Enhancing the elimination of a xenobiotic from a poisoned patient is a logical step after initial stabilization of the airway, breathing, and circulation; supportive measures; and techniques to inhibit absorption. Table 6–1 lists methods that might be used to enhance elimination. In this chapter, hemodialysis, hemoperfusion, and hemofiltration are considered extracorporeal treatments (ECTRs) because xenobiotic removal occurs in a blood circuit outside the body. Extracorporeal treatments are used infrequently because most poisonings are not amenable to removal by these methods. In addition, because these elimination techniques have associated adverse effects, costs, and complications, the risk-to-benefit analysis suggests they are only indicated in a relatively small proportion of patients and situations.
Occurring Inside the Body | Occurring Outside the Body (Extracorporeal) |
---|---|
Cerebrospinal fluid replacement Forced diuresis Manipulation of urine pH Metal chelators Multiple-dose activated charcoal Peritoneal dialysis Resins (Prussian blue, sodium polystyrene sulfonate, cholestyramine, colestipol) | Exchange transfusion Hemodialysis Hemofiltration and hemodiafiltration Hemoperfusion (charcoal, resin) Liver support devices Plasmapheresis |
Although undoubtedly an underestimate of true use, enhanced elimination techniques were performed relatively infrequently in the cohorts of millions of patients reported by the American Association of Poison Control Centers (AAPCC) National Poison Data System (NPDS) (Chap. 130). Alkalinization of the urine was reportedly used 11,651 times, multiple-dose activated charcoal (MDAC) 1,104 times, hemodialysis 2,663 times, and hemoperfusion 49 times. As in the past, there continue to be many instances of inappropriate use of ECTRs, such as in the treatment of overdoses of cyclic antidepressants (CAs).35
The AAPCC data reveal that there is a continued increase in the reported use of hemodialysis, paralleling a decline in reports of charcoal hemoperfusion (Chap. 130) (Table 6–2). Lithium and ethylene glycol were the most common xenobiotics for which hemodialysis was used between 1985 and 2014 (Fig. 6–1).18 Possible reasons for the decline in use of charcoal and resin hemoperfusion are described in the section on hemoperfusion in this chapter. Peritoneal dialysis (PD), a slower modality that should have little or no role in any poisoning, is no longer reported separately. “Other extracorporeal procedures” in past AAPCC reports include continuous modalities (discussed later in the section Continuous Hemofiltration and Hemodiafiltration), plasmapheresis, and PD.
1986 | 1990 | 2001 | 2004 | 2007 | 2011 | 2015 | |
---|---|---|---|---|---|---|---|
Hemodialysis | 297 | 584 | 1,280 | 1,726 | 2,106 | 2,323 | 2,663 |
Charcoal hemoperfusion | 99 | 111 | 45 | 20 | 16 | 14 | 49 |
Resin | 23 | 37 | — | — | — | — | — |
Peritoneal dialysis | 62 | 27 | — | — | — | — | — |
Other extracorporeal: Such as CVVH and CVVHD | — | — | 26 | 33 | 24 | 26 | 51 |
Very few prospective, randomized, controlled clinical trials have been conducted to determine which groups of patients actually benefit from enhanced elimination of various xenobiotics and which modalities are most efficacious. For most poisonings, it is unlikely that such studies will ever be performed, given the relative scarcity of appropriate cases of sufficient severity and because of the many variables that would hinder controlled comparisons. Thus, limited evidence deemed of high quality exists. We must therefore rely on an understanding of the principles of these methods to identify the individual patients for whom enhanced elimination is indicated. In particular, isolated case reports in which the kinetics are well described, including half-lives with and without enhanced elimination, clearance, and quantification of removal are very useful in establishing the efficacy of a method. Fortunately, in the absence of robust evidence, consensus-based recommendations have now been published and are being developed to guide clinical decisions. The American Academy of Clinical Toxicology (AACT) and the European Association of Poisons Centres and Clinical Toxicologists (EAPCCT) published joint position papers on urine alkalinization50 and MDAC.56 The Extracorporeal Treatments in Poisoning (EXTRIP) work group, a collaboration of experts from diverse specialties (clinical toxicology, nephrology, pharmacology, critical care, emergency medicine) representing more than 30 international societies, has published several recommendations regarding the indications for dialysis and other ECTRs for overdose3,9,17,20,23,24,26,29,39,43,52,58 (see also http://extrip-workgroup.org).
