The indications for diagnostic thoracentesis are not different in the ICU patient, and receiving mechanical ventilation is not a contraindication. Establishing the diagnosis quickly in critically ill patients may be more important than in the noncritically ill. The reported incidence of pneumothorax in nonventilated patients ranges from 4% to 30% (22–25). Various risk factors for developing a pneumothorax after thoracentesis have been reported, although operator inexperience, baseline lung disease, and use of positive pressure mechanical ventilation appear to be the most established risk factors. Several earlier studies have demonstrated that the incidence of pneumothorax after blind thoracentesis in mechanically ventilated patients, 5% to 10%, is similar to that of nonventilated patients, and it is thus safe to perform blind thoracentesis in mechanically ventilated patients (26,27). If the patient on mechanical ventilation does develop a pneumothorax, however, a significant risk of progression to a life-threatening tension pneumothorax exists. As such, some authors have advocated the routine use of ultrasound guidance for all thoracentesis procedures in mechanically ventilated patients, given the observed pneumothorax rates of 0% to 3% with ultrasound guidance in nonventilated patients (25,28), as well as in patients receiving mechanical ventilation (9–11). Strong consideration should be given to using US guidance in patients with small or moderate effusions. US or CT guidance should be used to sample loculated pleural fluid collections. There are no absolute contraindications to diagnostic thoracentesis; the major relative contraindications are a bleeding diathesis or anticoagulation. In one study of 207 patients requiring thoracentesis with mild to moderate coagulopathy, defined as a prothrombin time (PT) or partial thromboplastin time (PTT) up to twice normal, or a platelet count from 50,000 to 100,000 cells/μL, no increase in bleeding complications was noted (29). Thoracentesis should not be performed through an area of active skin infection. Analysis of pleural fluid in the ICU patient is similar to that in other settings and is beyond the scope of this chapter.
Therapeutic Thoracentesis and Physiologic Effects
The primary indication for therapeutic thoracentesis or chest tube drainage of a pleural effusion is relief of dyspnea, although pulmonary mechanics and oxygenation may be improved in some patients (30). Contraindications to and complications of therapeutic thoracentesis are similar to those of diagnostic thoracentesis, with the additional complications of hypoxemia, reexpansion pulmonary edema, and hypovolemia. An increased risk of pneumothorax has been noted with therapeutic thoracentesis in some studies (24,25,31) although not others (32,33). We would recommend the use of a catheter-over-needle system in performing therapeutic thoracentesis to reduce the risk of developing a pneumothorax. In patients with pleural effusion and ipsilateral shift suggesting endobronchial obstruction, chronic atelectasis, or a trapped lung, the risk of reexpansion pulmonary edema may be increased, and the patient may be less likely to experience a beneficial effect. In addition, patients with initial negative pleural pressures and those with more precipitous falls in pleural pressures with fluid removal also likely have trapped lung or endobronchial obstruction and are less likely to benefit from therapeutic thoracentesis (34).
Pleural effusions compress the lung, causing atelectasis, ventilation/perfusion mismatch, and shunt physiology with resultant hypoxemia (35). Pleural fluid tends to enlarge the volume of the hemithorax more than it compresses lung volume. Studies in humans have shown that total lung capacity following thoracentesis increases by only approximately one-third of the thoracentesis fluid volume, and forced vital capacity increases by approximately half of the increase in total lung capacity. Studies evaluating gas exchange in nonventilated patients have been mixed. One found a decrease in PaO2 (36), while another found no change in PaO2 (37), and a third showed a mild increase in PaO2 (38). More recent studies have also shown variable results, with one study reporting a small increase in PaO2 and decrease in alveolar-arterial O2 gradient (39), although another noted no change in PaO2, alveolar-arterial O2 gradient or shunt, while the amount of blood flow to low ventilation/perfusion units increased slightly (35).
Despite these mixed results, some patients requiring mechanical ventilation may benefit from pleural fluid drainage. Talmor et al. (30) reported that 19 patients with acute respiratory failure and pleural effusions who had a poor response to positive end-expiratory pressure (PEEP), defined as the inability to wean FiO2 to 0.5 with PEEP up to 20 cm H2O, benefited from chest tube drainage of the pleural effusions. The PaO2 increased from 125 to 199 mmHg, and the PaO2/FiO2 ratio increased from 151 to 254. Fourteen patients had a unilateral effusion, and five patients had bilateral effusions necessitating bilateral chest tube placement. More recently, Doelken et al. (40) studied the effects of thoracentesis on respiratory mechanics and gas exchange in eight mechanically ventilated patients. Following removal of 800 to 1,950 mL (mean 1,495 mL), no significant change in PaO2 or dead space ventilation was observed. No significant changes were noted for peak and plateau pressures, dynamic and effective static compliance, respiratory system resistance, and intrinsic PEEP. Mean work performed by the ventilator did significantly decrease, however. A recent study of 20 patients showed that drainage of more than 500 mL of pleural fluid in mechanically ventilated patients improved respiratory mechanics with a decrease in plateau pressure and a large increase in end-expiratory transpulmonary pressure. Improvement in the PaO2/FiO2 ratio from baseline to 24 hours post drainage was positively correlated with the increase in end-expiratory lung volume but not with the amount of fluid drained. There was no significant effect of large volume thoracentesis on patient hemodynamics (41).
Further studies are required to confirm these results. In patients who are difficult to wean from mechanical ventilation, we would consider a trial of therapeutic thoracentesis.
