Abstract
The main functions of the kidney are (1) regulation of salt and water balance, (2) toxin and metabolite elimination, (3) electrolyte homeostasis, (4) acid–base balance, and (5) hormone production.
Renal blood flow is directly proportional to the transrenal gradient, the pressure difference between the renal artery and the renal vein. Autoregulation mechanisms maintain constant renal blood flow over a range of blood pressures.
Keywords
clearance, glomerular filtration rate, renin, acid-base balance, electrolyte homeostasis, acute kidney injury
Chapter Outline
Renal Blood Flow and Glomerular Filtration Rate
Toxin and Metabolite Excretion
Risk of Perioperative Acute Kidney Injury
Acute Kidney Injury in Surgical Patients
Assessment and Management of Acute Kidney Injury
Intraoperative Management of Renal Function
Anesthetic Drugs and Impaired Renal Function
Common Perioperative Medications That Impair Renal Function
Perioperative Renal Replacement Therapy
The kidney is a complex multifunctional organ that can be affected by anesthesia and the physiologic alterations of the perioperative period. The main functions of the kidney in the context of anesthesiology include (1) regulation of salt and water balance, (2) toxin and metabolite elimination (including drugs), (3) electrolyte homeostasis, (4) acid–base balance, and (5) hormone production. These functions must be understood and recognized for optimal patient care during and after surgery or intensive care. The anesthetist must understand the kidney’s role in salt and water balance; otherwise, hypovolemia, volume overload, or acute renal failure can complicate surgery. The elimination of toxins and metabolites is important in the perioperative period; this function also includes the elimination of many anesthetic drugs, which can be viewed as exogenous toxins given their narrow therapeutic margin. Electrolyte balance is key for cardiovascular stability and prevention of dysrhythmias. Acid–base balance is vital for proper enzyme and cellular function. Hormones such as renin and erythropoietin play critical roles in blood pressure regulation and red blood cell production, critical concerns during the perioperative period. These many functions of the kidney help maintain homeostasis during periods of changing fluid and electrolyte intake and losses. Fig. 40.1 shows the anatomy and blood supply of the kidneys.

Renal Blood Flow and Glomerular Filtration Rate
Renal Blood Flow
The kidney is the most robustly perfused organ per gram of tissue in the human body, receiving 20% to 25% of cardiac output. Renal blood flow (RBF) is directly proportional to the transrenal gradient , the pressure difference between the renal artery and renal vein, and inversely proportional to the resistance of the renal vasculature. Autoregulation of RBF is accomplished by changing renal vascular resistance as arterial pressure changes, thereby maintaining constant blood flow across a range of mean arterial pressure from 50 to 150 mm Hg in normotensive people.
While global RBF is relatively constant, its distribution within the kidney is quite heterogeneous. The renal cortex and renal cortical nephrons receive 90% of RBF while the renal medulla and its juxtamedullary nephrons receive only about 10%. This great disparity in regional blood flow makes the renal medulla sensitive to ischemic injury. RBF is calculated by determining both the clearance of a given substance from the plasma and the renal plasma flow (RPF).
Renal Clearance
The renal clearance of a substance is the volume of plasma completely cleared of the substance per unit time.
C = U × V / P ,
Renal Plasma Flow
RPF is calculated by the clearance of para-aminohippuric acid (PAH), as at low concentrations this compound is completely cleared from the plasma by renal tubular filtration and secretion in a single pass.
RPF = C PAH = U PAH × V / P PAH ,
RBF (in mL/min) is determined via the following equation:
RBF = RPF / 1 − hematocrit .
Glomerular Filtration Rate
Glomerular filtration rate (GFR) is measured by the clearance of inulin, a fructose polysaccharide that is readily filtered but not resorbed or secreted by the renal tubule, therefore being directly proportional to GFR.
GFR = U inulin × V / P inulin = C inulin ,
Normal GFR is about 120 mL/min in men and 100 mL/min in women. GFR can also be estimated with blood urea nitrogen (BUN) and plasma creatinine. BUN and plasma creatinine increase as GFR decreases. Notably, GFR decreases with age although plasma creatinine remains relatively constant owing to a decrease in muscle mass. Creatinine clearance is less accurate than inulin clearance but much more practical to measure.
GFR ≈ U creatinine × V / P creatinine = C creatinine ,
The filtration fraction (FF) is the fraction of RPF that is filtered across the glomerular capillaries and is normally about 0.20. Therefore, 20% of the RPF is filtered, leaving 80% of the RPF to leave the glomerulus via the efferent arterioles, making up the peritubular capillary circulation.
FF = GFR / RPF ,
Blood flow to the glomerulus is regulated by the afferent and efferent arteriolar vascular tone, which adjusts glomerular filtration pressure. Afferent arteriolar dilation or efferent arteriolar constriction increases the FF and GFR. This autoregulatory mechanism is capable of maintaining GFR across a wide range of blood pressures and is achieved, in part, by the juxtaglomerular apparatus (see later discussion).
If blood pressure falls, there is a concomitant reduction in afferent arteriolar pressure with a fall in RBF and in filtered solute. This triggers release of renin in the juxtaglomerular apparatus. Renin, a selective protease, cleaves angiotensinogen to angiotensin I, which is subsequently cleaved to angiotensin II by angiotensin-converting enzyme (ACE) in the lungs. Angiotensin II causes thirst (and water intake), vasoconstriction, and Na + and water retention (via aldosterone), all of which increase blood volume and pressure, renal perfusion pressure, and thus RBF. Additionally, the kidney responds to low levels of catecholamines circulating when blood pressure drops by preferential efferent arteriolar vasoconstriction, which acts to maintain GFR.