Enhanced elimination is generally indicated for several types of patients, with the preexisting condition that the responsible xenobiotic is removable.22
Patients who fail to respond adequately to comprehensive supportive care. Such patients have evidence of poor prognostic factors such as intractable hypotension, heart failure, seizures, metabolic acidosis, or dysrhythmias. Hemodialysis is much better tolerated than in the past and offers potentially life-saving opportunities for patients with toxicity caused by theophylline, lithium, salicylates, or toxic alcohols, among others.
Patients in whom the normal route of elimination of the xenobiotic is impaired. Such patients have kidney or hepatic dysfunction, either preexisting or caused by the overdose. For example, a patient with impaired kidney function is more likely to develop toxicity from a large lithium ingestion and require hemodialysis as treatment.
Patients in whom the amount of xenobiotic absorbed or its high concentration in serum indicates that serious morbidity or mortality is likely. Such patients do not necessarily appear acutely ill on initial evaluation. Xenobiotics in this group include ethylene glycol, lithium, methanol, paraquat, salicylates, and theophylline.
Patients with concurrent disease or in an age group (very young or old) associated with increased risk of morbidity or mortality from the overdose. Such patients are intolerant of prolonged coma, immobility, and hemodynamic instability. An example is a patient with both severe underlying respiratory disease and chronic salicylate poisoning.
Patients with concomitant electrolyte disorders that could be corrected with hemodialysis. An example is the metabolic acidosis with elevated lactate associated with metformin toxicity discussed in the hemodialysis section of this chapter.
Ideally, these techniques are applied to poisonings for which studies suggest an improvement in outcome in treated patients compared with patients not treated with extracorporeal removal. As previously mentioned, these data are rarely available.21
The need for extracorporeal elimination is less clear for patients who are poisoned with xenobiotics that are known to be removed by an ECTR but that cause limited morbidity if supportive care is provided. Relatively high rates of endogenous clearance would also make extracorporeal elimination redundant. Examples of such xenobiotics include ethanol and some barbiturates; both are subject to substantial rates of hepatic metabolism, and neither would be expected to lead to significant morbidity after the affected patient has had endotracheal intubation and is mechanically ventilated. There may be instances of severe toxicity from these two xenobiotics for which enhanced elimination will reduce the length of intensive care unit (ICU) stays and the associated nosocomial risks; extracorporeal elimination would then be a reasonable option. Dialysis should be avoided if other more effective and less invasive modalities are available. For example, a patient with an acetaminophen (APAP) overdose should be initially treated with N-acetylcysteine instead of hemodialysis unless evidence of mitochondrial injury is apparent.26
The appropriateness of any modality for increasing the elimination of a given xenobiotic depends on various properties of the molecules in question. Effective removal by the ECTR and other methods listed in Table 6–1 is limited by a number of factors, including a large volume of distribution (Vd), molecular and compartmental factors. The Vd relates to the concentration of the xenobiotic in the blood or serum as a proportion of the total body burden. The Vd can be envisioned as the apparent volume in which a known total dose of drug is distributed before metabolism and excretion occur:
The larger the Vd, the less the xenobiotic is present in the blood compartment for elimination. A xenobiotic with a Vd below 1 L/kg is usually considered amenable to extracorporeal elimination. Methanol is an example of a xenobiotic with a small Vd, approximately equal to total body water (0.6 L/kg). A substantial fraction of a dose of methanol can be removed by hemodialysis. In addition to the alcohols, other xenobiotics with a relatively low Vd include phenobarbital, lithium, salicylates, valproic acid, bromide and fluoride ions, and theophylline. In contrast, lipid-soluble xenobiotics have large volumes of distribution (≥1 L/kg of body weight); an insignificant fraction of digoxin with a large Vd (5–12 L/kg of body weight) would be removed by hemodialysis. Other high Vd xenobiotics include many β-adrenergic antagonists (with the possible exception of atenolol), diazepam, organic phosphorus compounds, antipsychotics, quinidine, and the CAs.
Pharmacokinetics also influences the ability to enhance elimination of a xenobiotic. Kinetic parameters after an overdose often differ from those after therapeutic or experimental doses. For instance, carrier- or enzyme-mediated elimination processes may be overwhelmed by higher concentrations of the xenobiotic in question, making extracorporeal removal potentially more useful. Similarly, plasma protein- and tissue-binding sites may all be saturated at higher concentrations, making extracorporeal removal feasible in instances in which it would have no role in less significant overdoses. Examples include salicylates and valproic acid, which are poorly dialyzed at nontoxic concentrations because of high protein binding. However, at higher concentrations, protein-binding sites become saturated and lead to a higher proportion of the drug free in the serum, amenable to removal by hemodialysis at a clinically relevant rate. Estimated endogenous elimination rates of a xenobiotic should be derived by proper toxicokinetic models and not by pharmacokinetic data after therapeutic doses.
