for rescue. Dexamethasone can also be given for PONV prophylaxis, but unfortunately there is limited data on the utility of steroids for lung biopsies.
1.16
Complications and oversight
EBUS and TTNA biopsies can be complicated by post operative hypoxia, pneumothorax, hemoptysis, hypotension, arrythmias, or an escalation of care. In a prospective study of over 1300 patients going for EBUS, only 19 patients experienced a complication, highlighting the overall safety of endobronchial procedures [ ]. Moreover, a meta-analysis of CT guided thoracic biopsies, major complications requiring intervention such as hemothorax, air embolism, needle tract seeding, and death were noted to be under 6% [ ]. Similar complication rates have been shown for RAB [ ]. Regardless of the approach, the anesthesiologist must stay vigilant during the perioperative period to expedite any interventions needed to manage procedural complications.
2
Summary
Reliable and highly accurate diagnostic strategies with low complication rates are needed to facilitate rapid diagnosis and treatment of early-stage lung cancer. Given the promising features of improved reach, stability and navigation, the adoption and usage of these RAB systems has dramatically increased. However, studies comparing whether these newer innovative approaches are truly superior to other advanced bronchoscopic techniques are currently lacking, particularly when considering a combined approach of RAB and if indicated, potential definitive surgery. Likewise, given the increasing utilization of these technologies, studies also need to evaluate further granular aspects such as risk stratification, appropriate patient selection, impact on cost, time to initial diagnosis to definitive treatment, and implications for other adjunctive treatment approaches such as radiation, chemotherapy, and immunotherapy.
Practice points
- •
Lung cancer continues to be one of the most commonly diagnosed cancers and it has the highest cancer-related mortality both in the U.S. and globally
- •
Diagnosis of PNs continues to be challenging for multiple reasons: most lesions are located peripherally, patients tend to be asymptomatic in early disease, inherent built-in delay persist for needed procedures in the diagnostic process
- •
Diagnostic yield continues to improve for advanced bronchoscopy techniques however technical limitations persist
- •
Robotic-assisted bronchoscopy is overcoming some of these limitations enhancing peripheral reach, stability, and resultant improved precision
- •
The primary focus of a preoperative assessment should encompass an evaluation of the patient’s functional capacity, the presence of adverse effects stemming from prior oncological interventions, identification of paraneoplastic syndromes, and the overall optimization of the patient’s condition.
- •
Combined procedures with either EBUS/RAB and lung resection require a workup that includes cardiac and pulmonary function testing
- •
TIVA or a combined technique is recommended for EBUS and RAB to minimize the risk of awareness under anesthesia.
Research agenda
- •
Future studies needed to evaluate if RABs are in fact superior in terms of higher sensitivity to other advanced bronchoscopic techniques
- •
Furthermore granular evaluation of increased costs will need to be looked at as well (especially if combining with other ancillary technologies EBUS, rEBUS, CBCT, fluoroscopy and simultaneous surgical approach with or without robotic surgical approach)
- •
Studies will need to look at the impact of cost for patients getting earlier diagnosis and potentially earlier surgery with fewer anesthetics, need to evaluate unique RAB associated challenges CTBD, atelectasis
- •
Future studies will look at the value proposition for staged versus combined procedures and how they impact the ability for patients to receive other neoadjuvant treatments such as radiation, immunotherapy, or chemotherapy prior to surgery.
Declaration of competing interest
The authors declare that they have no known competing financial interests or personal relationships that could have appeared to influence the work reported in this paper.
Acknowledgements
The authors thank Kate J. Krause, in the Research Medical Library at MD Anderson Cancer Center, for her assistance with the literature search.
