Abstract
This chapter reviews the clinical pharmacology, therapeutic mechanisms, and side effects of corticosteroids, botulinum toxins, radiocontrast agents, and topical antiseptics. Corticosteroids are the most potent and effective agents in controlling inflammation through numerous mechanisms, including effects on cytokines, inflammatory mediators, and others. Several synthetic steroid preparations are available and widely used for interventional pain procedures. They vary in their antiinflammatory potencies, mineralocorticoid activities, solubility, and durations of action. Botulinum toxins are produced by the gram-negative anaerobic bacterium Clostridium botulinum . They produce flaccid paralysis by preventing the presynaptic release of acetylcholine (Ach) at the neuromuscular junction. Two botulinum neurotoxins, types A and B, are used in clinical practice. In addition to the pain relief associated with its paralytic effect, botulinum toxin A (BoNT-A) has been shown to influence pain processing via reduction in peripheral and central sensitization through a number of different mechanisms. Interventional physicians routinely use radiocontrast media (RCM) agents during various pain procedures; it serves as confirmation of correct needle placement, and it also helps the proceduralist with the visualization of the spread of medication during therapeutic or diagnostic injections. The majority of interventional pain procedures are performed with percutaneous needle placement. The use of antiseptic solutions is instrumental in preventing infectious complications when performing peripheral and neuraxial pain procedures. A comprehensive understanding of pharmacologic agents used by the interventional pain physician is vital for safe and successful patient care.
Keywords
antiseptic agents, corticosteroids, interventional pain, pharmacology, radiographic contrast
A comprehensive understanding of pharmacologic agents used by the interventional pain physician is vital for safe and successful patient care. This chapter reviews the clinical pharmacology, therapeutic mechanisms, and side effects of corticosteroids, botulinum toxins (BoNT), radiocontrast agents, and topical antiseptics.
Corticosteroids
Naturally occurring corticosteroids are classified into three functional groups: (1) mineralocorticoids, (2) glucocorticoids, and (3) adrenal androgens. Mineralocorticoids maintain normal fluid and electrolyte balance. Glucocorticoids act primarily to enhance the production of high-energy fuel, glucose, and reduce other metabolic activity. Injections of glucocorticoids for the relief of vertebrogenic, arthritic, and radiculopathic pains are widely accepted. There are many proposed mechanisms of action that are believed to underlie the therapeutic use of corticosteroids. These actions include antiinflammatory effects, modulation of peripheral nociceptor neurons and spinal cord dorsal horn cells, and direct stabilization of neural membranes.
Corticosteroids are the most potent and effective agents in controlling inflammation through numerous mechanisms, including effects on cytokines, inflammatory mediators, inflammatory cells, nitric oxide synthase, and adhesion molecules. Cytokines are important mediators of inflammation, and the pattern of their expression largely determines the magnitude and duration of the inflammatory response. Steroids have inhibitory effects on cytokine transcription and synthesis, especially the ones relevant in chronic inflammation (interleukin [IL]-1, IL-3, IL-4, IL-5, IL-6, IL-8, tumor necrosis factor [TNF]-α, and granulocyte-macrophage colony-stimulating factor). Steroids not only inhibit cytokine synthesis, but also interfere with their activity. Steroids increase the synthesis of lipocortin (annexin) 1, a phospholipase A 2 inhibitor, and thus decrease the production of inflammatory mediators, such as leukotrienes, prostaglandins, and platelet-activating factor. Corticosteroids interfere with macrophage activity by impairing phagocytosis, intracellular digestion of antigens, and macrophage release of IL-1 and TNF-α. By inhibiting the expression of chemokines, corticosteroids prevent the activation and recruitment of inflammatory cells, including eosinophils, basophils, and lymphocytes. In addition, steroids cause an expansion in the number of circulating neutrophils secondary to decreased adherence to vascular endothelium (demargination) and stimulation of bone marrow production. Corticosteroids interfere with T-cell-mediated immunity. They inhibit the production of T lymphocytes by downregulating T-cell growth factors IL-1β and IL-2, and inhibit the release of various T-lymphocyte cytokines.