COMMON CAUSES OF PLEURAL EFFUSIONS IN THE ICU
Abdominal Surgery
Approximately one-half of patients undergoing abdominal surgery will develop small unilateral or bilateral pleural effusions 24 to 48 hours following surgery (42,43). The incidence of pleural effusions is higher in procedures involving the upper abdomen, in patients having ascitic fluid at time of surgery, and in patients who have postoperative atelectasis (34). Larger left-sided effusions are common following splenectomy. The effusion after abdominal surgery is usually exudative∗ with a normal glucose level, pH more than 7.40, and less than 10,000 nucleated cells/μL (42). Small effusions generally do not require diagnostic thoracentesis and resolve spontaneously without becoming clinically significant. Thoracentesis is indicated to exclude empyema if the effusion is relatively large or loculated or if the possibility of a subdiaphragmatic abscess related to the surgery exists.
Acute Respiratory Distress Syndrome
The presence of pleural effusions in acute respiratory distress syndrome (ARDS) has not been well appreciated or studied. In a retrospective study of 25 patients with ARDS, 36% were found to have pleural effusions (44). All patients had extensive alveolar infiltrates in addition to pleural effusions. Pleural effusions have been observed in animal models of ARDS using α-naphthylthiourea, oleic acid, and ethchlorvynol (45,46). In the oleic acid model, 35% of the excess lung water collected in the pleural spaces (45). Effusions are likely underdiagnosed in ARDS because the patient has bilateral alveolar infiltrates and the radiograph is taken in the supine position. In experimental models of ARDS, the effusions are serous to serosanguineous with a predominance of polymorphonuclear leukocytes (PMNs) (46). In a post hoc analysis of a study looking at the effect of large volume thoracentesis in mechanically ventilated patients, no significant improvement in PaO2/FiO2 ratio was observed in patients with ARDS (41). These effusions resolve as the ARDS resolves and require no specific therapy.
Atelectasis
Atelectasis is a common cause of small pleural effusions in the ICU due to patients being immobile (4). Atelectasis and small effusions are commonly observed following cardiothoracic or abdominal surgery; other potential causes include endobronchial obstruction from tumor, foreign body, or mucus plugging as well as extrinsic airway compression from malignancy. With lung collapse, local areas of increased negative pressure are created by the separation of the lung and chest wall. The decrease in pleural pressure favors the movement of fluid into the pleural space, presumably from the surface of the parietal pleura (19).
Pleural effusions from atelectasis are serous transudates with a few mononuclear cells, a glucose concentration equal to serum, and a pH of 7.45 to 7.55. The pleural effusions dissipate over several days when the atelectasis resolves.
Chylothorax
A chylothorax is defined as the accumulation of chyle in the pleural space. The predominant mechanisms of chylothorax formation include disruption of the thoracic duct, extravasation from pleural lymphatics, and transdiaphragmatic efflux from chylous ascites (47). The most common cause of chylothorax is lymphoma, accounting for 37% of chylothoraces in a series of 191 patients (48); the second most frequent cause is surgical trauma, which represented 25% of cases in the same series of 191 patients (48). The incidence of chylothorax following thoracic surgery has been reported to be 0.36% to 0.42% (49,50) and 1.9% following lower neck surgery (46). A higher proportion of chylothoraces are noted following esophagectomy. Virtually all intrathoracic surgical procedures, including lobectomy, pneumonectomy, and coronary artery bypass grafting, have been reported to cause chylothorax (48). Nonsurgical trauma, including blunt and penetrating injuries to the neck, thorax, and upper abdomen as well as obstruction of the superior vena cava or thrombosis of the left subclavian vein from indwelling central venous catheters (CVCs), may produce chylothoraces in ICU patients (51).
The patient may be asymptomatic if the effusion is small and unilateral, or may be dyspneic with a large unilateral effusion or bilateral effusions. The pleural fluid is usually milky but can be serous, serosanguineous, or bloody. The fluid may not have a milky appearance if the patient is malnourished or not eating (52). The pleural fluid typically has less than 7,000 nucleated cells/μL, which are over 80% lymphocytes. The pH is alkaline (7.40–7.80), and the triglyceride levels exceed plasma levels (19). A pleural fluid triglyceride concentration greater than 110 mg/dL makes the diagnosis of chylothorax highly likely, whereas a concentration below 50 mg/dL makes the diagnosis highly unlikely. With triglyceride concentrations of 50 to 110 mg/dL, lipoprotein electrophoresis is indicated to demonstrate the presence of chylomicrons, which confirms the diagnosis of chylothorax (52).
Up to 2 to 3 L of chyle may drain daily, causing loss of fluid, electrolytes, protein, fat, fat-soluble vitamins, and lymphocytes. Severe nutritional depletion and immunodeficiency may result if these losses are not addressed. In addition to chest tube drainage, initial conservative management consists of intravenous hydration and a nonfat, high-protein, high-calorie diet with medium-chain triglycerides, which are absorbed directly into the portal system, or discontinuing all oral feeding and initiating total parenteral nutrition. If the chylothorax fails to resolve with conservative measures after 7 to 14 days, then surgery with thoracic duct ligation may be considered (53), although pleuroperitoneal shunting has also been used.
Congestive Heart Failure
Congestive heart failure (CHF) is the most common cause of all transudative pleural effusions and in one study was the most common cause of pleural effusions in a medical ICU (4). Pleural effusions due to CHF are associated with increases in pulmonary venous pressure. In a study of 37 patients admitted for CHF, the mean pulmonary capillary wedge pressure (PCWP) was higher in patients with pleural effusions than in those without—24.1 versus 17.2 mmHg, respectively (54). Isolated increases in right heart pressures were not associated with pleural effusions. Patients with chronic obstructive pulmonary disease (COPD) and cor pulmonale, in the absence of left ventricular dysfunction, thus, rarely have pleural effusions, and other causes for pleural effusions should be sought in these patients.
Most patients with pleural effusion secondary to CHF have the usual signs and symptoms. The chest radiograph classically demonstrates cardiomegaly and bilateral small to moderate pleural effusions of similar size, with right-sided effusions often being slightly greater than the left. Radiographic evidence of pulmonary edema is usually present, with the severity of pulmonary edema correlating with the presence of effusions. In patients who have been hospitalized, records will usually show intake greater than output for several days, weight gain, an increasing alveolar-arterial O2 gradient, and decreasing compliance in those patients requiring mechanical ventilation. Some patients without a history of CHF may not be suspected of having CHF until intravenous hydration produces pleural effusions and subsequent echocardiograms demonstrate left ventricular dysfunction (4).