When assessing the efficacy of any technique of enhanced elimination, a generally accepted principle is that the intervention is worthwhile only if a large portion of total body drug burden can be eliminated or if total body clearance of the xenobiotic is increased by a factor of at least 2.36 This substantial increase is easier to achieve when the xenobiotic has a low endogenous clearance. Examples of xenobiotics with low endogenous clearances (<4 mL/min/kg) include valproic acid, lithium, paraquat, phenytoin, salicylate, and theophylline. Xenobiotics with high endogenous clearances include many β-adrenergic antagonists, lidocaine, opioids, nicotine, and CAs. Enhancement of elimination is expected to contribute more to overall clearance of the former group than to the latter.
There are many caveats in interpreting the toxicokinetic efficacy of any technique of elimination.37 Several publications only report the blood or serum concentrations of the xenobiotic at the beginning and the end of the procedure; these data are somewhat informative for one-compartment xenobiotics that are limited to the extracellular space, such as theophylline. The difference between the theophylline concentration before the procedure and the concentration at the end of the procedure, divided by the concentration at the beginning, is the fraction of the body burden of the xenobiotic that is eliminated. However, this calculation does not account for endogenous clearance and would not be appropriate to estimate the effect of treatment on the total body burden of a xenobiotic that distributes in a larger Vd or with multicompartment kinetics. Furthermore, the serum concentration of a xenobiotic seldom reflects its concentration in the toxic compartment.
Certain xenobiotics, such as lithium, distribute in part to the intracellular compartment, and equilibrium is established between the intracellular and extracellular compartments. The latter compartment includes the blood from which xenobiotic elimination occurs and the interstitium. An increase in the elimination rate from the extracellular compartment, such as by hemodialysis, alters this equilibrium. The rate of redistribution of the xenobiotic from the intracellular compartment into the now dialyzed intravascular compartment is often slower than the rate of clearance across the dialysis membrane. In that case, the serum concentration becomes relatively low despite a substantial intracellular burden. This low serum concentration reduces the concentration gradient for diffusion from serum to dialysate so that dialysance (the dialysis clearance) is reduced. Thus, although serum concentrations decrease precipitously during the procedure, the total-body burden of the xenobiotic is not affected significantly if it does not move from cells to the dialyzable intravascular compartment. To fully appreciate the limitation of only observing serum concentration, the following example can be illustrated: a 60-kg patient ingests 100 (25-mg) amitriptyline tablets, a CA.14 Because of significant lipophilicity, this class of xenobiotics has a high Vd. Assuming that the drug is fully absorbed, the 2,500 mg of amitriptyline distributes in an apparent volume of 40 L/kg of body weight to achieve a serum concentration of 1,000 ng/mL, a potentially toxic concentration. One might consider dialysis useful if the serum concentration of amitriptyline decreases from 1,000 ng/mL to 400 ng/mL during a 4-hour dialysis. If dialysis is performed with a blood flow rate of 350 mL/min (plasma flow rate of 200 mL/min, assuming a hematocrit of ~43%) and the extraction ratio is 100%, the clearance of drug is 200 mL/min or 200 mcg/min. In 4 hours (240 minutes) of treatment, only 48 mg (48,000 mcg), or less than 2% of the amount ingested, would be removed. The treatment would not affect toxicity despite decreasing serum concentrations.
When assessing overall efficacy, the evidence of enhanced elimination must be considered in the context of the clinical response. In some instances, observed improvement is not predicted by the kinetics of the parent xenobiotic and may not result from the intervention. For example, in severe cases of CA poisoning, unexpected improvement during hemoperfusion could be fortuitous because the toxicity is manifested early and ameliorates rapidly during the initial distribution phase.
Beginning a procedure during the initial distribution phase will likely increase the fraction of the drug burden that can be removed. Later, after the xenobiotic has distributed into fat, moved intracellularly, or has been bound by plasma or tissue proteins, administration of ECTR will have much lower clearance rates and benefits. Examples of xenobiotics that might be better removed soon after ingestion include paraquat and thallium.
