KEY POINTS
1. The incidence of overt stroke is 2% to 6% for closed-chamber cardiac procedures. For open-chamber procedures, the incidence of stroke is increased to 4.2% to 13%.
2. Risk factors for early stroke have been found to be advanced age, duration of cardiopulmonary bypass (CPB), high postoperative creatinine, and extensive aortic atherosclerosis, while delayed stroke was associated with female gender, postoperative atrial fibrillation, cerebrovascular disease and requirement for inotropic support.
3. Within the first postoperative week, up to 83% of all patients undergoing bypass surgery using CPB demonstrate a degree of cognitive dysfunction. Efforts to mitigate against early postoperative cognitive dysfunction are warranted since early postoperative cognitive issues are in part reflective of subclinical brain injury.
4. Watershed lesions are commonly due to profound hypotensive episodes but may also be the result of cerebral embolism. Embolization and hypotension acting together may magnify CNS injury.
5. The etiology of CNS damage associated with embolization is from multiple sources that are patient related, procedure related, and equipment related.
6. Leukocytosis is associated with a higher risk for ischemic stroke. The results strongly implicate inflammation and white cell activation as etiologic factors in both the extent and severity of perioperative cerebral events.
7. α-Stat blood gas management, which maintains a normal transmembrane pH gradient and maintains cerebral autoregulation of blood flow, should be used in adult patients undergoing bypass. This modality may help prevent cerebral embolization. See Table 24.7 for additional evidence-based practice guidelines.
8. A wide variety of autoregulation thresholds occurs even with α-stat blood gas management and is likely a consequence of increased age and cerebrovascular disease. There is an emerging consensus during normothermic and tepid bypass to maintain MAP greater than 70 mm Hg. See Table 24.8 for other management strategies to decrease CNS brain injury.
I. Central nervous system (CNS) dysfunction associated with cardiac surgery
A. Overview. Despite the continuing improvements in surgical and cardiopulmonary bypass (CPB) techniques during cardiac surgery, stroke remains a devastating postoperative complication for patients and their families. In a recent study in which 1,800 patients with three-vessel or left mainstem coronary artery disease were randomized to percutaneous intervention (PCI) or conventional coronary artery bypass (CAB) surgery, there was no difference in mortality at 1 yr but a significantly lower incidence of primary composite endpoint of major adverse cardiac or cerebrovascular event in CAB (12.4%) versus PCI (17.8%) patients [1]. However, while the overall outcome should argue strongly in favor of CAB surgery, the stroke rate was significantly higher in CAB (2.2%) than PCI (0.6%) patients. Given the relatively equivalent risk factors between PCI and CAB patients in this study, the mechanism of perioperative stroke must be better understood if we are to further reduce the risk of CNS morbidity related to cardiac surgery. This chapter will review the current incidence and risk factors for brain damage in cardiac surgery and will outline strategies aimed at protection of the brain during cardiac surgical procedures.
1
B. Stroke incidence. In most series reported to date, the incidence of clinically apparent neurologic injury or overt stroke is 2% to 6% for closed-chamber cardiac procedures (e.g., CAB surgery). Up to 25% to 65% of strokes after CAB surgeries are bilateral or multiple, suggestive of an embolic etiology [2]. For open-chamber procedures (e.g., valve surgery), the reported incidence varies from 4.2% to 13%, which could be related to increased risk of embolization, increased hemodynamic instability, or prolonged CPB time. However, more subtle neurologic changes, such as development of primitive reflexes (e.g., snouting, palmomental reflex), visual field defects, and subtle motor-sensory abnormalities, can be demonstrated in the early postoperative period in up to 61% of all patients undergoing CPB. By 2 mos postoperatively, the prevalence of such new subtle neurologic dysfunction decreases to about 20% and persists for at least 1 yr [3].
