FIGURE 17.1 Schematic depiction of a villus. The villi are foldings of the intestinal epithelium that greatly increase the surface area in contact with the contents within the lumen. They are prominent in the small bowel, where completion of digestion and absorption of nutrients is paramount. Villi are absent in the colon. The cells facing the lumen are composed of a single layer of columnar epithelium consisting of different types of cells. At the base of the villus, stem cells are constantly dividing to produce enterocytes (the most abundant of all cells), goblet cells, neuroendocrine, and Paneth cells. Whereas enterocytes and goblet cells migrate toward the tip of the villus and are shed in just a few days, Paneth cells migrate toward the base (crypt). Goblet cells generate mucous and are most abundant in the colon. Paneth cells produce antimicrobial peptides (AMPs). The tuft cells (not depicted) constitute yet another cell type. Intraepithelial lymphocytes (IEL) can be found in between enterocytes. (From Mowat AM, Agace WW. Regional specialization within the intestinal immune system. Nat Rev Immunol. 2014;14(10):667–685. Reprinted by permission from Macmillan Publishers Ltd.)
Digestion and Absorption
The primary function of the GI tract is to process food, breaking it down mechanically and then, through digestion, convert it into the different substrates that are absorbed and subsequently serve all metabolic processes within the body. The mouth actively participates in the selection of the appropriate quantity and quality of food. Digestion also starts in the mouth with the secretion of amylase and initial breakdown of carbohydrates. In the stomach, the secretion of hydrochloric acid (HCl) by specialized parietal cells and pepsin by chief cells are essential for the initial digestion of protein into peptides. The acid environment also nearly sterilizes ingested food. Further digestion occurs in the duodenum where chyme mixes with bile and exocrine pancreatic secretions. The acid pH of chyme coming from the stomach is neutralized post pylorus by the secretion of bicarbonate. Completion of digestion occurs in the brush border of the small bowel mucosa. In addition, the secretion of fluid into the small bowel dilutes chyme to create an isotonic fluid.
Absorption of digested nutrients proceeds in the small bowel, starting proximally in the duodenum with the absorption of iron, proceeding with the absorption of amino acids, dipeptides, and tripeptides; lipids are broken down by lipases liberating mono- and diglycerides and essential fatty acids, which are surrounded by biliary salts to generate chylomicrons, which are absorbed in the distal ileum. Vitamin B12 is also absorbed in the ileum.
The volume of fluid secreted by the GI tract during digestion can be as high as 9 L; absorption of large amounts of this fluid is initiated in the small bowel. The ascending and transverse colon provide a large amount of absorption of fluid and electrolytes so that only a few 100 mL of water are evacuated in the stool.
Neuroendocrine Regulation
A normally functional GI tract has to be able to sense the amount and quality of the food, determine the rate of progression of the food bolus through the GI tract, communicate with other organs in the body to create the necessary metabolic and physiologic responses to a meal. To do this, highly specialized communication systems with distinct but overlapping functions had to be created.
The central and autonomic nervous systems interact with all anatomic and physiologic sites of the GI tract. For one, conscious issues of hunger and satiety, thirst and the desire for specific nutrients (water, sugar, lipids, salt, and others), which may appear as volitional and hedonistic brain functions, are a result of a careful interaction with the GI tract. Classic examples demonstrate an increase in salivation and release of gastric juices in response to a visual stimulus. Satiety is in part governed by hormones such as ghrelin and GLP1 providing another example for this interaction.
Mechanical progression of food and chyme is determined by peristaltic waves which, while promoting mixing of food and GI secretions, provide a measured aboral “wave” from the GI tract; peristalsis is governed by the autonomic nervous system.
The secretion of digestive enzymes is modulated by hormones secreted by specialized cells in the GI tract. In the stomach, gastrin produced by G cells increases the secretion of pepsin and HCl. In the pancreas, secretion of secretin increases the release of bicarbonate-rich pancreatic juice. Cholecystokinin increases the secretion of bile by the liver and contractility of the gallbladder.
