Respiratory Failure
Micelle J. Haydel
Respiration is the exchange of gases at the level of the alveoli occurring by simple diffusion. Gas in the alveoli has a high concentration of oxygen and a low concentration of carbon dioxide relative to the deoxygenated blood passing through the alveolar capillary bed. Oxygen and carbon dioxide both diffuse freely through alveolar membranes. Oxygen molecules bind to hemoglobin to be off-loaded in the tissues to be used to generate cellular energy, and the by-product CO2 which is then carried back to the alveoli for exhalation. The O2–CO2 gradient at the capillary-alveolar border is constantly maintained, permitting the passive diffusion of gases, while the contraction and relaxation of intercostal muscles actively draws oxygenated gases into the alveoli and then pushes the carbon dioxide–rich gas out of the alveoli. Any part of this process can be altered leading to respiratory distress and failure.
CLINICAL PRESENTATION
Respiratory distress implies that the work of breathing has increased to the point that a patient displays clinical evidence of difficulty in breathing, such as tachypnea, dyspnea, and accessory muscle use. Dyspnea is the sensation of difficulty breathing and is most commonly caused by congestive heart failure, asthma, chronic obstructive pulmonary disease, and pneumonia. Respiratory failure implies that a patient is not able to maintain adequate oxygenation and/or ventilation. Respiratory failure is a clinical diagnosis, although measurements of the partial pressure of oxygen and carbon dioxide are used to assess the patient’s respiratory status. In general, patients with clinical respiratory failure exhibit hypoxemia (PO2 <60) and/or hypercapnea (PCO2 >45) with a concomitant respiratory acidosis (pH <7.34) (1). Symptoms of respiratory distress and failure depend on the chronicity of the respiratory impairment, the presence of comorbidities, and the patient’s physical ability to perform the increased work of breathing.
Hypoxia leads to a physiologic increase in respiratory rate and cardiac output to compensate for the oxygen deficit. As the patient attempts to compensate for the hypoxemia, clinical findings will include tachypnea, accessory muscle recruitment, abdominal paradoxical movements, and intercostal retractions. This increased work of breathing increases a patient’s oxygen consumption to 10 times the resting state (2). Patients with mild–moderate hypoxemia display tachypnea, tachycardia, and hypertension, and may complain of headache. Patients with severe hypoxemia display somnolence, confusion, and seizures. As hypoxemia worsens, patients develop bradycardia and hypotension, leading to permanent hypoxic encephalopathy or death.
Hypercarbia leads to physiologic increase in rate and depth of respirations, and signs and symptoms of hypercarbia depend on the level and rate of increase in PCO2. A high PCO2 can be well tolerated if it develops slowly, minimizing acidemia via renal compensatory mechanisms. Acute noncompensated hypercarbia (PCO2 >80) leads to intracellular acidosis, myocardial depression, dysrhythmias, cerebral vasodilation, and elevated intracranial pressure. Patients with hypercarbia may have agitation, confusion, headaches, seizures, and/or coma, although these neurologic effects of hypercarbia are reversible in patients without concomitant intracerebral injury or stroke (3).
DIFFERENTIAL DIAGNOSIS
Respiratory complaints account for up to 10% of emergency department (ED) visits and dyspnea as a chief complaint has the highest association with inhospital mortality (4). A differential diagnosis can be based on the primary blood gas abnormality, such as hypoxic, hypercapnic, or mixed respiratory failure. In hypoxic respiratory failure, gas exchange is blocked in an area of the lungs due to disease processes such as pneumonia, pulmonary edema, pulmonary embolism, or pneumothorax. Carbon dioxide has a high diffusion capacity and may be unaffected by this “increased shunt,” but will be greatly affected by hypoventilation. Hypercarbic respiratory failure is the inability of gas flow to maintain the necessary off-loading of carbon dioxide at the alveolar level, and is commonly seen in COPD, neuromuscular disease, and opiate toxidromes.