Pleural effusions from CHF are transudates and have less than 1,000 nucleated cells/μL, which are mainly mesothelial cells and lymphocytes. Acute diuresis may increase the protein concentration of the pleural fluid and thus change the classification of the fluid from transudative to exudative in 8% to 38% of patients (55). In the afebrile patient with clinical CHF and cardiomegaly with bilateral effusions of relatively equal size on chest radiograph, the diagnosis is reasonably secure and observation is appropriate. Thoracentesis should be considered in patients who are febrile, have pleuritic chest pain, or are noted on chest radiograph to have effusions of disparate size, unilateral effusions, a larger effusion on the left than the right, or absence of cardiomegaly.
Treatment consists of decreasing preload and improving cardiac output with diuretics, inotropes, and afterload-reducing agents. With appropriate management, the pleural effusions will resolve over days to weeks.
Coronary Artery Bypass Surgery
A small left pleural effusion is virtually always present following coronary artery bypass surgery (CABG). The effusion is associated with left lower lobe atelectasis and elevation of the left hemidiaphragm on chest radiograph. Approximately 10% of patients will have a larger effusion occupying more than 25% of the hemithorax. These large effusions can be separated into early effusions occurring within the first 30 days of surgery that are bloody exudates with a high percentage of eosinophils, and late effusions occurring more than 30 days after surgery that are clear yellow lymphocytic exudates (56). Factors associated with higher incidence of post-CABG effusions and large effusions are internal mammary artery grafting, on pump surgery, and topical cardiac hypothermia with cold saline.
Rarely, a loculated hemothorax may develop with a trapped lung, resulting in clinically significant restriction (57). If a large effusion that qualifies as a hemothorax is present, the fluid should be drained by tube thoracostomy. It is unclear if a large hemorrhagic effusion with a pleural fluid/blood hematocrit less than 50% needs to be drained to avoid later necessity for decortication. If the effusion is small and the patient is asymptomatic, no treatment is necessary. Treatment with anti-inflammatory agents and possibly chemical pleurodesis may be required for patients with recurrent nonbloody effusions (56).
Esophageal Rupture
Spontaneous esophageal rupture—Boerhaave syndrome—is a potentially life-threatening event and requires immediate diagnosis and therapy. Esophageal rupture or perforation may rarely occur with blunt thoracic trauma or as a complication of endoscopy and nasogastric/orogastric tube placement. The history in spontaneous esophageal rupture is usually severe retching or vomiting; however, activities that generate a Valsalva maneuver can cause esophageal rupture, and in some patients the perforation may be silent (58). The findings on chest radiograph may vary depending on the time between perforation and obtaining of the chest radiograph, the site of perforation, and integrity of the mediastinal pleura. Mediastinal emphysema is present in less than half of patients, and may take 1 to 2 hours to be observed, whereas mediastinal widening may take several hours. Pneumothorax, indicating rupture of the mediastinal pleura, is present in 75% of patients; 70% of pneumothoraces are on the left, 20% are on the right, and 10% are bilateral (59). Pleural effusion, with or without associated pneumothorax, occurs in 75% of patients. A presumptive diagnosis should be confirmed radiographically with an esophagram as soon as possible. Because rapid passage of the contrast in the upright patient that may not demonstrate a small perforation, the study should be done with the patient in the appropriate lateral decubitus position.
Pleural fluid findings depend on the degree of perforation and the timing of thoracentesis. Early thoracentesis without mediastinal perforation shows a sterile serous exudate with a predominance of PMNs and a pH greater than 7.30. Amylase of salivary origin appears in the fluid in high concentration following disruption of the mediastinal pleura. With the seeding of the pleural space by anaerobic bacteria, the pH falls rapidly and progressively to approach 6.00. The presence of food particles and squamous epithelial cells in the pleural fluid also suggests esophageal rupture (60). Management is usually operative intervention in conjunction with pleural space drainage and antibiotics. Nonoperative therapy with antibiotics and chest tube drainage alone may be considered in a nontoxic patient with small perforations due to instrumentation.
Esophageal Sclerotherapy
Pleural effusions are found in approximately 50% of patients 48 to 72 hours following esophageal sclerotherapy (61). Effusions may be unilateral or bilateral, with no predilection for side. The effusions tend to be small serous exudates with variable nucleated (38,000–90,000 cells/μL) and red cell counts (126,000–160,000 cells/μL) and glucose concentrations similar to serum. The mechanism for development of these effusions is likely extravasation of the sclerosant beyond the esophageal mucosa, resulting in mediastinal and mediastinal pleural inflammation. An effusion that is not associated with fever, chest pain, or signs of perforation is not important clinically, and will usually resolve over several days to weeks without specific therapy. A diagnostic thoracentesis should be performed and an esophagram considered in patients with symptomatic effusions for 24 to 48 hours to exclude empyema and esophageal perforation.