Governing the communication between food, the GI tract, and the rest of the body are chemical messengers with different functions. Attempts to classify these messengers are difficult due to the constant growth and discovery of different substances and also to the overlapping functions of some of these. Nonetheless, a simple classification suggests the following categories:
- Endocrine mediators (hormones). GI hormones are peptides released into the circulation generating local and distant changes in tissue and organ physiology. Five GI hormones are recognized (although there are multiple unique peptides released from GI cells) and include:
- Gastrin
- Cholecystokinin
- Motilin
- Glucose-dependent insulinotropic peptide
- Ghrelin
- Gastrin
- Neurocrine messengers. These messengers, mediating communication between the nervous system and the GI tract, include acetylcholine, vasoactive intestinal polypeptide (VIP), substance P, nitric oxide (NO), cholecystokinin, 5-hydroxytryptamine, somatostatin, and calcitonin gene–related peptide.
- Paracrine messengers mediate local communication between GI cells, and include molecules such as histamine, prostaglandins, somatostatin, and 5-hydroxytryptamine.
- Immune/juxtacrine messengers, as the names implies, mediates communication between the immune system and the GI tract. Some examples are histamine, cytokines, reactive oxygen species, and adenosine (3).
The Immune System of the Gastrointestinal Tract
Diverse immune tissues are distributed across the entire GI tract where they play significant role in the prevention of invasion by microorganisms. Equally as important, proper immune function creates tolerance against antigenic stimuli that come from food and from symbiotic microorganisms, preventing autoimmune responses and self-injury. The immune system in the GI tract is modulated by nutrients such as vitamin A, by antigens of the microorganisms, and by bacterial products such as short-chain fatty acids.
Immune cells are not equally distributed across the GI tract with significant and dramatic variations in concentrations of cells from organ to organ. Thus, for example, the largest concentration of Th17 cells are located preferentially in the duodenum and jejunum. In contrast, the gut-associated lymphoid tissues (GALT) are preferentially distributed in the distal jejunum, ileum, and cecum. This suggests that there are highly different biologic roles in this distribution, which we are only beginning to delineate (1,3).
The GALT deserves special attention. In the distal small bowel, the GALT is organized in histologically distinct zones called Peyer patches. These contain numerous B-lymphocyte follicles which are flanked by smaller T-cell zones. The Peyer patches appear to be the main source of immunoglobulin A (IgA) in the small bowel. In addition, T lymphocytes are distributed across the intestine. Lymphocytes localized at the basement membrane between enterocytes and are called intraepithelial lymphocytes (IELs), and have a wide variety of regulatory and effector activities. Most frequently, IELs are T lymphocytes and are broadly divided into two categories: conventional (type A IELs) and unconventional (type B IELs).
Both helper T cells (CD4+) and cytotoxic T cells (CD8+) are observed in the lamina propria. Most of these T-cell subsets display a memory T phenotype possibly in response to the constant antigenic “sampling” that occurs at the level of the mucosa.
Of growing interest are the so-called innate lymphoid cells (ILCs) distributed across the small and large bowels, that play important and growing roles in the regulation of physiologic responses to antigenic stimuli and may also play pathologic roles during illness. There are three types of ILCs. Of these, the type 2 ILCs (ILC2) appear to play important roles in antigenic sampling. ILC2 cells proliferate in response to interleukin (IL)-25 which is in turn exclusively generated by tuft cells when activated by an antigenic stimulus. ILC2 cells play essential roles in generating type 2 humoral immune responses; ILC2 cells are also controlled by VIP.
Myeloid cells (macrophages and dendritic cells) are also distributed along the GI tract. Macrophages are the most abundant leukocytes in the intestinal lamina propria where they play essential homeostatic roles including phagocytosis, tissue repair, and healing. Intestinal dendritic cells are a distinct subset of dendritic cells as identified by their receptor signature (CD11c+, MHC class II+, CD64–, F4/80–, and expressing ZBTB46).