Age-Related Renal Changes
RBF decreases by 10% per decade of life after age 50 years; parallel changes occur in the kidney’s ability to handle acid and Na + . Creatinine becomes increasingly unreliable as a marker of GFR in older adults owing to loss of muscle and malnutrition. The National Kidney Foundation recommends using the Cockcroft-Gault formula for calculation of GFR.
GFR men = 140 − age ( years ) × weight ( kg ) / serum creatinine ( mg / dL ) × 72
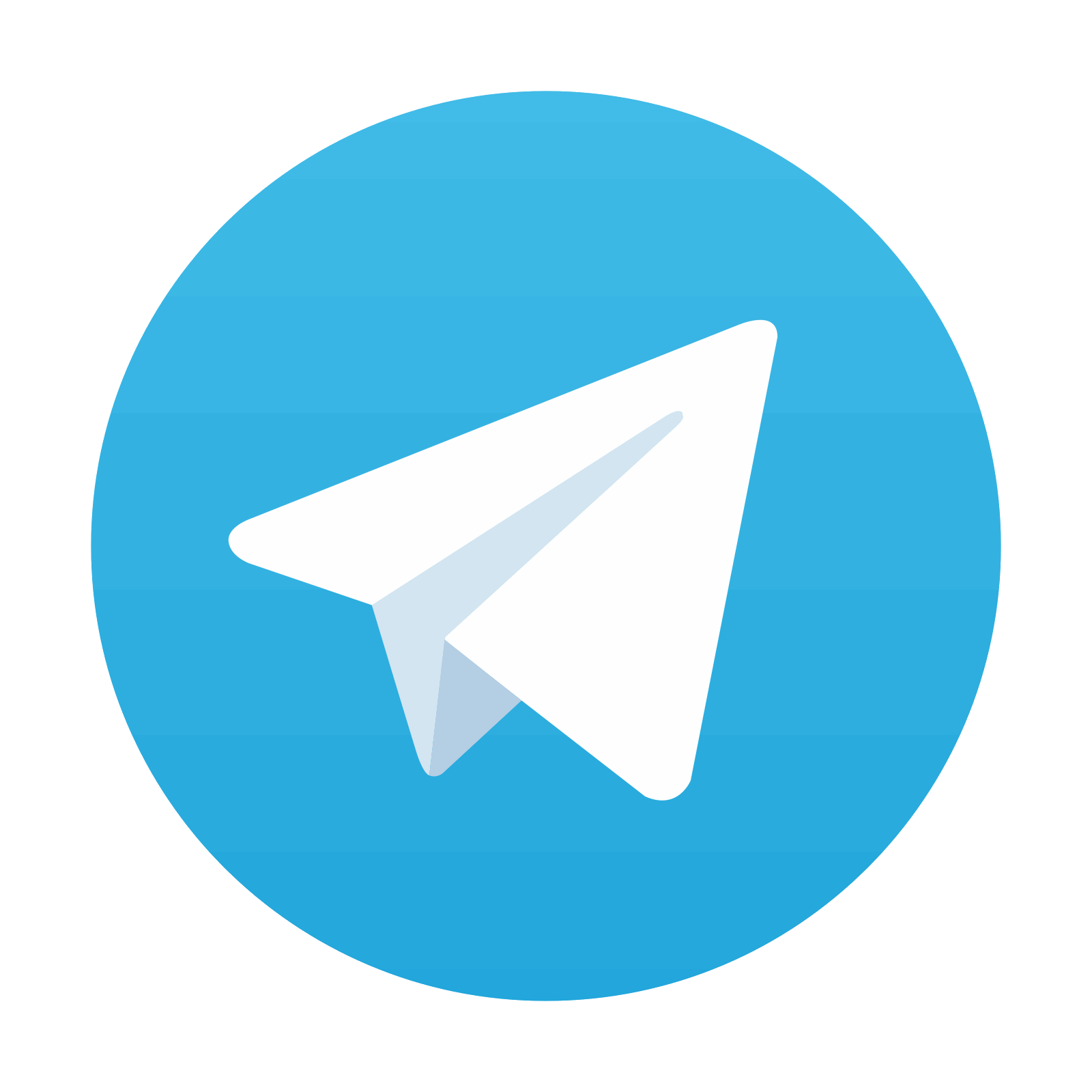
Stay updated, free articles. Join our Telegram channel

Full access? Get Clinical Tree
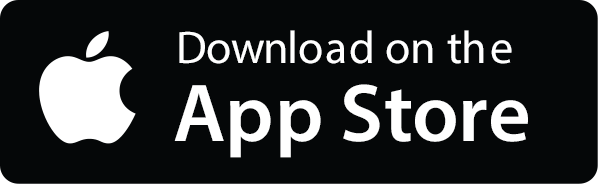
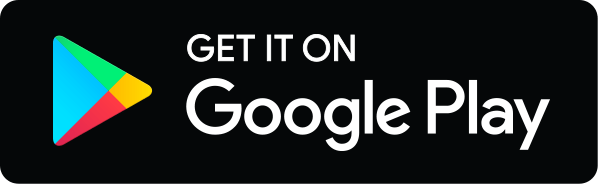