While the most common causes of dyspnea are pneumonia, asthma/COPD, congestive heart failure, and pulmonary embolism (5), several potentially lethal disease states can cause dyspnea and respiratory failure without hypoxemia or hypercarbia on blood gas analysis. When the etiology of dyspnea cannot be explained by the hypoxic/hypercarbic approach, a cognitive map based on four general categories: pulmonary, cardiovascular, neuromuscular, and systemic (Table 73.1), leads the clinician to consider less common causes of respiratory distress, to identify contradictory findings in the H&P, and to determine if more than one disease process is involved. The differential diagnosis of dyspnea in the trauma setting is listed in Table 73.2.
ED EVALUATION
Emergency patients with undifferentiated respiratory distress or impending respiratory failure should be approached in a logical, step-wise manner (Table 73.3) with a goal of:
1. Immediately detect and mitigate life-threatening hypoxemia and hypercarbia.
2. Formulate and initiate critical actions without a complete dataset.
3. Establish a differential diagnosis based first on the most lethal, and then the most likely diagnosis.
Assess Upper Airway
Clinicians must rapidly evaluate the upper airway of patients with dyspnea to rule out the possibility of obstruction, as well as to prepare for the possibility of difficult airway management. Patients with stridor, muffled voice, drooling, neck hematoma, or swelling should be examined for mechanical obstruction due to infection, foreign body, malignancy, or trauma. The presence of obesity, facial hair, poor or absent dentition, and older age are associated with a difficult bag-mask seal. Mallampati classification and measurement of the thyromental and inter-incisor distance can be used to assess for challenges in intubation (6) (see Chapter 1).
TABLE 73.1
Differential Diagnosis of Respiratory Distress and Failure
Assessment of Adequacy of Oxygenation
Oxygen delivery to vital organs is the cornerstone of the management of respiratory failure. Bedside pulse oximetry estimates hemoglobin oxygen saturation (SaO2) by analyzing the transmission of light through a cutaneous vascular bed. Pulse oximetry will overestimate arterial oxygenation in the presence of poor perfusion or carboxyhemoglobin; it will underestimate it in the presence of methemoglobin (7). An arterial blood gas (ABG) analysis, most commonly measures SaO2 indirectly by plotting the PaO2 on a standardized temperature- and pH-adjusted O2–hemoglobin dissociation curve, while ABG analysis using a co-oximeter has the ability to directly measure the concentrations of oxygenated and deoxygenated hemoglobin.
TABLE 73.2
Differential Diagnosis of Dyspnea in the Trauma Setting
TABLE 73.3
Severe Undifferentiated Respiratory Distress
TABLE 73.4
Formula to Predict Acid–Base Compensation
Assessment of the Adequacy of Ventilation
While pulse oximetry is used routinely in most emergency settings, it does not provide information about ventilation, and can be falsely reassuring if the clinician assumes that a normal pulse oximetry reading reflects adequate alveolar ventilation. Noninvasive measures of ventilation (exhaled CO2) have gained widespread use in the ED as an adjunct to pulse oximetry. Bedside capnography provides a numeric and waveform representation of exhaled CO2 (ETCO2), corresponding to arterial PCO2. Patients with a normal baseline (35 to 45 mm Hg) ETCO2 can be expected to have an increase in their ETCO2 during hypoventilation and a decrease during hyperventilation. ETCO2 measures only the exhaled CO2, not the actual PaCO2 and therefore a low ETCO2 can also be seen in the setting of reduced cardiac output and pulmonary embolism.
ABG analysis remains the time-honored gold standard for assessing the adequacy of gas exchange and the acid–base balance. Many diseases are neither acute nor found in isolation, and the ABG is very useful in helping to determine the chronicity of the process, as well as the presence of a mixed respiratory–metabolic disorder. Commonly used acid–base formulas are listed in Table 73.4.
Physical Examination
In the early stages of respiratory distress, vital signs may reflect tachycardia, tachypnea, and hypertension, with the patient demonstrating increased work of breathing and accessory muscle use. As respiratory distress progresses, the patient is unable to speak in complete sentences and must rely on the tripod position to maintain respirations. Increasing hypoxemia or hypercarbia may lead to confusion and combativeness, progressing to a patient who is obtunded or comatose. Depending on the etiology of the respiratory distress and the patient’s physical abilities, the progression from dyspnea to respiratory failure can be gradual or precipitous. In order to prepare for the possibility of a difficult airway, the initial assessment of any patient with respiratory complaints should include assessment of visibility of the soft palate, sternomental distance, and thyromental distance (6) (see Chapter 1).