Disruption of immune responses are independent causative factors of illness. Such is the case of the inflammatory diseases such as celiac disease, Crohn disease, and ulcerative colitis.
The Gut Microbiome
Central to maintaining normal function of the GI tract is a healthy microbiome. While a microbiome exists all the way from the mouth to the anus, the duodenum and jejunum are nearly sterile. The microbial flora in the mouth and colon are distinct from each other and each one contains hundreds to over a thousand distinct bacterial and viral species and archaebacteria. Protozoa and helminths may also exist, although it is only recently that the role of these organisms in the maintenance of health for the overall microbiome has been recognized. Of all microbiomes the colonic microbiome has currently garnered the most interest.
Technologic advances using the tools of molecular biology have permitted a more complete and diverse mapping of the microbiome than otherwise could be afforded by attempting isolated cultures. In 2010, initiatives in the United States and in Europe created a complete mapping of the microbiome in distinct healthy individuals from different geographic, cultural, and genetic backgrounds. In these samples, over 750,000 distinct genes have been identified; thus, our microbiome contains multiple times more genes than our own cells (4).
Neither the type nor the proportion of bacteria is shared by all individuals. There is also an individual variation in the proportion of bacteria in a given individual in response to dietary changes, although there is a striking stability in bacterial species in a given individual across time.
A healthy microbiome exists in a symbiotic relation with its host that involves the creation of a “tolerant” immune response. The host provides food for bacteria, of which dietary fiber is particularly important. The host also provides for a healthy “temperature and oxygen-controlled” environment. In turn, the microbiome generates important nutrients such as short-chain fatty acids, and other micronutrients that feed the gut mucosa and can be absorbed and distributed systemically.
Disruption of the microbiome can occur as a result of multiple conditions. Acute and chronic alterations can occur as a result of diet. These alterations have been linked to obesity, cardiovascular diseases, and inflammatory bowel diseases such as Crohn disease. Acute disruption of the microbiome associated with antibiotic use causes GI intolerance in acute illness and diarrhea. Clostridium difficile colitis constitutes a classic illness associated with a disrupted microbiome, and is now being managed with interventions aimed at restoring microbial homeostasis.
SPECIFIC DISORDERS
Acute Pancreatitis
Acute pancreatitis, a disease process with a wide spectrum of clinical presentations and causes, can challenge any critical care physician. Only 10% to 15% of cases are severe enough to threaten patient survival, and these, therefore, likely involve the critical care physician. Gallstones and alcohol intake cause 85% of the cases of acute pancreatitis (5); the risk in heavy drinkers who also smoke heavily is increased fourfold. Other causes include hyperlipidemia, viral infections, and certain drugs such as propofol (6–8) (Table 17.1).
Of special importance to intensivists is the association between propofol, a medication commonly used for sedation of critically ill patients, and the presence of hypertriglyceridemia and acute pancreatitis. Devlin et al. (6) retrospectively studied 159 patients in the intensive care unit (ICU) with propofol sedation, finding that 29 (18%) patients developed hypertriglyceridemia and, among these 29 patients, three presented a clinical picture of acute pancreatitis. Their final recommendation was to monitor the serum triglycerides levels and pancreas enzymes after 48 hours on propofol.
The diagnosis of acute pancreatitis requires two of the following three criteria: (1) Abdominal pain consistent with acute pancreatitis; (2) pancreatic enzyme elevation to greater than three times the upper limit of normal; and (3) findings characteristic of acute pancreatitis on contrast-enhanced computed tomography (CT) of the abdomen (9). Thus, the usual initial workup for a patient with symptoms consistent with acute pancreatitis is to obtain serum amylase and lipase values and, generally, obtain an abdominal CT (see below). Severity of the disease may be defined using the guidance of the revised Atlanta criteria (Table 17.2). Note, however, that the degree of enzyme elevation at admission does not necessarily forecast eventual disease severity (10).