Hemothorax
Hemothorax needs to be differentiated from a hemorrhagic pleural effusion, as the latter can be the result of only a few drops of blood in serous pleural fluid. The arbitrary definition of a hemothorax is a pleural fluid to blood hematocrit ratio greater than 50%. Hemothorax can be divided into three categories based on etiology: spontaneous, iatrogenic, and traumatic hemothorax; most hemothoraces result from blunt or penetrating thoracic trauma (62). Other etiologies include invasive procedures, pulmonary infarction, malignancy, and ruptured aortic aneurysms. Anticoagulation therapy or coagulopathy may rarely cause a spontaneous hemothorax. Hemothorax should be suspected in any patient with blunt or penetrating chest trauma with a pleural effusion on chest radiograph. Chest tube thoracostomy with a 28-Fr chest tube, or larger, should be performed in these patients and pleural fluid hematocrit measured. In patients with suspected iatrogenic or spontaneous hemothorax, thoracentesis should be performed first, and if positive, a chest tube should be inserted. Chest tube drainage allows the monitoring of the rate of bleeding, may potentially tamponade the bleeding, and will evacuate the pleural space, thus decreasing the risk of developing empyema or a subsequent fibrothorax (62,63). Prophylactic use of antibiotics (usually a first-generation cephalosporin) for at least 24 hours after the start of chest tube drainage for traumatic hemothorax, reduces the incidence of pneumonia and empyema (64). Whether antibiotic prophylaxis is useful for spontaneous hemothorax has not been investigated accurately.
Intrapleural fibrinolytic therapy can be applied in an attempt to evacuate residual blood clots and breakdown adhesions when initial chest tube drainage is inadequate. The dose, time to initiate, frequency, and duration of fibrinolytic use is unclear from existing literature. Generally, it is advised to evacuate the clotted hemothorax within 7 to 10 days. We recommend 5 to 10 mg of tissue plasminogen activator (tPA) in 50 mL of normal saline given intrapleurally once daily for 3 days starting on day 4 or later of the development of the hemothorax and evaluating the response at the end of 3 days with a chest CT. Indications for surgical exploration vary between clinicians, but general guidelines are hemodynamic instability despite adequate resuscitation, initial drainage greater than 1,500 mL, continued bleeding of more than 200 mL/hr for 3 consecutive hours, continued bleeding of more than 1,500 mL/day, and radiographic evidence of significant retained clot despite adequate noninvasive management as mentioned above (greater than one-third of the pleural space).
Hepatic Hydrothorax
Pleural effusions are present in approximately 6% of patients with cirrhosis and clinically apparent ascites (65,66). The effusions result from movement of ascitic fluid through congenital or acquired diaphragmatic defects. Rarely, a hepatic hydrothorax may be found in a patient without clinical ascites but with ascites demonstrated only by US, implying the presence of a large diaphragmatic defect. With a small pleural effusion, the patient may be asymptomatic, whereas with large to massive effusions, the patient may have varying degrees of dyspnea. The chest radiograph usually demonstrates a normal cardiac silhouette and a right-sided pleural effusion in 70% of patients, which can vary from small to massive. Effusions are less commonly isolated to the left pleural space (15%) or are bilateral (15%). The pleural fluid is a serous transudate with a low nucleated cell count and a predominance of mononuclear cells, pH greater than 7.40, a glucose level similar to serum, and an amylase less than serum amylase (19). The diagnosis is substantiated by demonstrating that the pleural fluid and ascitic fluid have similar chemistries. If the diagnosis is still in question, injection of a radionuclide into the ascitic fluid with subsequent detection on chest imaging supports the diagnosis (67).
Treatment of hepatic hydrothorax is directed at resolution of the ascites with sodium restriction, diuretics, and paracentesis. It is not uncommon for the effusion to persist until all of the ascitic fluid is mobilized. If the patient is acutely dyspneic or hypoxemic, therapeutic thoracentesis may be done as a temporizing measure. Chest tube drainage should be avoided, as it can cause infection of the fluid, and the prolonged drainage can lead to volume depletion, protein and lymphocyte depletion, and may precipitate renal failure. Chemical pleurodesis is usually unsuccessful due to rapid movement of ascitic fluid into the pleural space. Transjugular intrahepatic portal systemic shunt (TIPS) has been used to treat symptomatic hepatic hydrothorax refractory to medical management (65,66), as has video-assisted thoracoscopic surgery to patch the diaphragmatic defect followed by pleural abrasion or talc poudrage (68).
Hepatic hydrothorax may occasionally be complicated by spontaneous bacterial empyema (SBE), also known as spontaneous bacterial pleuritis, in 13% to 16% of the cases (69). The formation of SBE is a result of either bacterial translocation from infected ascitic fluid or bacteremia and seeding of a hepatic hydrothorax. However, in approximately 40% of cases, SBE can occur in the absence of spontaneous bacterial peritonitis (SBP) and even in the absence of ascites (70). The diagnostic criteria for SBE are similar to those for SBP, requiring a serum/pleural fluid albumin gradient greater than 1.1, a PMN count more than 250 cells/μL with a positive culture, or a PMN count greater than 500 cells/μL with negative cultures and exclusion of contiguous infections. The treatment for SBE is a third-generation cephalosporin given IV for 7 to 10 days. Given the significant mortality rate and its proven benefit in SBP, IV albumin 1.5 mg/kg on day 1 and 1 mg/kg on day 3 post diagnosis is recommended, although albumin use has not been specifically studied in SBE. Chest tube is generally not recommended in SBE unless frank pus is present, because it can lead to life-threatening fluid depletion, protein loss, and electrolyte imbalance (71).
Hypoalbuminemia
Many patients admitted to the medical ICU have chronic illnesses and associated hypoalbuminemia. Pleural effusions may be observed when the serum albumin is less than 1.8 g/dL. In one study evaluating the association of pleural effusions with hypoalbuminemia, 3 of 21 (14%) patients with serum albumin less than 2.0 g/dL had pleural effusions (72). Since the normal pleural space has an effective lymphatic drainage system, pleural fluid tends to be the last site of collection of extravascular fluid in patients with low oncotic pressure. It is, therefore, unusual to find a pleural effusion solely due to hypoalbuminemia in the absence of anasarca. The chest radiograph usually shows small to moderate bilateral effusions with a normal heart size. The pleural fluid is a serous transudate with pH ranging from 7.45 to 7.55, and the glucose level is similar to serum. Since hypoalbuminemia is an extremely rare cause of pleural effusion, recognition in these patients should prompt careful clinical evaluations to identify other potential causes for the effusion. The effusions resolve when the hypoalbuminemia is corrected.