The extent of xenobiotic removal by enhanced elimination is not necessarily correlated to clinical improvement. For example, no difference was observed between patients with lithium poisoning for whom hemodialysis was done and those for whom it was recommended by a poison control center but not done.4 Unfortunately, this study was underpowered and limited by confounding by indication. Furthermore, although paraquat exhibits all the chemical characteristics necessary for high extracorporeal removal, the outcome of patients presenting late after paraquat ingestion remains dismal despite active elimination procedures.
Occasionally, there will be unanticipated evidence of improvement despite removal of marginal amounts of the xenobiotic and active metabolites. Some authors have attributed this surprising finding to removal of a critical amount of xenobiotic from a shallow “toxic effect” compartment. This theory has been advanced to explain the response to hemodialysis of patients overdosed with the CAs and the antipsychotic chlorprothixene. Such effects may lead to transient improvements that are not sustained as drug redistributes from one pool to another, leading to early benefit but eventual recurrence of symptoms. Much of the relevant literature fails to provide long-term follow-up to demonstrate prolongation of benefits after the ECTR is completed and xenobiotics have redistributed.
These many limitations in the available medical literature regarding the appropriate indications and utilization of ECTRs in the treatment of poisonings led EXTRIP to promote improvements in case reporting.37 The paper makes suggestions for the data that should be obtained when the ECTR is applied. We recommend that this paper be reviewed when possible before instituting therapy so that the requisite and comprehensive data will be obtained. The result of this consideration will be more thorough case reporting and the ensuing publication of more thorough and useful medical literature.
Although the efficacy of, or need for, removal of many xenobiotics remains controversial, consensus regarding the indications for these procedures has developed. This consensus has led to consistent application of several techniques of enhanced elimination for some toxic exposures that occur relatively more frequently.
Oral administration of multiple doses of activated charcoal increases elimination of some xenobiotics present in the blood. This modality is discussed in more detail in Antidotes in Depth: A1 and will not be discussed here.
Resins are sometimes used in poisoning management. They can reduce the bioavailability of ingested drugs and act as decontaminants, similarly to activated charcoal. In addition, however, they can promote elimination of certain xenobiotics by enhancing their back-diffusion from plasma to gut (gastrointestinal dialysis) and interrupt enterohepatic recirculation.
The most commonly used resins are sodium polystyrene sulfonate (Kayexalate), cholestyramine, and Prussian blue. Sodium polystyrene sulfonate is an ion exchanger that is used regularly for hyperkalemia in patients with chronic kidney disease. Limited data now exist on its potential use in lithium poisoning, although treatment results in hypokalemia.19 Prussian blue is also an ion exchanger used for treatment of poisoning by cesium or thallium (Antidotes in Depth: A31). Cholestyramine, a bile acid sequestrant, binds several xenobiotics, including digoxin, ibuprofen, and mycophenolate mofetil, although its application in poisoning is doubtful (Chap. 62).
Forced diuresis by volume expansion with isotonic sodium–containing solutions, such as 0.9% sodium chloride and Ringer’s lactate (RL) solution with or without the addition of a diuretic, does increase renal clearance of some molecules. This therapy would theoretically be most useful for xenobiotics such as lithium for which the glomerular filtration rate (GFR), representing the volume of plasma filtered across the glomerular basement membrane per minute, is an important determinant of excretion. In people with normal extracellular fluid (ECF) volume, the increase in GFR and increase in xenobiotic renal elimination expected with plasma volume expansion is variable and unpredictable and not likely to be clinically significant. The effect is potentially more important in patients who have contraction of the ECF volume; loss of extracellular volume leads to a reduction of GFR partly as a result of decreased cardiac preload and cardiac output, which, in turn, reduces renal plasma flow. This circumstance is also accompanied by activation of angiotensin II, a small peptide that acts as a pressor and stimulates sodium reabsorption in the proximal tubule. Because small molecules such as lithium are both filtered at the glomerulus and reabsorbed by the proximal tubule, especially when volume depletion has occurred and angiotensin II has been activated, repletion of ECF volume with 0.9% sodium chloride will increase GFR and suppress proximal tubular sodium reabsorption. The result is an increase in excretion of low-molecular-weight xenobiotics such as lithium. After the ECF volume is restored, the continued infusion of 0.9% sodium chloride or RL will not enhance elimination of lithium to any major extent.