Acute pancreatitis due to pancreatic duct obstruction—as from a gallstone obstructing the duct—triggers the activation of endogenous pancreatic enzymes such as trypsin, causing autolysis and activation of the inflammatory response which may progress to organ failure (11). Alcohol-induced pancreatitis is more complex, inducing acinar cell dysfunction that leads to precipitation of secretions in the ducts, obstructing outflow (12).
TABLE 17.1 Classification System of Drug-Induced Acute Pancreatitis |
![]() |
TABLE 17.2 Grades of Severity of Acute Pancreatitis |
![]() |
Bacterial seeding of necrotic pancreatic tissue can occur, most probably through bacterial translocation (BT) from the gut, and may lead to sepsis and delayed death (13); a severe aseptic inflammatory response to pancreatitis may be difficult to differentiate from a septic response due to bacterial contamination. Ideally, in all cases of severe acute pancreatitis, the extent of necrosis should be determined using the gold standard to make such determinations, contrast-enhanced CT. Furthermore, the CT can identify other life-threatening causes of SIRS and shock not uncommonly seen in such critically ill individuals, such as major bleeding and/or hollow viscus erosion/perforation.
After the first 2 to 4 weeks of the disorder it is often seen that initially sterile peripancreatic inflammatory fluid collections coalesce into structures known as pseudocysts, which often regress spontaneously, but may persist and become infected, warranting drainage. The patient may show a persistent inflammatory state, and can deteriorate with SIRS, sepsis, or septic shock. Although the presence of air bubbles in the pancreas on abdominal CT scan suggests infection, the gold standard to rule out this possibility is CT-guided needle aspiration of the necrotic pancreatic bed. Intervention, however, is not without hazard: “routine” drainage of pancreatic fluid collection often leads to infection in previous sterile collections (14), and thus the suspicion of infection must be convincing before intervention for drainage is undertaken. A variety of modalities of treatment exists to address infected fluid collections, ranging from percutaneous drainage to endoscopic procedures to minimally invasive or open necrosectomy. Studies of patients managed with percutaneous procedures have shown varying results (15). Transgastric drainage guided by endoscopic ultrasound is ideal in certain clinical situations (15), and may compare favorably with surgical intervention as experience develops (16). Surgical choices include the so-called VARD (video-assisted retroperitoneal debridement) technique with single or repeated procedures for removal of necrotic pancreatic tissue, or open complete necrosectomy with drain placement and continuous irrigation in the postoperative period (17). Clearly, with this variety of choices available for treatment, the decision for optimal treatment of a specific patient with acute pancreatitis must be individualized.
Clostridium difficile Colitis
The selective pressure of antibiotic use may lead to the disruption of normal fecal flora allowing the emergence of resistant organisms and causing disease. Best known of these organisms is C. difficile, which can cause diarrheal outbreaks in health care institutions. The emergence of a hypervirulent strain of C. difficile known as NAP1/B1/ribotype 027 which produces both toxins A and B—and is frequently fluoroquinolone-resistant—has been a problem of particular importance in ICUs in many countries. C. difficile colitis can be a lethal disease particularly if treatment is delayed or inadequate. There is an increased risk of developing severe C. difficile colitis in the chronically ill, the elderly, and immunosuppressed patients. Early identification and treatment are critical, and the presence of significant leukocytosis should trigger the possibility of such a diagnosis. The severity of disease ranges from asymptomatic carriage, through diarrhea that is mild in frequency and severity, up to a fulminant and rapidly progressive toxic state with profound leukocytosis, hypotension, hypoalbuminemia, and lactic acidosis; the latter condition warrants the most aggressive treatment regimen which often includes emergent surgery (18). Significant abdominal findings warrant radiologic investigation. An initial abdominal radiograph—a KUB (kidney, ureters, and bladder) study—may reveal significant small intestinal or colonic dilatation with pneumatosis in the colonic wall; abdominal CT scan is useful to assess colon integrity and to rule out other pathologies. Definitive diagnosis can be made using one of a