Iatrogenic
Insertion of a CVC or extravascular migration of a CVC into the pleural space can cause a pneumothorax, hemothorax, chylothorax, or transudative pleural effusion (73,74). The incidence of this complication appears to be approximately 0.4% to 1.0% of catheter placements, but it may be higher considering that some cases remain unrecognized. It is more common with insertion into the left subclavian and internal jugular veins due to the horizontal orientation of the left brachiocephalic vein in relation to the superior vena cava (58). Catheterization via the internal jugular vein may result in fewer malpositions than catheterization via the subclavian vein (75). The postprocedure chest radiograph should always be assessed for proper catheter placement, with catheter positioning parallel to the long axis of the superior vena cava and tip positioning at the right tracheobronchial angle indicating proper placement (76).
Symptoms include chest pain and dyspnea in the conscious patient. Depending on the volume and rate of infusion of fluid into the mediastinum, tachypnea, respiratory distress, and cardiac tamponade may occur. The chest radiograph demonstrates the catheter tip in an abnormal position, a widened mediastinum, and unilateral or bilateral effusions. The effusion can have characteristics similar to the infusate (milky if lipid is being given), and may be hemorrhagic and neutrophil predominant due to trauma and inflammation. If a glucose-containing solution is being infused, the pleural fluid to serum glucose ratio is greater than 1.0 (74). The CVC should be removed immediately. Observation is sufficient if the effusion is small; if the effusion is large or causes respiratory distress, thoracentesis or tube thoracostomy should be performed. If a hemothorax is discovered, a chest tube should be placed.
Pancreatitis
Pleural effusions are commonly associated with pancreatitis due to the close proximity of the pancreas to the diaphragm. Pleural effusions have been noted in 3% to 20% of patients with pancreatitis (77). They are usually small to moderate left-sided effusions (60%), although effusions may be isolated to the right side (30%) or occur bilaterally (10%) (78). Pleural effusions related to acute pancreatitis have been shown to be an independent negative prognostic factor as well as a predictor of subsequent pseudocyst development (77–79). The diagnosis is confirmed by an elevated pleural fluid amylase concentration that is greater than serum, although a normal pleural fluid amylase may be found early in the course of acute pancreatitis. The pleural fluid is an exudate with 10,000 to 50,000 nucleated cells/μL, predominantly PMNs. The pleural fluid pH is usually 7.30 to 7.35, and the glucose level is similar to serum (19). No specific treatment is necessary for pleural effusions associated with acute pancreatitis. The effusion resolves as the pancreatic inflammation subsides; if the pleural effusion does not resolve in 2 to 3 weeks, pancreatic abscess or pseudocyst should be suspected.
As opposed to acute pancreatitis, pleural effusion due to a pancreaticopleural fistula is extremely unusual, with a frequency of 0.4% to 7% in chronic pancreatitis patients and 6% to 14% in patients with a pseudocyst. These effusions are large, recurrent, and often left sided (76%) (80). The underlying pancreatic disease is often asymptomatic, and therefore the diagnosis can be missed. Pleural fluid analysis reveals an extremely elevated pleural fluid amylase level (normal <150 IU/L), lipase, and high albumin content (>3 g/dL) (81). Serum amylase, is usually only mildly elevated. CT of the abdomen may demonstrate the fistulous tract and/or a pseudocyst. Magnetic resonance cholangiopancreaticography (MRCP) is reported to be particularly useful in demonstrating the presence of a pancreatic fistula. Endoscopic retrograde cholangiopancreaticography (ERCP) leads to diagnosis in 80% of cases and demonstrates the fistulous tract in 59% to 74% of the cases. Early endoscopic intervention with pancreatic duct stent placement is recommended given its high success rate in fistula closure. Medical therapies are useful adjuncts to endoscopic therapy but, alone, rarely result in pancreaticopleural fistula closure. Surgical interventions should only be considered after failure of endoscopic and medical therapies (82,83).
Parapneumonic Effusions and Empyema
Pleural effusions are a common finding in patients with pneumonia. More than 40% of patients with bacterial pneumonia, and 60% of patients with pneumococcal pneumonia, develop parapneumonic effusions. While treatment with antibiotics leads to resolution in most patients, some patients develop a more fibrinous reaction, with the presence of frank pus in the most severe cases. An empyema is defined as the presence of pus in the pleural space, although many clinicians extend the definition to include pleural fluid that has a positive Gram stain for bacteria or a positive bacterial culture. Complicated parapneumonic effusions are defined as pleural effusions in the setting of pneumonia that have either a pH below 7.2, glucose less than 60 mg/dL, lactate dehydrogenase (LDH) more than 1,000 IU/L, or septations or loculations, whereas uncomplicated parapneumonic effusions are the ones which do not meet the criteria for complicated parapneumonic effusion or empyema (84–87).
The usual presentation is similar to the non-ICU patient with fever, dyspnea, chest pain, purulent sputum, leukocytosis, and a new alveolar infiltrate on chest radiograph. In the elderly, debilitated, or immunosuppressed patient, however, many of these findings may be absent. Although pleural space infections most commonly occur in association with pneumonia, it should also be recognized that pleural space infections may result from thoracic surgery, chest tube placement, penetrating chest trauma, esophageal perforation, mediastinitis, subdiaphragmatic abscesses, SBP, and bacteremic seeding of a pre-existing effusion (84).