2
C. Early versus delayed stroke. In considering the incidence of perioperative stroke, it is apparent that distinguishing stroke as early (i.e., neurologic deficit apparent on emergence from anesthesia) or delayed (i.e., neurologic deficit developing after awakening from anesthesia) is important to better discriminate etiology and assess potential risk-reduction strategies, as it is apparent that only approximately 50% of perioperative strokes present during the first 24 hrs postsurgery [3], and tend to be more severe with a higher permanent deficit and a higher impact on mortality [3–5]. The higher mortality could be related to sicker patients, or that stroke is just one manifestation of other concomitant embolic/hypoperfusion-mediated complications. Stroke can also have a long-term effect on mortality (Fig. 24.1). In a prospective study on CAB patients, the adjusted survival rates at 1, 5, and 10 yrs were 94.1%, 83.3%, and 61.9% among patients free from stroke versus 83%, 58.7%, and 26.9% for those suffering perioperative stroke [6]. Risk factors for early stroke have been found to be advanced age, duration of CPB, high preoperative creatinine, and extensive aortic atherosclerosis, while delayed stroke was associated with female gender, postoperative atrial fibrillation, cerebrovascular disease, and requirements for inotropic support [5]. Delayed but not early stroke was associated with long-term mortality.
Figure 24.1 Effect of stroke on 10-yr survival after CAB graft. The crude annual incidence of death was 18.1/100 person-years among patients with strokes and 3.7/100 person-years among patients without strokes (p <0.001). (From Dacey L J, Likosky DS, Leavitt BJ, et al. Perioperative stroke and long-term survival after coronary bypass graft surgery. Ann Thorac Surg. 2005;79:532–536, with permission.)
D. Cognitive dysfunction. It has been demonstrated that within the first postoperative week, up to 83% of all patients undergoing CAB surgery using CPB demonstrate a degree of cognitive dysfunction. Of these patients, 38% have symptoms of intellectual impairment and 10% are considered to be overtly disabled. Concentration, retention, and processing of new information and visuospatial organization are the most frequently affected domains. At 5-yr follow-up, more than 35% of CAB patients exhibit some degree of neuropsychologic dysfunction [7]. Variable definitions, different measurement techniques, and different intervals of postoperative cognitive testing confound this issue, however, giving rise to reported incidences of perioperative cognitive decline that vary from 4% to 90%. Additional confounders include the variability in performance to repeated neuropsychometric testing even in healthy subjects, and an innate decline in cognitive function associated with both aging and the various comorbidities found in cardiac surgical patients. The challenge lies in discerning whether a specific event, for example, cardiac surgery, is causal or coincidental to deterioration in cognitive function. On the basis of more recent studies, the consensus is that longer-term cognitive dysfunction has a similar incidence whether patients undergo cardiac surgery with or without use of CPB, or instead undergo PCI or are managed medically, with the implication that aging and progression of underlying atherosclerosis and related comorbidities are of most significance [8].
E. Comparison groups. The incidence of new postoperative CNS dysfunction in CAB patients has been compared with that of patients undergoing major abdominal vascular or thoracic surgical procedures. Most of these patients usually have concomitant disease including hypertension, diabetes mellitus, diffuse atherosclerosis, and chronic lung disease. After adjusting for identified risk factors, patients undergoing any surgical procedure have been found more likely to suffer a cerebrovascular accident (CVA) than nonoperated controls with an odds ratio of 3.9. Even after excluding high-risk surgery (cardiac, vascular, and neurologic), the odds ratio is 2.9, which suggests the perioperative period itself predisposes patients to stroke. This observation is of particular relevance in considering the role of inflammatory processes and the salutary effect of statins as discussed below.
Studies on patients undergoing CAB surgery have demonstrated minimal difference in long-term cognitive function between patients undergoing on-pump versus off-pump procedures [9]. However, in general, it does appear that compared with other noncardiac surgical groups, the incidence of early postoperative cognitive dysfunction is higher in CAB patients, and since new ischemic lesions on magnetic resonance imaging (MRI) studies in valve surgery patients have been correlated with early postoperative cognitive dysfunction [10], as has intraoperative cerebral oxygen desaturation and early postoperative cognitive dysfunction in CAB patients, it does appear as though efforts to mitigate against early postoperative cognitive dysfunction are warranted since early postoperative cognitive dysfunction is in part reflective of subclinical brain injury.
3
F. Risk factors. Table 24.1 shows risk factors for both stroke and cognitive dysfunction. Risk factors have been pooled into various risk prediction models, which, while useful to compare patient groups, are still not predictive of a particular individual’s outcome, although the presence of key risk factors may help in deciding the best procedure for a particular high-risk patient (i.e., surgery vs. angioplasty or valvuloplasty). Specific risk factors (i.e., aortic atherosclerosis, recent stroke) should prompt further preoperative investigations (e.g., carotid scanning, modification of intraoperative management) and may even suggest a change in surgical approach (i.e., off-pump CAB [OPCAB] with no instrumentation of the aorta) to minimize the potential for neurologic complications.