variety of laboratory tests. However, the selected test should only be performed in individuals with diarrhea so as to avoid treatment of asymptomatic carriers (19). Combination testing designs including immunoassay for C. difficile glutamate dehydrogenase antigen followed by a rapid toxin A/B assay in screen-positive samples is a 92% accurate tactic with rapid turnaround (20). Polymerase chain reaction testing for C. difficile toxin genes is rapid with high sensitivity and specificity (21). It is important to remember that laboratory tests cannot distinguish between colonization and infection so it is important that diagnostic testing and treatment be made within the appropriate clinical context (22). The current mainstay of medical treatment includes the discontinuation of the inducing antibiotics, if possible (23) and the immediate initiation of oral vancomycin and/or metronidazole—the latter given either intravenously or orally. More serious disease warrants use of high-dose oral vancomycin, vancomycin retention enemas, and possibly fidaxomicin, although the expense of this new medication may limit its availability. The reader is referred to a recent review of treatment recommendations for more detail (24). Aggressive fluid resuscitation—guided by careful monitoring of the clinical condition and volume status—and timely surgical intervention, since this disease entity can progress rapidly to the point of need for surgery, are important to decrease mortality. Despite aggressive treatment of C. difficile infection (CDI), however, morbidity from this disease and a significant incidence of recurrence continue to be a problem. Adjunctive treatments such as dietary manipulations, the use of probiotics and toxin-binding agents, and restoration of the colonic flora through the use of fecal transplant are all treatments that are being investigated, although their exact roles are unclear (25). Fecal microbiota transplantation, the administration of fecal flora directly to the lumen of the colon or via a nasogastric (NG) or nasojejunal (NJ) tube to replete the eradicated normal gut bacterial flora, is sometimes used; it appears to have positive effects in recurrent CDI, although with low strength evidence (26). Effectiveness of administration via NG/NJ tube or rectally via colonoscope appears to be equal (27). Some promising findings that warrant further study have been seen in investigations of treatment of CDI with rifampin, rifaximin, nitazoxanide, tigecycline, monoclonal antibodies, and immunoglobulins (28).
Abdominal Compartment Syndrome
Increased intra-abdominal pressures (IAP) compromising blood flow to splanchnic organs have been described in an increased percentage of patients in the ICU. Elevated IAP can have a major negative impact on the functioning of all major body systems that are vital for life (29). Malbrain et al. from the European Community analyzed 265 consecutive patients in the ICU, measuring IAP via transduction of the urinary bladder; this work demonstrated that nonsurvivors tended to have higher IAP (30). Furthermore, patients with prior elevated IAP exhibited increased sepsis-related organ failure assessment (SOFA) scores. In an Argentinian study of 83 critically ill patients, 54% displayed intra-abdominal hypertension (IAH) (IAPmean >12 mmHg) during ICU admission, with significantly higher ICU length of stay and hospital mortality (31). The 2013 consensus definitions from the World Society of Abdominal Compartment Syndrome are these: (1) Normal IAP is 5 to 7 mmHg in critically ill adults, as measured using 25 mL of distilled water instilled into the bladder; (2) IAH exists when the sustained or repeated IAP is greater than 12 mmHg; abdominal compartment syndrome (ACS) exists when the sustained IAP is greater than 20 mmHg and/or abdominal perfusion pressure ([mean arterial pressure] MAP–IAP) is less than 60 mmHg and associated with new organ dysfunction/failure (32). Severity is graded from one to four, based upon the IAP, with recommendations for management steps at each grade. A variety of risk factors for this condition exist. Management obviously involves reduction of the pathologically elevated IAP, most often by fluid removal, sedation and chemical paralysis, optimal patient positioning, decompression by NG drainage and, sometimes, by surgical decompression—decompressive laparotomy. Determining when abdominal decompression to improve splanchnic organ perfusion should be done remains controversial and is partially subjective (33). While this procedure can be life-saving, it is associated with considerable morbidity and mortality in both the short and long time frames.