Once the diagnosis of parapneumonic effusion or empyema has been made, treatment options should be considered, which center around antibiotic therapy and pleural drainage. Pleural drainage can include therapeutic thoracentesis, image-guided catheter placement, tube thoracostomy, and surgical drainage procedures. There are multiple consensus guidelines to aid clinician with decisions regarding pleural drainage (88). In essence, free-flowing effusions less than 10 cm on lateral decubitus can be managed with antibiotics and close observation. For small to moderate effusions the fluid should be sampled, but further drainage procedures are not necessary in absence of empyema or evidence of complicated parapneumonic effusion, as diagnostic thoracentesis can completely drain the pleural space, and thus also serve a therapeutic role. In patients with complicated parapneumonic effusion or empyema, formal pleural drainage with a chest tube is indicated. Considerable controversy exists about what size chest tube is appropriate in which clinical settings. Typically, chest tubes are divided into small-bore (≤14 Fr) versus large-bore (≥14 Fr) tubes with smaller tubes generally placed by percutaneous Seldinger approaches and larger-bore tubes by open incision. Prospective evaluation of the outcomes relative to quartiles of chest tube size (<10 Fr, 10–14 Fr, 15–20 Fr, or >20 Fr) was performed in one study and demonstrated no difference in mortality or need for surgery based on chest tube size (89). In general, a larger-bore catheter has a theoretical advantage of facilitating effective drainage. However, this must be balanced against the increased pain associated with larger-bore tube insertion, which could compromise a patient with a tenuous respiratory status. When using smaller-bore tubes, the authors urge all practitioners to develop protocols to assure catheter patency. This approach would include routine checks to identify catheter kinking including careful anchoring at the entry site, routine catheter flushes, and connection to a closed pleural drainage system with continuous, regulated suction.
Evidence of pus, loculations, pleural thickening, and progressive organization in the chest would suggest that less invasive drainage procedures may be unsuccessful. These are the patients in whom intrapleural fibrinolytic therapy should be considered based on the results of the MIST2 trial, a four-arm double-blinded, double-dummy study of tPA plus placebo, deoxyribonuclease (DNase) plus placebo, combined tPA and DNase, or double placebo. Unlike the outcome in the MIST1 trial, the MIST2 study demonstrated improvement in pleural opacity in the tPA–DNase group compared with either agent alone or placebo. Combined therapy also demonstrated a reduction in surgical referral and length of hospital stay (90). Consequently, we recommend that, in a stable patient, chest tube drainage including fibrinolytic and DNase therapy (twice daily intrapleurally for 3 days) be considered first, reserving surgical intervention for clinical or radiographic fibrinolytic failure (72–75).
Postcardiac Injury Syndrome
Postcardiac injury syndrome (PCIS) is characterized by the onset of fever, pleuropericarditis, and parenchymal infiltrates typically 3 weeks (2–86 days) following injury to the myocardium or pericardium (91,92). PCIS includes different pleuropericardial syndromes that are elicited by an initial traumatic trigger affecting the pericardium/myocardium and/or pleura after cardiac surgery, an invasive percutaneous intervention, myocardial infarction, or chest trauma. The incidence following myocardial infarction has been estimated at up to 4%, and up to 30% following cardiac surgery. Based on available data, it appears that PCIS results from an autoimmune reaction following myocardial or pericardial injury (93).
Pleuritic chest pain is reported by virtually all patients, whereas one-half of patients are noted to have dyspnea, fever, pericardial rub, and rales. Half of the patients have a leukocytosis, and almost all have an elevated erythrocyte sedimentation rate. The chest radiograph is abnormal in most patients, with the most common abnormality being left-sided or bilateral pleural effusions (92). Pulmonary infiltrates are present in 75% of patients and are most commonly seen in the left lower lobe (91). The pleural fluid is a serosanguineous or bloody exudate with pH greater than 7.30 and glucose level greater than 60 mg/dL. Nucleated cells range from 500 to 39,000 cells/μL, with a predominance of PMNs early in the course (19). The finding of pericardial fluid on echocardiogram suggests PCIS. The diagnosis is made clinically after pulmonary embolism and parapneumonic effusion have been excluded. An antimyocardial antibody titer in pleural fluid greater than in serum further supports the diagnosis (94).
PCIS is usually self-limited and may not require treatment if symptoms are minor. Spontaneous recovery occurs in 66% of patients. Horneffer et al. conducted a trial for treatment of PCIS, comparing ibuprofen 600 mg orally four times per day, indomethacin 25 mg orally four times per day, or placebo for 10 days. The rate of resolution of the postpericardiotomy syndrome was 90% in the ibuprofen group, 89% in the indomethacin group, and 63% in the placebo group (p = 0.003), while recurrences at 30 days were 25% in the ibuprofen and indomethacin groups, and 50% in the placebo group (95). Corticosteroid therapy at low doses (i.e., prednisone 0.2–0.5 mg/kg/day) is useful when aspirin/NSAID are either contraindicated or not well tolerated, and to reduce the possible interference of aspirin/NSAID therapy with oral anticoagulant therapy (96); adjunctive colchicine is also advised in such cases. Following treatment, the pleural effusion resolves within 1 to 3 weeks. It is important to not misdiagnose PCIS as a pulmonary embolism, as anticoagulation therapy may lead to pericardial hemorrhage and tamponade.
Pulmonary Embolism
Pleural effusions occur in up to 50% of patients with pulmonary embolism (86). Pulmonary embolism has been established to be the fourth main cause of pleural effusion in the United States after congestive heart failure, parapneumonic effusion, and malignant effusion (97). The pathogenesis of pleural effusions in pulmonary embolism includes ischemia and inflammatory mediator-induced increased pleural capillary permeability, imbalance in microvascular and pleural space hydrostatic pressures, pleuropulmonary hemorrhage, and atelectasis. With pulmonary infarction, necrosis and hemorrhage into the lung and pleural space may result. More than 80% of patients with pulmonary infarction will have bloody pleural effusions, while up to 40% of patients without radiographic evidence of infarction will also have hemorrhagic fluid (98). Ipsilateral pleuritic chest pain occurs in most patients with pleural effusions complicating pulmonary embolism. A coexistent pulmonary infiltrate is noted on chest radiograph in approximately half of patients with pulmonary embolism and pleural effusion.