Diagnostic Studies
An electrocardiogram (ECG) should be obtained in any adult with risk factors for coronary disease or cardiac symptoms. Dyspnea can be a symptom of cardiac as well as pulmonary disease; therefore, the ECG is useful in the evaluation of both adult and pediatric patients with undifferentiated dyspnea.
The measurement of peak expiratory flow rate as a surrogate index for forced expiratory volumes and should be standard practice in the management of patients with respiratory distress due to asthma or COPD. Flow rates <50% of personal best or baseline indicate a severe exacerbation and typically require consideration of inpatient therapy if there is no satisfactory improvement after treatment.
Chest radiography can detect disease processes not identified by physical examination alone, while computed tomography (CT) is useful in patients when lung parenchymal or pleural disease cannot be delineated by plain radiography alone. CT angiogram has largely replaced ventilation–perfusion scanning in detecting pulmonary embolism. Bedside ultrasound can be used to identify pneumothorax, pleural effusion, and pulmonary edema. Ultrasound has more recently been proven to be very useful in the approach to the undifferentiated patient with respiratory distress (8).
Routine complete blood count, electrolytes, glucose, and renal indices should be obtained in patients with respiratory distress of unknown etiology. Other studies that may be indicated when specific diseases are suspected include cardiac enzymes (acute coronary syndromes), brain natriuretic peptide (congestive heart failure), D-dimer assay (thromboembolic disease), coagulation profile, liver enzymes (multisystem involvement), erythrocyte sedimentation rate (autoimmune diseases, pericarditis, myocarditis), and lactate dehydrogenase (LDH) (Pneumocystis carinii pneumonia).
ED Management Approach to the Undifferentiated Patient with Impending Respiratory Failure
1. Exclude upper airway obstruction: Foreign body, hematoma, infection, cancer, angioedema
2. Assess and ameliorate hypoxia
3. Assess ventilation and ameliorate hypercarbia
4. Initiate mechanical ventilation for hemodynamic instability, unstable dysrhythmias, fatigue, progressive respiratory acidosis and/or hypoxemia, loss of airway protective reflexes, alteration in level of consciousness, expected clinical course or lack of response to NIPPV
5. Bedside testing: Portable chest radiograph, electrocardiogram, ultrasound
6. Targeted treatment based on differential diagnosis (Table 73.1)
Oxygen Supplementation
The first step in the management of patients with respiratory distress is the addition of supplementary oxygen, titrating to optimize the individual clinical situation (9). Supplementary oxygen may be provided using a nasal cannula, simple face mask, and nonrebreathing face mask. Nasal cannula provides 24% to 40% oxygen via 1 to 6 L of flow, but high flow rates are poorly tolerated. A face mask provides supplemental O2 concentrations of 40% to 60% when set to 10 to 12 LPM, whereas a nonrebreathing mask with a collapsible bag reservoir of high flow O2 (10 to 15 LPM), optimally delivers O2 concentrations of more than 70%. If these modalities do not provide adequate oxygenation, then mechanical modalities should be initiated to add positive pressure.
Noninvasive Ventilation
Noninvasive positive pressure ventilation (NIPPV) delivers mechanically assisted breaths without the need for intubation. The positive pressure improves oxygenation and gas exchange by increasing both tidal volume and functional residual capacity, and recruiting collapsed alveoli. NIPPV does reduce the work of breathing, but does not “protect the airway” and is therefore contraindicated in patients at high risk for aspiration, those with impaired level of consciousness or need for prolonged ventilator support (Table 73.5). NIPPV has been established in a variety of disease states in the emergency setting, including COPD, cardiogenic pulmonary edema, hypoxemic respiratory failure, and in patients with an acute respiratory exacerbation who do not wish to be intubated (10–12). NIPPV can be used cautiously as a bridge in patients with asthma or pneumonia, but these patients continue to require close monitoring (typically ICU) for deterioration (10). NIPPV failure is not uncommon, and can be predicted during the first hour of use by monitoring closely for continued respiratory distress, or failure to normalize respiratory rate and ABGs (13).