Acute Mesenteric Ischemia
The splanchnic organs are perfused by three major arterial systems: those vessels radiating from the celiac axis (the left gastric, common hepatic, and splenic arteries) and perfusing the liver, stomach, and spleen; the superior mesenteric artery (SMA), supplying most of the small bowel and the right side of the colon; and the inferior mesenteric artery (IMA), supplying the left side of the colon, sigmoid, and superior portion of the rectum. Acute mesenteric ischemia may be caused by several conditions, such as classic arterial occlusion due to embolism (40% to 50% of cases), atherosclerosis, states of low cardiac output due to shock—including cardiogenic shock—mesenteric venous occlusion or, less frequently, arterial dissection, vasculitis, or nearby inflammatory conditions (34).
Manifestations of acute mesenteric ischemia may range from subtle findings of mild abdominal distention and/or mild pain to those of a devastating disease process of peritonitis, hypotension, circulatory collapse, and death. Reperfusion of the splanchnic organs with restoration of circulation to the affected organs may provoke a dramatic systemic inflammatory response (35). Difficulty in making a swift and accurate diagnosis, and in formulating and executing timely therapy, most often immediate surgical intervention, leads to staggering mortality: 60% to 80% (36).
Physical, laboratory, and radiologic findings in the patient with acute mesenteric ischemia can be difficult to analyze accurately. The presence of severe abdominal pain with few abdominal findings on physical examination in an individual (very often elderly) with risk factors is suggestive of mesenteric ischemia, and warrants immediate and aggressive investigation of this possibility. Patients with this condition—often sedated and mechanically ventilated ICU patients—making accurate abdominal examination quite difficult. Others may present with sudden overt peritoneal signs leading to pursuit of other acute abdominal conditions and delay in diagnosis. Laboratory investigation may reveal hemoconcentration and/or an otherwise unexplained metabolic acidosis or elevated lactate suggesting that progression to bowel injury has occurred. Occasionally, brisk lower GI tract bleeding or, more subtly, heme-positive stools may be discovered. Plain abdominal films are less specific, findings ranging from normal to the demonstration of ileus, portal vein air, air in the colonic wall, or free intraperitoneal air (Figs. 17.2 and 17.3). A contrast-enhanced abdominal CT scan is useful as it may identify the precise location of the compromised vessel (artery or vein) and the extent of visceral damage. Ominous signs such as air in the portal vein, the bowel wall, or free air in the peritoneal cavity may be seen. Bedside diagnostic laparotomy/laparoscopy is an option for patients too unstable to be moved for a radiologic study. Recently, treatment of acute mesenteric ischemia has evolved into a treatment strategy combining surgical and endovascular techniques. In suitable candidates, vascular occlusion of the SMA may be treated using catheters placed through the femoral or brachial artery to perform aspirative SMA embolectomy, SMA thrombolysis with recombinant tissue plasminogen activator (rtPA), or recanalization and stenting of the SMA. Such a procedure may be performed in combination with surgical intervention (37,38).

FIGURE 17.2 This abdominal plain film shows the presence of air inside the portal vein, a classic sign for mesenteric ischemia (arrows).