References
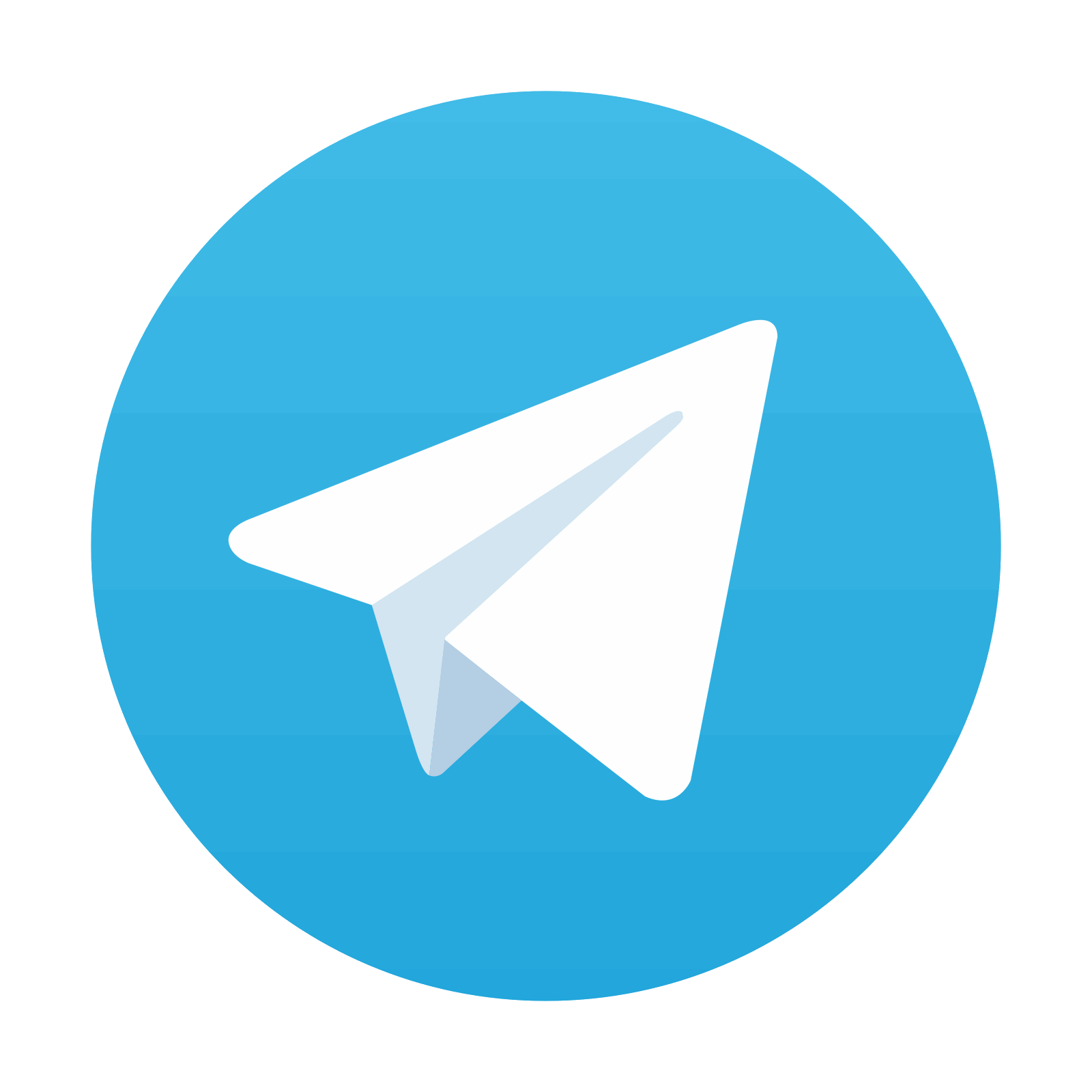
Stay updated, free articles. Join our Telegram channel

Full access? Get Clinical Tree
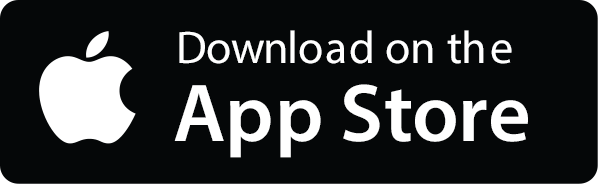
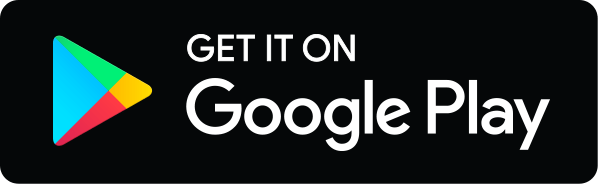