Table 24.1 Risk factors for neurologic complications in cardiac surgery
II. Cerebral physiology
A. Cerebral autoregulation. In normal subjects, cerebral blood flow (CBF) remains constant at 50 mL/(100 g·min) over a wide range of mean arterial pressure (MAP) from 50 to 150 mm Hg. This autoregulatory plateau reflects the tight matching between cerebral metabolic rate for O2 (CMRO2) and CBF, mediated in part by endothelium-derived relaxing factor (EDRF-nitric oxide [NO]). With decreased metabolic activity resulting from certain anesthetics or hypothermia, lowered CMRO2 produces a resultant reduction in CBF and establishment of a lower autoregulatory plateau. It is apparent and should be considered that rather than a single cerebral autoregulatory curve, there are instead a series of autoregulatory curves. Each autoregulatory curve represents a differing set of metabolic conditions of the brain (e.g., normal metabolic activity) at 37°C versus lowered metabolic activity at 28°C. The autoregulatory plateau is a manifestation of intact cerebral flow and metabolism coupling, and it varies with metabolic rate.
With intact autoregulation, adequate substrate (blood flow) can be delivered at a lower perfusion pressure during conditions of lowered metabolic rate (e.g., anesthesia, hypothermia) in the absence of cerebral vasodilators (Fig. 24.2). Cerebral autoregulation is lacking in patients with diabetes mellitus and appears to be lost during deep hypothermia (e.g., less than 20°C) and for several hours after deep hypothermic circulatory arrest (DHCA). This results in pressure-passive CBF; in these instances, hypotension may entail increased risk for cerebral hypoperfusion. Similarly, in patients with chronic hypertension, cerebral autoregulation has been reset and higher perfusion pressures might be needed during CPB. In these circumstances, an uncoupling of CBF and cerebral metabolism could be related to neurocognitive decline [11].
It is notable that most of the initial studies on CBF and CMRo2 specifically excluded patients with overt cerebrovascular disease while more recent studies which included elderly patients and those with previous CVA have indicated a striking variability in the autoregulatory threshold [12]. Using transcranial Doppler (TCD) or cerebral near-infrared spectroscopy (cNIRS), lower limits of cerebral autoregulation ranging from 45 to 80 mm Hg have been demonstrated, and in a study of 127 adult patients during CPB, a correlation has been made between loss of cerebral autoregulation during rewarming and postoperative neurologic events [13,14].
Figure 24.2 Cerebral autoregulatory curves during normothermia and hypothermia. The upper curve demonstrates a higher CBF autoregulatory plateau that is appropriate for the higher CMRO2 in the awake state, versus a lower CBF plateau during hypothermia. With maximal cerebral vasodilation, lower CPP results in lower CBF that is appropriate at a lower CMRO2 (hypothermia), but not at higher CMRO2. (From Murkin JM. The pathophysiology of cardiopulmonary bypass. Can J Anesth. 1989;36:S41–S44, with permission.)
B. pH management. There is an inverse relationship between solubility of respiratory gases and blood temperature. With cooling of blood, CO2 partial pressure (PaCO2) decreases and arterial pH (pHa) increases, producing an apparent respiratory alkalosis in vivo. To compensate for this condition during hypothermic CPB, total CO2 must be increased by addition of exogenous CO2 to the oxygenator, known as pH-stat pH management.
1. a-Stat maintains pHa 7.4 and PaCO2 40 mm Hg at 37°C without addition of exogenous CO2. Intracellular pH is primarily determined by the neutral pH (pHN) of water. Since pHN becomes progressively more alkaline with decreasing temperature, intracellular pH becomes correspondingly more alkaline during hypothermia. Since this intracellular alkalosis occurs in parallel with the hypothermia-induced increased solubility of CO2 and increased blood pH, the normal transmembrane pH gradient of approximately 0.6 units remains unchanged, thus preserving optimal function of various intracellular enzyme systems. This preservation of normal transmembrane pH gradient is the crux of a- stat pH theory, and, in fact, we function in vivo according to α-stat principles. Since different tissues have differing temperatures (e.g., exercising muscle at 41°C vs. skin at 25°C), they also will have correspondingly different pHa values (e.g., 7.34 vs. 7.6, respectively), although the net pHa at 37°C will be 7.4. a-Stat management acknowledges the temperature dependence of normal pHa and strives to maintain a constant transmembrane pH gradient by maintaining PaCO2 at 40 mm Hg and pHa at 7.4 as measured in vitro at 37°C. For this strategy during CPB, total CO2 is kept constant by not adding exogenous CO2 and thus not compensating for increased solubility of CO2. Blood samples measured at 37°C will show pHa 7.4 and PaCO2 40 mm Hg, but those same samples measured at 28°C would have pHa 7.56 and PaCO2 26 mm Hg (Fig. 24.3).