Other mechanisms of action for injected corticosteroids include suppression of spontaneous ectopic neural discharge originating in experimental neuromas. Following peripheral nerve injury, a number of morphologic and biochemical changes occur at the injury site, including the formation of neuromas, which leads to increased electrical excitability. Ectopic discharge from the injury site leads to a persistent afferent barrage, which maintains neuralgic pains and paresthesias. Furthermore, the topical application of methylprednisolone has also been shown to reversibly block transmission of C-fibers but not the A-β fibers. Together, these data support the notion that locally applied corticosteroids suppress afferent ectopic nociceptive discharges, suggesting a direct membrane-stabilizing effect. Lastly, glucocorticoid receptor sites have been found on 5-hydroxytryptamine (5-HT) and noradrenergic neurons within the dorsal horn substantia gelatinosa. These are known pathways of pain transmission, and this suggests that corticosteroids may possibly modulate nociceptive transmission by a direct action on the spinal cord.
Several synthetic steroid preparations are available and widely used for interventional pain procedures. They vary in their antiinflammatory potencies, mineralocorticoid activities, solubility, and durations of action. It should be noted that none of the available preparations have been approved for perineural or epidural administration by any regulatory agency in the United States or internationally.
Short-acting synthetic steroids have durations of action of 8 to 12 hours; these include the active agent hydrocortisone and the inactive cortisone (converted by the liver to the biologically active cortisol). The intermediate-acting steroids, prednisone, prednisolone, methylprednisolone, and triamcinolone, have durations of action of 24 to 36 hours. Prednisone is an inactive agent, which is metabolized to the active agent prednisolone by the liver. The longest acting steroids, dexamethasone and betamethasone, have durations of action longer than 48 hours. Although the choice of steroid used by interventional pain physicians is frequently based upon its antiinflammatory potency and duration of action, the steroid particulate size relative to a red blood cell and whether or not it aggregates has become major determinants of corticosteroid selection given reported catastrophic neurological sequelae as described elsewhere in this text. Table 55.1 provides a summary of the properties of commonly used injectable corticosteroids.
Steroid | Commercial Name | Equivalent Potency (mg) | Relative GC Potency | Solubility | Maximum Particle Size (μm) | Particles > 10 μm (%) | Particle Aggregates |
---|---|---|---|---|---|---|---|
Methyprednisolone acetate | Depo-medrol, solu-medrol | 4 | 5 | 0.001 | >500 | 45 | Extensive |
Triamcinolone acetonide | Kenalog | 4 | 5 | 0.0002 | >500 | 45 | Extensive |
Betamethasone acetate, betamethasone sodium phosphate | Celestone soluspan, betaject | 0.75 | 33 | Acetate form: “practically insoluble.” Sodium phosphate form:freely soluble | 500 | 35 | Some |
Dexamethasone sodium phosphate | Decadron phosphate, adrenocort, decaject | 0.75 | 27 | Freely soluble | 0.5 | 0 | None |
The majority of systemic adverse effects from corticosteroid administration are usually mild and short-lived when they are administered as intermittent injections, as is common in interventional pain management procedures. This is in contrast to long-term supraphysiologic administration of steroids, where more serious side effects may occur. Side effects from short-term therapy are rare, but may include fluid retention (if higher mineralocorticoid activity steroids are used), hyperglycemia, elevated blood pressure, mood changes, insomnia, menstrual irregularities, gastritis, Cushing syndrome, increased appetite, weight gain, increased infections, delayed wound healing, bone demineralization, and acneiform eruptions.
Sterile meningitis and arachnoiditis have been reported following intrathecal injection of methylprednisolone, but may have been related to the polyethylene preservative. Anaphylactoid and hypersensitivity reactions are rare, but have been reported following intramuscular and soft-tissue injections. Any type of anaphylactic reaction warrants prompt and aggressive life-support therapy, including resuscitation of airway, breathing, and circulation, with oxygen support and cardiac life support when indicated.