TABLE 73.5
NIPPV—Exclusion Criteria
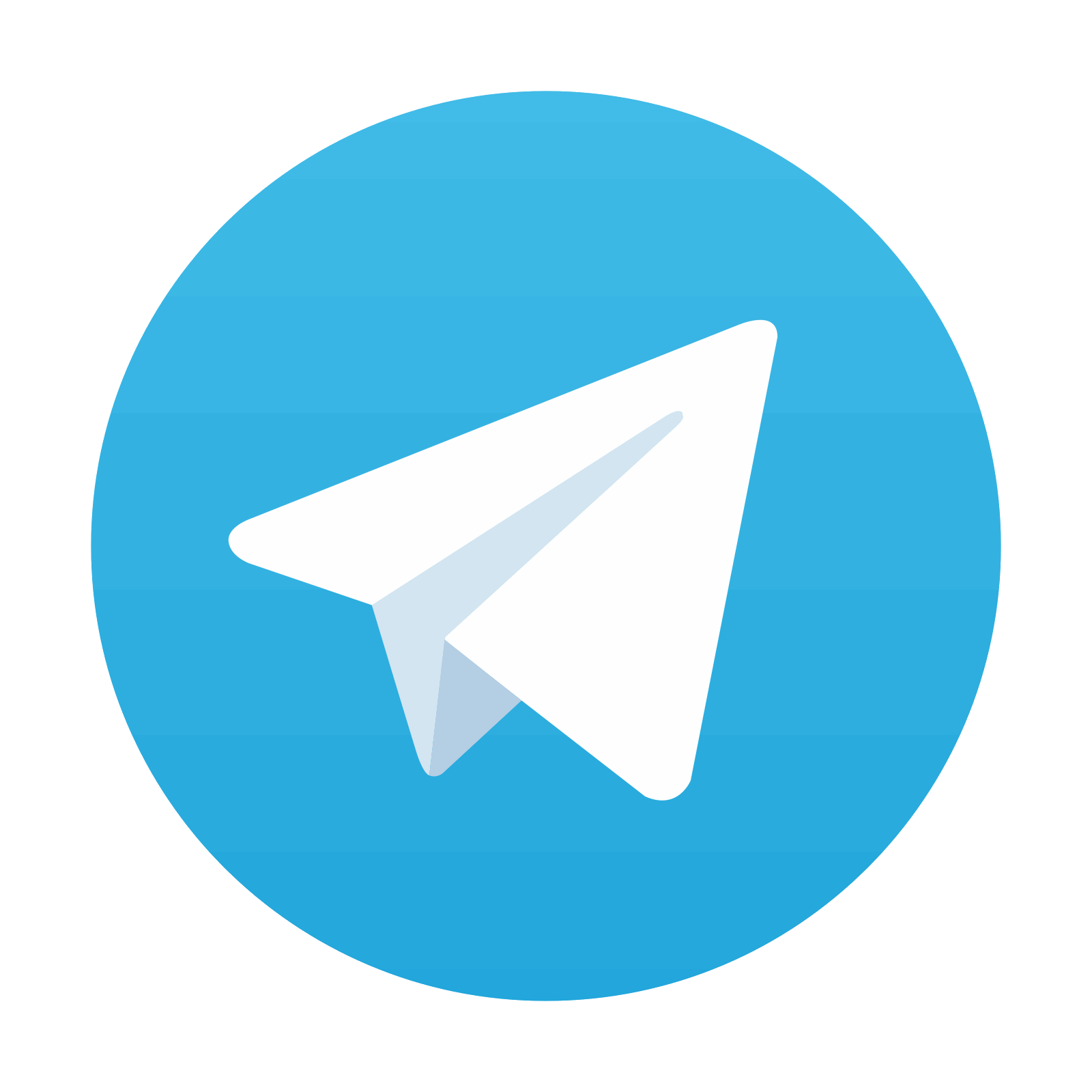