Figure 24.3 Contrasting arterial blood gas values as seen in vitro at 37°C or in vivo at 28°C when using α-stat or pH-stat management. Using pH-stat, laboratory values in vitro would be pHa 7.26 and PaCO2 56 mm Hg, whereas temperature-corrected values in vivo would be pHa 7.4 and PaCO2 40 mm Hg. If α-stat were used, laboratory values in vitro would be pHa 7.4 and PaCO2 40 mm Hg, whereas temperature-corrected values in vivo would be pHa 7.56 and PaCO2 26 mm Hg.
2. pH-stat management involves addition of exogenous CO2 to maintain PaCO2 40 mm Hg and pHa 7.4 when corrected for the patient’s body temperature in vivo. Until the mid-1980s, pH-stat management was generally the most common mode of pH management during moderate hypothermic CPB. Since it is a potent cerebral vasodilator, such increases in total PaCO2 associated with pH-stat have been shown to produce cerebral vasodilation, impairing cerebral flow and metabolism coupling and producing loss of cerebral autoregulation (Fig. 24.4). There is evidence that pH-stat management can increase the incidence of postoperative cognitive dysfunction when CPB duration exceeds 90 min [15]. This likely reflects both increased delivery of microemboli into the brain resulting from CO2-induced vasodilation, and impairment of regional cerebral autoregulation. It is notable that in recent studies even with use of a-stat, a wide variability of autoregulatory threshold has been found likely as a consequence of the increased age and presence of overt cerebrovascular disease in current surgical populations [12,14].
Figure 24.4 Linear regression analysis of CBF and CMRO2, or CPP, for patients managed using α-stat (non–temperature-corrected) or pH-stat (temperature-corrected) management during moderate hypothermia (28°C). With pH-stat (A), there is no correlation between CBF and CMRO2, demonstrating loss of cerebral flow and metabolism coupling, whereas with α-stat (C) there is a highly significant (p <0.005) correlation. CBF significantly (p <0.002) correlates with CPP using pH-stat (B), reflecting pressure-passive CBF and loss of autoregulation, whereas with α-stat (D), CBF is independent of CPP. (From Murkin JM, Farrar JK, Tweed WA, et al. Cerebral autoregulation and flow/metabolism coupling during cardiopulmonary bypass: The influence of PaCO2. Anesth Analg. 1987;66:825–832, with permission.)
III. Etiology of CNS damage
A. Embolization. In the context of CPB, focal ischemia is most often a consequence of isolated cerebral arteriolar obstruction by a particulate or gaseous embolus. Emboli vary in size, nature (particulate vs. gaseous), and origin (patient vs. equipment). Embolus factors influencing potential for damage include size, solubility, viscosity, and buoyancy relative to blood. Vessel diameter, anatomical location, and inflammatory responsivity influence tissue vulnerability. Open-chamber procedures generally entail greater risk of embolization than closed-chamber procedures. Calcific or atheromatous macroembolic debris from the ascending aorta or aortic arch appears to be a prime factor in the production of clinical stroke syndromes. It was formerly thought that microembolic elements, either gaseous or particulate, produced cognitive dysfunction. Studies from beating-heart surgery in which CPB is avoided, despite a much lower incidence of embolic events, appear to have a relatively similar incidence of long-term cognitive dysfunction when compared to CAB using conventional CPB. Microgaseous emboli, the numbers of which are greatly reduced by avoidance of CPB, paradoxically appear to be relatively less injurious than otherwise predicted. The use of heparin anticoagulation in cardiac surgical patients has been demonstrated to ameliorate some of the overt effects of cerebral gas emboli [16].