Most studies showed increased blood glucose levels after an epidural steroid injection of triamcinolone or betamethasone. There appears to be no correlation between preinjection hemoglobin A1c levels or patient age with the changes in blood glucose. In most patients, the blood glucose returned to baseline 7 days later. Another study showed a minimal effect of epidural methylprednisolone. The depot steroid preparations used for epidural injections may produce adrenocorticotropic hormone (ACTH) suppression and cushingoid symptoms that can last up to a few weeks. Cushing syndrome has occurred following a single epidural injection of 60 mg of methylprednisolone or triamcinolone, and has been reported in several patients following repeated epidural steroid injections when 200 mg of methylprednisolone was exceeded. Lumbar epidural injection of triamcinolone, 80 mg, caused profound hypothalamic, pituitary, adrenal (HPA) axis suppression for 3 weeks, although a steroid was undetectable in the plasma during this time. Comparable studies of patients who have received intraarticular steroid injections have shown detectable levels in the circulation and HPA axis suppression for up to 4 weeks.
Epidural injection of triamcinolone, 80 mg, caused a marked reduction in insulin sensitivity in patients with normal glucose tolerance and caused fasting hyperglycemia in patients with a preexisting degree of insulin resistance. In this study, insulin sensitivity and fasting glucose levels were normal 1 week after injection. Because patients with diabetes commonly experience increased insulin requirements for several days following the injection, it is suggested that they be given specific advice on the management of their condition following epidural steroid injection.
The overall safety of fluoroscopically guided epidural steroid injections has been confirmed in a retrospective study. However, there have been several reported cases of central nervous system injuries after transforaminal epidural steroid injections, and these complications are discussed in Chapter 63 .
Botulinum Toxin Therapy
BoNTs are produced by the gram-negative anaerobic bacterium Clostridium botulinum. They produce flaccid paralysis by preventing the presynaptic release of acetylcholine (ACh) at the neuromuscular junction. There are eight BoNT subtypes—A, B, C 1 , C 2 , D, E, F, and G. The US Food and Drug Administration (FDA) approved the use of onabotulinum toxin A for the treatment of strabismus, blepharospasm, and hemifacial spasm. In 2000, BoNT B (Myobloc) was approved by the FDA for treating cervical dystonia, and 10 years later the agency approved the use of onabotulinum toxin A (Botox or Botox Cosmetic) in chronic migraine treatment. The type A subtypes appear to be the most potent and has the longest duration of action.
BoNT is synthesized as a single-chain polypeptide, consisting of a heavy (H) chain (molecular weight [MW], 100,000) and a light (L) chain (MW, 50,000). The H chain is responsible for binding to presynaptic cholinergic nerve terminals at the neuromuscular junction, whereas the L chain is the neurotoxic component. The H and L chains are bound together by disulfide bonds, and the toxin is activated by proteolytic enzymes in a cleaving process. It is estimated that the human lethal dose of BoNT-A is about 2800 to 3500 units for a 70-kg adult. The lethal dose of BoNT-B in humans is estimated at 144,000 units.
Two botulinum neurotoxins, types A and B, are used in clinical practice. There are three commercially available type A preparations, Botox, Dysport, and Xeomin. Each vial of Botox (Allergan, Inc., Irvine, California) contains 100 units of onabotulinum toxin A as a sterile, vacuum-dried form without preservative that can be reconstituted with preservative-free sterile saline prior to injection. Dysport (abobotulinum toxin A) (Ipsen, Ltd., Berkshire, UK) is marketed in the form of 500-unit vials. Data from patients treated for cervical dystonia suggest that 1 unit of Botox equals 3 to 5 units of Dysport. Xeomin (Merz Pharmaceutical, Frankfurt AM Main, Germany) is marketed as 100 units of incobotulinum toxin A powder, reconstituted upon use in preservative-free sterile normal saline. Myobloc (Solstice Neurosciences Inc., Malvern, Pennsylvania) is marketed as a sterile liquid formulation of a purified BoNT B (rimabotulinum toxin B).
BoNT A (BoNT-A) in the Botox formulation, the commercially available product in the United States, is inactivated by heat, shaking, excessive dilution, and surface tension from bubbles during reconstitution. Boiling dissolves the disulfide bonds between the H and L chains of BoNT-A, thus rendering the toxin inactive, because neither chain can exert neurotoxicity independently. BoNT-A must be reconstituted with preservative-free sterile normal saline. Dilution of a 100-unit vial may be performed with 1, 2, 5, or 10 mL of 0.9% sodium chloride. This will yield a concentration of 10, 5, 2, or 1 units/0.1 mL, respectively. The higher concentrations are appropriate for larger muscles; for example, hip flexors or piriformis muscles. Lower concentrations are used for facial injections, for example, for the glabella, temporalis, and frontalis muscles.