Pleural fluid analysis is variable and may demonstrate either an exudate or a transudate (19,99). A bloody pleural effusion in the absence of chest trauma, recent cardiac injury, asbestos exposure, or malignancy should increase the suspicion of pulmonary embolism. The pleural fluid is hemorrhagic in two-thirds of patients, although the number of red blood cells exceeds 100,000 cells/μL in less than 20% (19). The nucleated cell count ranges from less than 100 (presumably atelectatic transudates) to 50,000 cells/μL (pulmonary infarction). When thoracentesis is performed near the time of acute symptoms, PMNs are predominant; with later thoracentesis, lymphocytes represent the majority of cells, and eosinophils may be present as well. The effusion from pulmonary embolism is usually apparent (92%) on the initial chest radiograph and reaches a maximum volume during the first 72 hours. In patients who demonstrate progression of effusions after 72 hours of therapy, recurrent embolism, hemothorax secondary to anticoagulation, an infected infarction, or an alternative diagnosis should be considered. The effusions usually resolve in 1 week in the absence of an infiltrate on chest radiograph. When an infiltrate is present, presumably representing a pulmonary infarction, the resolution time is longer, typically 2 to 3 weeks (98).
The association of a pleural effusion with pulmonary embolism does not alter therapy. The presence of a bloody effusion is not a contraindication to full-dose anticoagulation, since hemothorax is a rare complication of heparin therapy for pulmonary embolism (100,101). An enlarging pleural effusion on therapy necessitates thoracentesis to exclude hemothorax, empyema, or another cause. The development of a hemothorax during therapy requires discontinuation of anticoagulation, chest tube thoracostomy, and placement of a vena cava filter.
Uremia
Uremic pleural effusions have been reported in 3% to 5% of patients undergoing chronic dialysis (102). In one study evaluating the cause of pleural effusions in 100 patients requiring long-term hemodialysis, uremic pleurisy accounted for 16% of cases (103). Patients may manifest fever, cough, chest pain, and pleural friction rubs. The chest radiograph usually shows a moderate unilateral effusion, although massive and bilateral pleural effusions have been reported (104–106). The pleural effusion is a serosanguineous or bloody exudate, with less than 1,500 nucleated cells/μL, predominantly lymphocytes. The creatinine concentration is high, although the pleural fluid to serum creatinine ratio is less than 1.0, unlike that seen in urinothorax (19). The effusions generally resolve with continued dialysis over several weeks but may recur. Uremic pleuritis may cause pleural fibrosis and restriction, requiring decortication in some patients (107,108).
PNEUMOTHORAX IN THE INTENSIVE CARE UNIT
Pneumothorax is defined as air identified within the pleural space and is a commonly encountered problem in the critical care setting. The prevalence of pneumothorax in ICU patients requiring mechanical ventilation ranges from 4% to 15%, (109,110) and it remains one of the most serious complications of positive pressure ventilation. Fortunately, if identified in a timely fashion, pneumothoraces in the ICU can be treated effectively with pleural drainage, minimizing both acute and long-term adverse sequelae. Three pathologic processes may give rise to extra-alveolar air: (a) generation by gas-forming microorganisms during an infectious process, (b) direct introduction following trauma to cutaneous or mucosal barriers, and (c) alveolar rupture due to pressure gradients between alveoli and the surrounding interstitial space (barotrauma) (111).
The mechanisms of spontaneous generation of extra-alveolar air were first delineated by Macklin and Macklin (112). In situations in which intra-alveolar pressure is increased, a gradient is produced between the alveolus and the adjacent vascular sheath, causing the alveoli to rupture at their bases. Following rupture, air is introduced in the perivascular adventitia, resulting in interstitial emphysema. The air then dissects proximally to the lung hilum and mediastinum due to a lower mean pressure in the mediastinum compared to that of the lung parenchyma. Once in the mediastinum, the accumulated air may decompress along paths of least resistance into the subcutaneous tissues or, less commonly, into the pericardium, peritoneum, and retroperitoneum. If mediastinal pressure increases abruptly or if decompression via these routes is not sufficient, the mediastinal parietal pleura may rupture, resulting in pneumothorax. Alternatively, air from ruptured alveoli may dissect to the periphery of the lung and rupture via subpleural blebs through the visceral pleura into the pleural space (113).
Pneumothoraces are classified as spontaneous, which occur without preceding trauma or other obvious causes, and traumatic, which occur as a result of direct or indirect trauma to the chest. Spontaneous pneumothoraces can be subdivided into primary spontaneous, which occur in otherwise healthy patients without clinical lung disease, and secondary spontaneous, which occur in patients with underlying lung disease. Traumatic pneumothoraces can be subdivided into the categories of iatrogenic and related to blunt or penetrating chest trauma. In addition, pneumothoraces can be classified as simple or complicated, with complicated pneumothoraces consisting of tension pneumothorax, hemopneumothorax, pyopneumothorax, and open pneumothorax in which the integrity of the chest wall is disrupted. The potential causes of pneumothoraces in critically ill patients are listed in Table 115.2. We will focus mainly on iatrogenic pneumothoraces and pneumothoraces resulting from barotrauma, as these are the most common causes of pneumothoraces in ICU patients.