Areas of brain localized at the boundary limits of major cerebral arteries (e.g., anterior and middle, or middle and posterior cerebral arteries, or superior and posterioinferior cerebellar arteries) are known as arterial boundary zones or watershed zones (Fig. 24.5), and these can manifest as isolated lesions as a consequence of transient global ischemia (see following sections). Although they are commonly due to profoundly hypotensive episodes, watershed lesions are not pathognomonic of a hypotensive episode and may be the result of cerebral emboli. Embolization and hypoperfusion acting together can play a synergistic role and either cause or magnify CNS injury in cardiac surgical patients.
4
Figure 24.5 Hatched areas showing the most frequent locations of boundary area, or watershed zone infarcts in the brain, situated between the territories of major cerebral or cerebellar arteries. (From Torvik A. The pathogenesis of watershed infarcts in the brain. Stroke. 1984;2:221–223, with permission.)
1. Detection of emboli
a. Brain histology. Isolated areas of perivascular and focal subarachnoid hemorrhage, neuronal swelling, and axonal degeneration are seen with higher frequency in the brains of patients dying after cardiac surgery than after non-CPB major vascular surgery. After surgery using unfiltered CPB circuits, fibrin and platelet emboli and calcific and atheromatous debris were seen frequently in small arterioles and capillary beds. Small cerebral capillary and arterial dilatations (SCADS) have been demonstrated histologically, occurring in nonsurvivors after proximal aortic instrumentation after either CPB or coronary angiography. These SCADS are increasingly believed to be due in part to lipid microemboli from usage of unprocessed cardiotomy suction blood.
b. Intraoperative emboli detection. Intraoperative fluorescein retinal angiography has demonstrated that extensive retinal microvascular embolization occurs during CPB. The incidence and extent of retinal obstruction are much greater with bubble than with membrane oxygenators, despite use of 40 μm arterial line filters. Use of TCD insonation enables assessment of blood flow perfusion characteristics through the middle cerebral artery (MCA). TCD insonation permits measurement of blood flow velocity and detection and quantification of emboli, though discrimination of gaseous from particulate emboli remains unreliable. Proximal aortic instrumentation and initiation of CPB have been identified as particularly embologenic events. After open-chamber surgery, cerebral emboli are detected as the heart fills and begins to eject, underscoring the importance of meticulous deairing techniques (see the following sections).
5
2. Sources of emboli
a. Patient-related sources
(1) Aortic atheroma. Atheromatous debris can be embolized during aortic clamping or cannulation. Intraoperative aortic ultrasonography using either transesophageal echocardiography (TEE; high sensitivity, low specificity) or epiaortic scanning (EAS) (high sensitivity, high specificity) enables visualization of aortic wall and can be used to guide cannulation sites. Ultrasonography has demonstrated that plaque may fracture or shear off and embolize during CPB as a consequence of trauma from aortic clamping and cannulation or from blood “jetting” from the aortic cannula or may result in intimal flap formation with potential for delayed postoperative embolization [17]. Using EAS, Ura et al. compared images before and after CPB in 472 patients undergoing cardiac surgery, and noted new lesions in the ascending aortic intima in 16 patients (3.4%) following decannulation [17]. In 10 patients, 3 of whom suffered postoperative CVA, the new lesions were severe with mobile lesions or disruption of the intima, of which 6 were related to aortic clamping and the other 4 to aortic cannulation. Only the maximal thickness of the atheroma near the aorta manipulation site was a predictor of new lesions. If the atheroma was 3 to 4 mm, the incidence of new lesions was 11.8% and was as high as 33.3% if the atheroma was >4 mm. As such, embolization of plaque or thrombus from such intimal fractures may explain one mechanism of delayed stroke cited above. Proximal aortic atherosclerosis is thus a significant risk factor for neurologic injury.
(2) Intraventricular thrombi. During closed-chamber procedures in patients with recent mural thrombi, manipulation of the heart can dislodge thrombi that embolize once the heart begins to fill and eject.
(3) Valvular calcifications. Valve surgery, particularly valve replacement surgery, is associated with increased risk of CVA resulting from embolization of intracavitary valve debris.