The major risk of excessive volume repletion is ECF volume overload, manifested by pulmonary and cerebral edema. This complication is particularly likely in patients with baseline chronic kidney disease such as is associated with long-standing lithium use. Other patients with acute kidney injury (AKI) not mediated by ECF volume depletion are also at risk. Knowing the result of past serum creatinine concentrations permits distinction between acute from chronic kidney disease in such cases. Administration of diuretics such as furosemide along with saline diminishes the risk of ECF volume overload but complicates the therapy, confuses the assessment of ECF volume, and increases the risk of metabolic alkalosis and hypokalemia. The unproven efficacy of enhanced diuresis in the management of any overdose has led most experts to abandon its use. On the other hand, the repletion of ECF volume when volume contraction is present, as determined by the history and physical examination, is, of course, appropriate. Saline diuresis may be used to treat toxin-induced hypercalcemia, which can result from excessive ingestion of calcium supplements or vitamin D ingestion.
Many xenobiotics are weak acids or bases that are ionized in aqueous solution to an extent that depends on the pKa of the xenobiotic and the pH of the solution. Knowing these variables, the Henderson-Hasselbalch equation (Chaps. 9 and 10) can be used to determine the relative proportions of the acids, bases, and buffer pairs. Whereas cell membranes are relatively impermeable to ionized, or polar molecules (eg, an unprotonated salicylate anion), nonionized, nonpolar forms (eg, the protonated, noncharged salicylic acid) cross more easily. As xenobiotics pass through the kidney, they may be filtered, secreted, and reabsorbed. If the urinary pH is manipulated to favor the formation of the ionized form in the tubular lumen, the xenobiotic is trapped in the tubular fluid and is not passively reabsorbed into the bloodstream. This is referred to as ion trapping. Hence, the rate and extent of its elimination can be increased. To make manipulation of urinary pH worthwhile, the renal excretion of the xenobiotic must be a major route of elimination. The 2004 position paper of the AACT and EAPCCT emphasizes that, as discussed earlier for extracorporeal therapies, enhanced removal does not necessarily translate into a clinical benefit with improved outcomes.50
Alkalinization of the urine to enhance elimination of weak acids has a role for salicylates, phenobarbital, chlorpropamide, formate, diflunisal, fluoride, methotrexate, and the herbicide 2,4-dichlorophenoxyacetic acid (2,4-D). These weak acids are ionized at alkaline urine pH, and tubular reabsorption is thereby greatly reduced. Alkalinization is achieved by the intravenous administration of sodium bicarbonate (1–2 mEq/kg rapid initial infusion with additional dosing) to increase urinary pH to 7 to 8. This degree of alkalinization will be difficult, if not impossible, if metabolic acidosis and acidemia are present, as often is the case in patients with salicylate poisoning. In this situation, bicarbonate (administered as the sodium salt, sodium bicarbonate) is consumed by titration of plasma protons before it can appear in the urine. On the other hand, salicylate poisoning often causes respiratory alkalosis as well. In that case, when the PCO2 is low, raising serum bicarbonate, equivalent to the induction of metabolic alkalosis, may lead to profound, life-threatening alkalemia. Finally, as for the case of 0.9% sodium chloride or RL, there is a risk of ECF volume overload with sodium bicarbonate administration. Modest reductions in GFR also reduce the likelihood of successful urinary alkalinization and increase the risk of pulmonary edema. Hypernatremia may also occur, especially if sodium bicarbonate is given as a hypertonic solution. Bicarbonaturia is also associated with urinary potassium losses, so the patient’s serum potassium concentration should be monitored frequently and the appropriate dose of potassium administered as long as GFR is not impaired. A further complication of alkalemia is a decrease of ionized calcium, which becomes bound by albumin as protons are titrated off serum proteins; in this event, tetany, prolonged QT interval, and cardiac dysrhythmias are risks. If these complications can be identified and dealt with judiciously and safely, the renal clearance of salicylate increases fourfold as urine pH increases from 6.5 to 7.5 with alkalinization.49 Increasing urine pH by decreasing proximal tubular bicarbonate reabsorption via administration of carbonic anhydrase inhibitors such as acetazolamide is not recommended. Although an alkaline urine pH will result and elimination of a xenobiotic enhanced, this is achieved at the expense of inducing a metabolic acidosis. In the case of salicylates, acidemia increases distribution into the central nervous system. The role of urinary alkalinization in the management of patients with salicylate poisoning is discussed further in Chap. 37.