Diagnostic Evaluation
The diagnosis of pneumothorax in the critically ill patient can sometimes be made with information from the history and physical examination, noting acute onset of dyspnea or chest pain, tachycardia, hypotension, decreased breath sounds, pulsus paradoxus, and contralateral tracheal deviation. Although clinical features can be used to diagnose the presence of a pneumothorax, it should be noted that many of these findings are nonspecific and have not been a reliable indicator of size. As a result, radiologic data remains the gold standard for the diagnosis of pneumothorax (114). Chest radiographs have traditionally been the first test ordered for suspected pneumothorax. In the critically ill, the traditional erect posterior–anterior expiratory film is not practical and thus, the supine or semirecumbent anterior–posterior film is frequently obtained. The radiographic signs of pneumothorax in the supine patient frequently differ from the classic visceral pleural line seen on erect views. In a review of 88 critically ill patients with 112 pneumothoraces, only 22% of pneumothoraces were in the classic apicolateral location (115). In this same study, 30% of pneumothoraces were not detected initially, and of these, half progressed to a tension pneumothorax. The anteromedial position is the most common location for pneumothoraces in the supine patient since this area is the least dependent pleural recess (116). With anteromedial collections of air above the level of the pulmonary hilum, the lucency sharply outlines adjacent vascular structures such as the ascending aorta, superior vena cava, and azygos vein. Below the hilum, the lateral cardiac borders are sharply outlined and paralleled by zones of radiolucency. Increased lucency in the region of the anterior cardiophrenic sulcus may also result from air below the hilar level. Alternatively, air may collect laterally in the costophrenic angle resulting in the classic deep sulcus sign (116).
TABLE 115.2 Causes of Pneumothoraces in ICU Patients |
![]() |
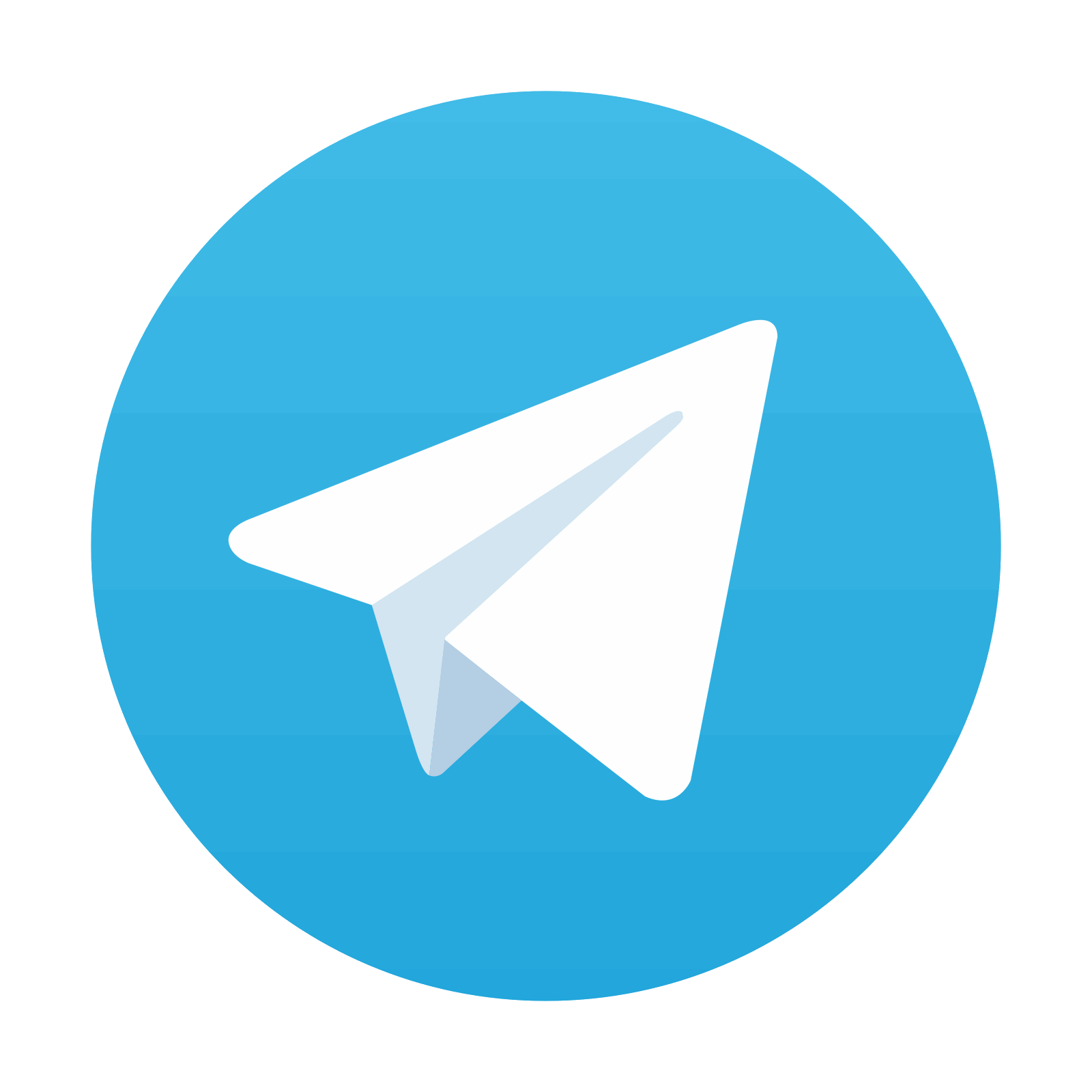
Stay updated, free articles. Join our Telegram channel

Full access? Get Clinical Tree
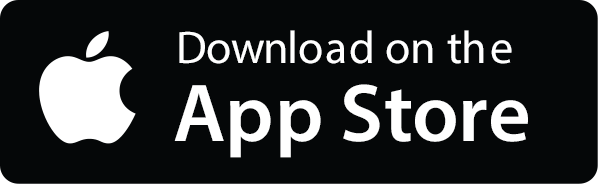
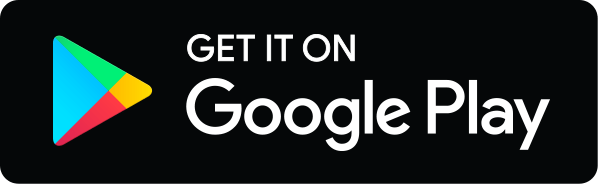