(4) Postoperative atrial fibrillation. Early-onset atrial fibrillation is associated with a variety of adverse outcomes and has been strongly linked to increased perioperative stroke risk, and is particularly associated with increased risk of delayed-onset postoperative stroke [5]. Even transient new-onset atrial fibrillation is associated with increased risk of 30-day and 1-yr cardiovascular events composed of stroke, cardiac death, and myocardial infarction (MI). In cardiac surgical patients a decreased incidence of atrial fibrillation has been associated with perioperative statin therapy as discussed below. Increased efforts should thus be aimed in part at reducing even transient new-onset postoperative atrial fibrillation.
b. Procedure-related sources
(1) While open-chamber procedures (e.g., septal repair, ventricular aneurysmectomy, valve surgery) expose the arterial circulation to air or particulate debris, closed-chamber procedures also can be associated with ventricular air. Use of a ventricular vent, particularly if active suction is applied and the heart is empty, produces localized subatmospheric pressure at the vent tip within the left ventricle (LV) and cause air to be entrained retrograde from the vent insertion site (usually through the superior pulmonary vein) into the LV. Use of TEE can assist in visualization and guide the removal of residual intracavitary air (see following discussion). Inadvertent opening of the left atrium (LA) or LV while the heart is beating also caused rapid air entrainment and increased potential for cerebral emboli.
(2) Aortic cannulation and clamping are associated with cerebral embolization, particularly in the presence of extensive aortic atherosclerosis.
(3) Duration of CPB is an independent risk for postoperative brain dysfunction. After 90 min of CPB, the incidence of cognitive dysfunction is increased compared to CPB of shorter duration. It is important to note that duration of CPB may be increased by factors (e.g., extensive atherosclerotic disease), which may independently contribute to neurologic injury [3,4].
c. Equipment-related sources
(1) Incorporation of a 25 μm filter into the aortic inflow line effectively reduces cerebral embolic load and has been shown to decrease the incidence of postoperative cognitive dysfunction.
(2) Membrane oxygenators give rise to markedly fewer gaseous microemboli than bubble oxygenators, but this does not entirely eliminate the risk of air emboli. Similarly, air entrained into the venous side of a membrane oxygenator, or gaseous emboli resulting from drug administration via injection directly into CPB circuitry can transit the membrane and appear in the arterial inflow line, despite use of arterial line filters.
(3) Use of 20 to 40 μm filters in the cardiotomy return line prevents particulate debris from the operative site from entering the CPB circuit. Use of cardiotomy blood washing techniques (cell saver) is associated with reduced amounts of cerebral lipid microemboli but has also been shown to result in greater transfusion requirements and has not been shown to consistently improve CNS outcomes.
(4) Use of nitrous oxide (N2O) before commencement of CPB has been associated with increased evidence of ischemic damage, likely because residual N2O increases the size of any microgaseous emboli in the cerebral circulation. This is especially true for several hours after CPB when high fractional inspired oxygen (FIO2) should be used to minimize the size of residual gaseous microemboli.
B. Hypoperfusion
1. Watershed areas. Collateral perfusion of the brain can occur via extracerebral anastomoses (primarily through the circle of Willis) or by way of intracerebral anastomoses between major cerebral arteries, known as arterial boundary zones (watershed zones; Fig. 24.5). Rapid severe hypoperfusion can produce ischemic lesions within these boundary zones found at the territorial limits of the major cerebral arteries. The most frequently affected area is the parieto-occipital sulci located at the limits of the anterior, middle, and posterior cerebral arteries. Despite a global ischemic stress, these watershed lesions may be focal and asymmetrical. Placement of electroencephalogram (EEG) electrodes using a parasagittal montage (see following) may allow increased sensitivity for border zone ischemia detection.
2. Cerebral perfusion pressure (CPP). During moderate hypothermia (28 to 30°C) using α-stat pH management, autoregulation is preserved in patients without overt cerebrovascular disease over the CPP range from 20 to 100 mm Hg. However, studies in elderly cardiac surgical patients and those with cerebrovascular disease indicate a wide variability of lower limit of autoregulation between 45 and 80 mm Hg [12]. Additionally, there are several conditions in which autoregulation may be lost (Table 24.2). With profound hypothermia (15 to 20°C), there appears to be loss of autoregulation as a result of hypothermia-induced vasoparesis, while diabetic patients have been shown to have impaired cerebral autoregulation even at moderate hypothermia.