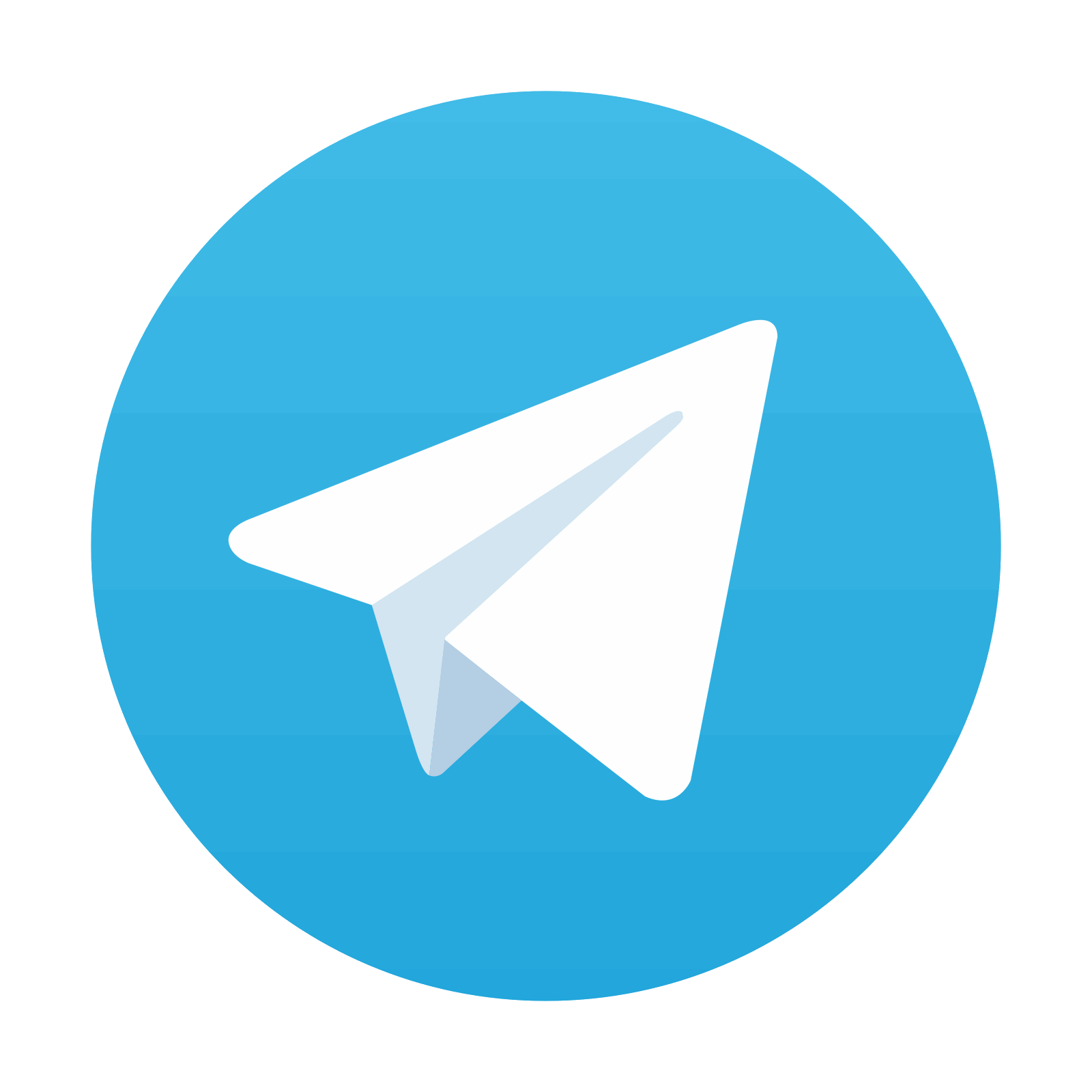
Stay updated, free articles. Join our Telegram channel

Full access? Get Clinical Tree
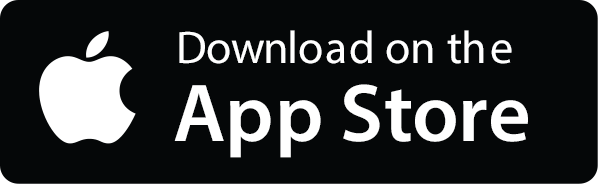
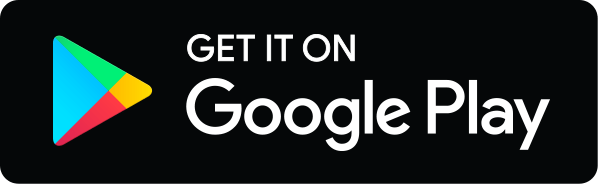