BoNT-A should be used within 4 hours of preparation and stored at 2°C to 8°C during this time. It has been shown that there is no loss of activity 6 hours after reconstitution at room temperature; however, a 44% loss of activity is observed at 12 hours. Refreezing the toxin after reconstitution causes a 70% reduction in bioactivity at 1 to 2 weeks.
BoNTs act by blocking the presynaptic release of ACh from cholinergic terminals of motor and autonomic nerves. BoNT neurotoxicity occurs in three stages: binding, internalization, and proteolysis. Following activation by proteolytic cleavage, the BoNT H chain binds irreversibly to the presynaptic terminals of cholinergic neurons. The C-terminal region of the H chain binds in a serotype-specific manner to receptors on the motor end plates and at autonomic cholinergic ganglia.
In addition to the pain relief associated with its paralytic effect, BoNT-A has been shown to influence pain processing via reduction in peripheral and central sensitization through a number of different mechanisms. BoNT-A has been shown to inhibit the release of pain peptides, substance P, calcitonin gene-related peptide (CGRP), glutamate, and bradykinin from the trigeminal and dorsal root ganglia. This mechanism is modulated by the synaptosomal-associated protein 25 (SNAP-25) located within the cell membrane. In a formalin model of pain, injection of BoNT-A into a rat paw one week prior to formalin injection, reduces the postformalin inflammatory peak of pain in a dose-related manner. Tissue examination of the injected site reveals decreased inflammation and a reduction of local glutamate accumulation compared with controls. Additionally, BoNT-A inhibits a family of G proteins, including Rho guanosine triphosphatase, which is necessary for activation of IL-1, an important proinflammatory cytokine. Finally, BoNT-A has been shown to affect cyclooxygenase-2 expression, impair sympathetic transmission of pain, and reduce allodynia in diabetic rats, suggesting a central analgesic effect of BoNT-A.
The BoNT molecule cannot cross the blood-brain barrier, and therefore does not have any direct central nervous system (CNS) effects. At the neuromuscular junction, BoNTs cause a chemical denervation, thereby inhibiting skeletal muscle contraction. In humans, clinical effects typically appear after 2 to 3 days, but the peak effects are observed at 2 to 6 weeks. BoNT-induced chemical denervation is permanent, so skeletal muscle remains paralyzed until new axons and synapses have formed to reestablish the neuromuscular junction. Functional denervation is observed for 6 weeks up to 6 months following injection, but usually lasts for 3 to 4 months.
Repeated injections of onabotulinum toxin A have been associated with antibody formation, which renders any subsequent onabotulinum toxin A injections ineffective. A study of 32 patients with spasmodic torticollis treated with repeated onabotulinum toxin A injections has revealed that four patients (12.5%) produced antibodies after 2 to 9 months of treatment. It appears that higher toxin doses and frequent injections are the leading factors in the development of neutralizing antibodies.
Reported complications of BoNT injection include injection site reaction, pain at the injection site, headache, fatigue, flulike symptoms, muscle weakness, fever, pruritis, and significant regional sequelae depending on the injection site (i.e., dysphagia, facial paralysis). Muscle weakness occurs if more than 50 units of onabotulinum toxin A are injected into a muscle.
The use of BoNT-A for analgesia in humans has been well studied and extensively reported with regard to the treatment of cervical dystonia. For those with cervical dystonia, BoNT (serotype A or B) significantly reduces neck pain in 60% to 80% of patients. Other prospective and placebo-controlled studies using BoNT in humans reported efficacy and improved pain in patients with myofascial pain syndrome, plantar fasciitis, pelvic pain, migraine headaches, lateral epicondylitis, postherpetic neuralgia, low back pain, and piriformis syndrome. Recent studies also showed improvement in neuropathic pain and allodynia associated with painful diabetic neuropathy after intradermal injections of BoNT-A.
Jabbari and Machado conducted an evidence-based review in which they used American Academy of Neurology methodology as stated by the guidelines of the society’s Therapeutics and Assessment Subcommittee to rate the scientific evidence for the use of BoNTs in clinical practice. They found four levels of clinical evidence in the use of BoNT in painful clinical conditions ( Table 55.2 ).