Table 24.2 Factors associated with loss of cerebral autoregulation
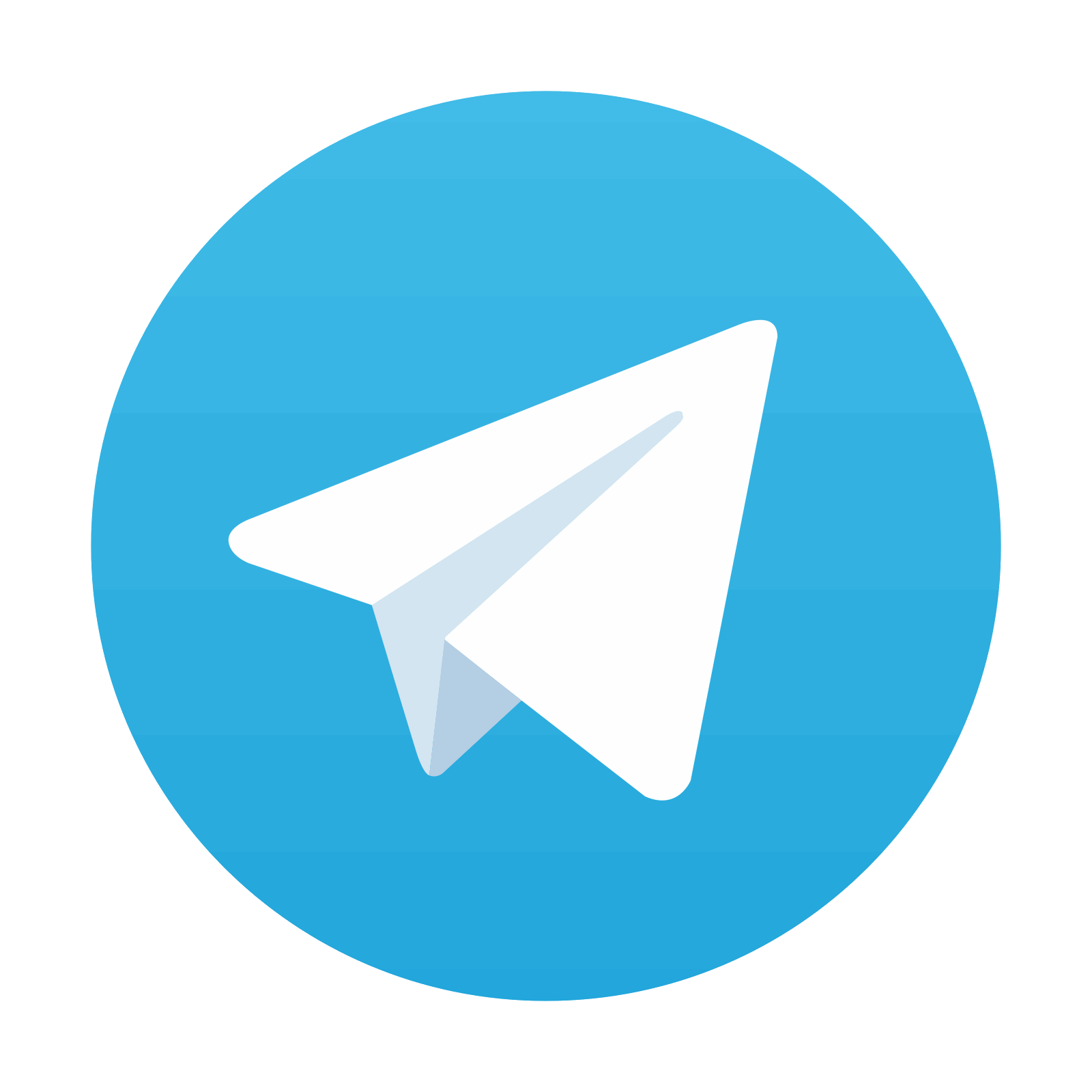
Stay updated, free articles. Join our Telegram channel

Full access? Get Clinical Tree
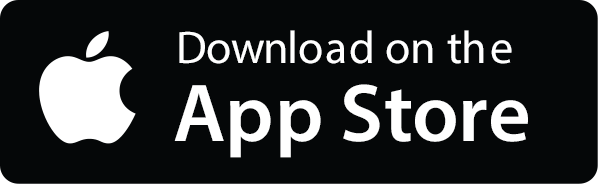
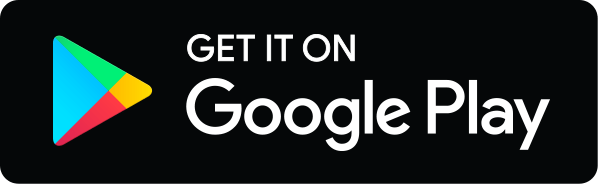