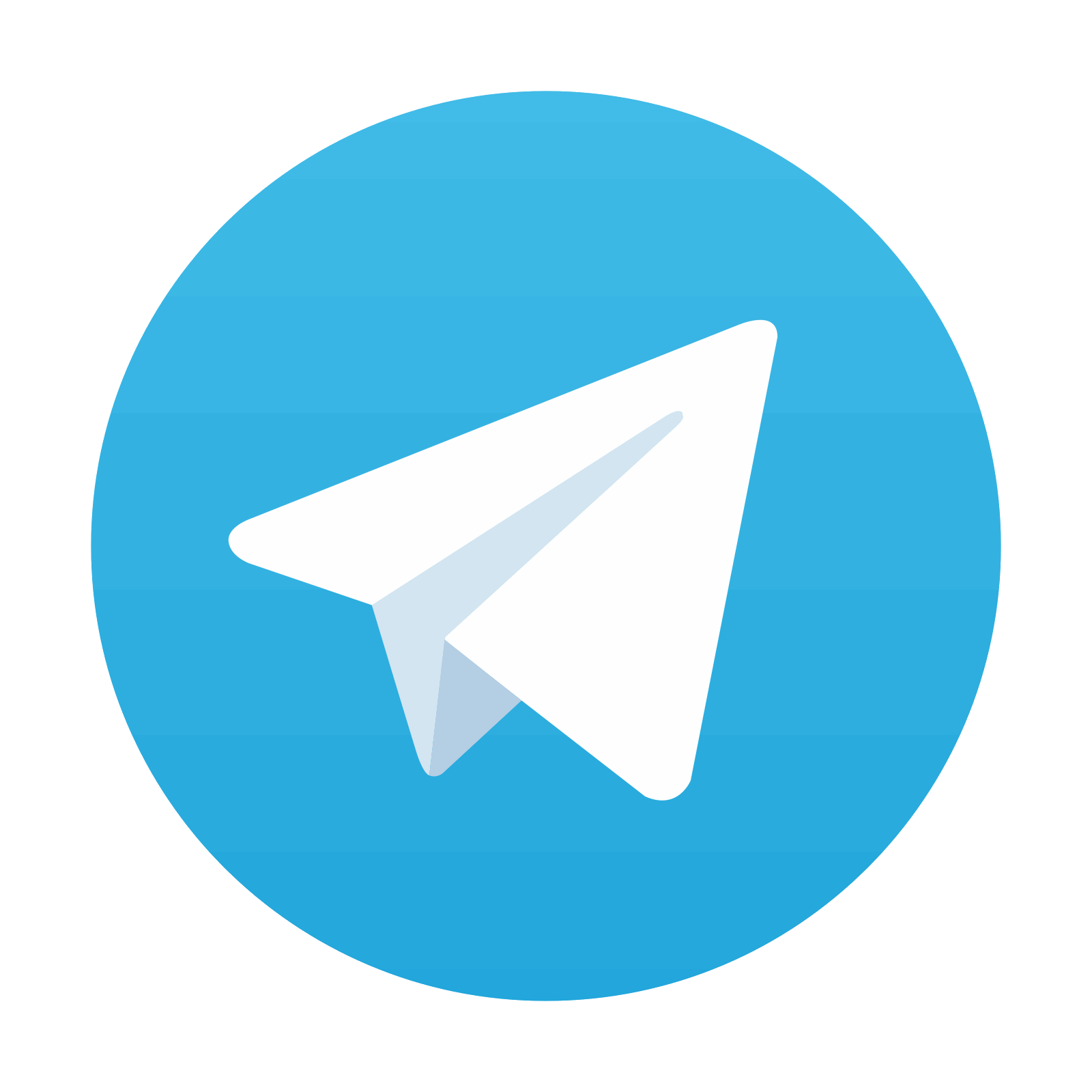
Stay updated, free articles. Join our Telegram channel

Full access? Get Clinical Tree
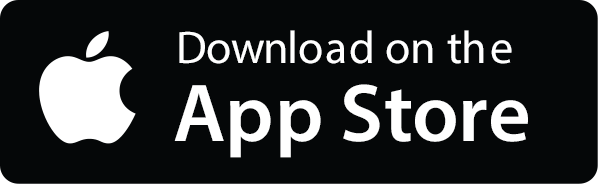
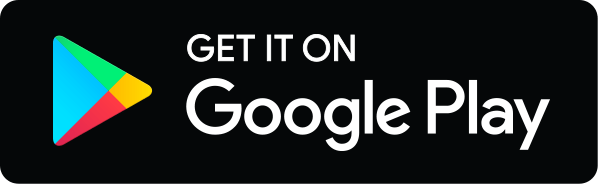