Level of Evidence | Recommendations | Clinical Condition |
---|---|---|
A | Established and recommended | Cervical dystoniaChronic migraineChronic lateral epicondylitis |
B | Probably effective, should be considered for treatment | Postherpetic neuralgiaPosttraumatic neuralgiaPlantar fasciitisPyriformis syndromeTotal knee arthroplasty |
C | Possibly effective; can be used at the discretion of the physician | Allodynia in diabetic neuropathyChronic low back painKnee osteoarthritisAnterior knee pain with vastus lateralis imbalancePelvic painPostoperative pain in children with cerebral palsyPostoperative pain after mastectomySphincter spasm and pain after hemorroidectomy |
U | Insufficient evidence due to contradictory results | Myofascial pain syndromeChronic daily headaches |
Radiographic Contrast Media
Interventional physicians routinely use radiocontrast media (RCM) agents during various pain procedures; it serves as confirmation of correct needle placement; it also helps the proceduralist with the visualization of the spread of medication during therapeutic or diagnostic injections.
The first contrast agent used in medicine for the study of neural structures was sodium iodide as a 40% solution suspended in the oil of poppy seeds. The product was very insoluble and carried significant side effects, such as severe local irritation, nausea and vomiting, arthralgias, lymph node swelling, and hives, and had a very low radiographic yield. In 1927, the reported concentration of this RCM was reduced to 20% and although still with a low radiographic yield, the physicians were able to diagnose a brain abscess with a reported decrease in side effects from the agent.
The following decades brought some new improvements in RCMs. In the 1950s, sodium iodide was replaced by sodium, calcium, or methylguanine diatrizoic acid salts; those agents had high osmolarity of greater than 1700 mOsm (5 to 8 times that of blood), and were therefore called high osmolar contrast media (HOCM). HOCM have a ratio of 3:2 (iodine to particle) and dissociation of those two components commonly occurred. Adverse events related to HOCM, such as hypertension, cardiac arrhythmias, or fluid overload, were commonly present.
Low osmolar contrast media (LOCM) were not available for clinical use until the late 1970s. Research and ultimate discovery was fueled by goals to increase the safety profile by decreasing the significant and commonly present adverse events with existing RCMs. With an osmolality of less than 850 mOsm and an iodine to particle ratio of 3:1, which allowed for less dissociation, these products were made from benzoic acid side chains. Despite improvements, adverse events such as nausea, angina, and anaphylaxis still occurred.
In the 1980s, RCM agents with low osmolar properties, less lipophilicity, and less toxicity were available for use. The improvements were due to the use of monoacid dimers with two benzoic rings containing iodine at positions 2, 4, and 6 bound together to form nonionic compounds.
The only iso-osmolar RCM agent was introduced in the mid-1990s, iodixanol, with an iodine content of 300 mg/mL, which nearly matches that of blood.
Commonly used RCM agents are divided in ionic and nonionic, and each category is subdivided into monomers and dimers. Table 55.3 presents a summary of the physical properties of the commonly used RCMs.
Name | Ionicity/Form | Type | Iodine Concentration (mg/mL) | Osmolality (mOsm/kgH 2 O) |
---|---|---|---|---|
Iodixanol | Nonionic dimer | IOCM | 320 | 290 |
Iomeprol | Nonionic monomer | LOCM | 350 | 620 |
Ioxaglate | Ionic dimer | LOCM | 350 | 680 |
Ioxilan | Nonionic monomer | LOCM | 350 | 695 |
Iopamidol | Nonionic monomer | LOCM | 350 | 730 |
Iopromide | Nonionic monomer | LOCM | 350 | 730 |
Iohexol | Nonionic monomer | LOCM | 350 | 780 |
Ioversol | Nonionic monomer | LOCM | 350 | 790 |
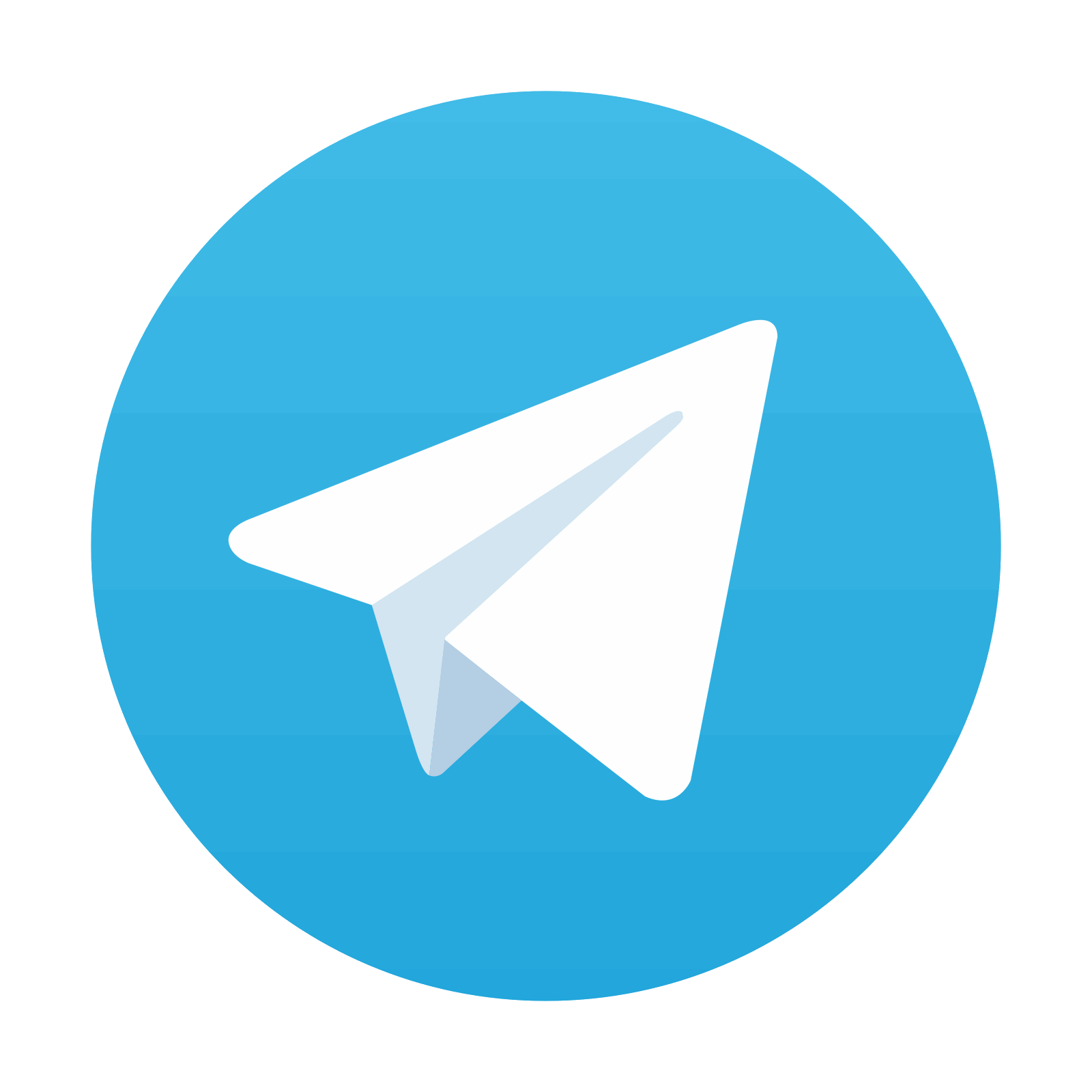
Stay updated, free articles. Join our Telegram channel

Full access? Get Clinical Tree
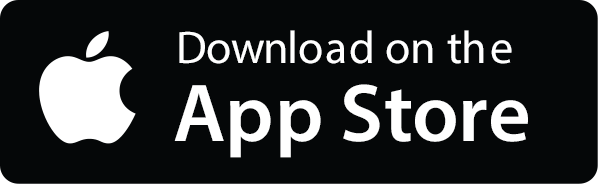
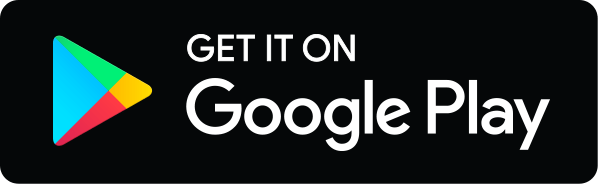
