Abstract
Myofascial pain is thought to occur by peripheral and central mechanisms. Peripheral factors include trauma, dysregulated deep-tissue microcirculation, and altered muscular metabolism and mitochondrial function. Supraspinal mechanisms include decreased modulatory activity, hippocampal suppression, and possibly impaired stress responses. Myogenic pain has been reported to contribute to up to 50% of temporomandibular joint disorders. Myofascial pain is characterized by the presence of loci of hypersensitivity within tender, taut, palpable bands of muscle called trigger points (TP).
There is increased paraspinal muscle tone in patients suffering from chronic LBP, and muscle spasm may be superimposed on primary injuries such as acute disc herniation. Individuals with nonspecific neck pain often manifest neck muscle contractions, altered patterns of muscle activation, and elevated levels of myoelectric activity.
True muscle cramps are painful involuntary skeletal muscle contractions associated with electrical activity. True muscle cramps, by definition, occur in the absence of fluid or electrolyte imbalance and have diverse etiologies. Seventy percent of patients with tension headaches suffer from muscle tightness and tenderness, with the percentage even higher in individuals suffering episodic headaches.
Studies showed TCAs to be effective in reducing the frequency and intensity of TTHs and facial pain/TMD. Gabapentin and pregabalin are first-line agents for the treatment of neuropathic pain but have also demonstrated some effectiveness in conditions characterized by muscle pathology. Studies showed varying effectiveness of muscle relaxants compared to other medications. Studies have shown conflicting results regarding the use of benzodiazepines in TMD and TTH. The evidence for their effectiveness in muscle spasm is moderate, and their adverse effect profile and inferiority compared to traditional muscle relaxants preclude their routine use.
NSAIDs are considered to be a first-line treatment for acute TTH, though persistent use may lead to rebound headaches. In TMD, NSAIDs can be beneficial although the evidence is limited by the poor quality of the studies. Controlled studies showed botulinum toxin to be effective in myofascial pain.
Opioids may provide short-term benefit for certain conditions characterized by myofascial pain, but they have not been shown to definitively provide long-term benefit, improvement in function, or greater benefit when compared to nonopioid therapy. The efficacy of topical treatments in myofascial pain has not been consistently shown.
Keywords
low back pain, myofascial pain, myofascial pain syndrome, muscle cramps, neck pain, temporomandibular disorder, tension headache
Myofascial pain disorders are a heterogeneous group of clinical entities that share features that originate from soft tissue with resultant regional symptomatology. Common examples of myofascial pain disorders include episodic tension-type headache (TTH), myofascial pain syndrome (MPS), temporomandibular disorder (TMD), muscle cramps, and nonspecific low back and neck pain.
Mechanisms of Muscle Pain
Muscle pain is thought to occur by two main mechanisms: peripheral and central. Peripheral factors include trauma, dysregulated deep-tissue microcirculation, and altered muscular metabolism and mitochondrial function. Mechanical, thermal, or chemical stimulation can lead to activation of intramuscular group III and group IV nociceptors, which in turn give rise to an inflammatory cascade mediated by immune cells, leading to further recruitment of inflammatory cells and propagation of local inflammation and sensitization. Pain transmission occurs along Aδ and C-fibers into the inner lamina of the spinal cord where complex changes occur, leading to sensitization and chronic pain.
Continuous nociceptive input via these pathways can lead to central sensitization of higher-order neurons, resulting in enhanced sensitivity to painful stimuli via excitatory glutamate- and aspartate-related neurotransmitter release (hyperalgesia), reduced thresholds to nonpainful stimuli (allodynia), and increased receptive fields, causing referred pain. Increased expression of the excitatory glutamate- and aspartate-related neurotransmitters and their receptors in the spinal cord is well known to play a role in the development of mechanical hyperalgesia in noninflammatory muscle pain.
Supraspinal mechanisms can also contribute to chronic muscular pain states. These include decreased modulatory activity, hippocampal suppression, and possibly impaired stress responses. Once central sensitization occurs, pain becomes autonomous from sensory input from the affected muscle(s).
Painful Conditions with Myofascial Involvement
Tension-Type Headache
The International Headache Society classifies TTHs as infrequent episodic (<12 days/year), frequent episodic (12 to fewer than 180 days/year), and chronic (≥180 days/year). Pathophysiologic mechanisms responsible for TTH can be divided into peripheral and central causes. Peripheral mechanisms are demonstrated by increased tenderness of pericranial myofascial tissue compared to a healthy population, as well as increased electromyographic (EMG) and algometric pressure recordings. Continuous nociceptive input can lead to central sensitization, thereby converting episodic TTH into chronic headaches. Patients with frequent TTH often demonstrate nociceptive hypersensitivity to various stimuli at both cephalic and extracephalic sites. Seventy percent of patients with tension headaches suffer from muscle tightness and tenderness, indicative of peripheral pain mechanisms, with the percentage even higher in individuals suffering episodic headaches. Other studies support roles for increased nitric oxide production, N-methyl- d -aspartate (NMDA) receptor activation, and neurogenic inflammation of the trigeminovascular system. Central pain processing appears to be relatively normal in patients with infrequent episodic headaches where peripheral pain mechanisms probably play a larger role.
Temporomandibular Disorder
TMD is a broad term used to describe conditions arising in the jaw joint, muscles of mastication, and associated craniofacial structures. These conditions most commonly include pain, dysfunction, arthritis, and internal derangement. The International Headache Society designates TMD as a subtype of secondary headaches, and the American Academy of Orofacial Pain subclassifies it into articular and masticatory muscle disorders. Many patients also have mixed arthrogenous and myogenous TMD. Myogenous pain has been reported to contribute to up to 50% of temporomandibular joint disorders. TMD is more prevalent in females than males, and mostly affects adults aged 20–50 years. Young patients may be more likely to suffer from myogenous TMD. TMD often coexists with other functional pain syndromes. According to a recent multicenter cohort study, patients with preexisting low back pain (LBP) have a 50% higher, and those with genital pain have a 75% higher incidence of TMD than those without these conditions. EMG recordings have demonstrated altered muscular contraction, as well as increased muscular tone in patients with TMD. Not surprisingly, EMG biofeedback has shown efficacy in TMD. Other evidence suggests that small muscles, such as those involved in mastication, may be more prone to hyperalgesia than larger muscles.
Myofascial Pain Syndrome
Despite the lack of specific objective criteria for the diagnosis of MPS, the work of Travell and Simons characterizes MPS by the presence of loci of hypersensitivity within a tender, taut, palpable band of muscle called a trigger point (TP). TPs are characterized by referred pain on palpation and elicitation of a local twitch response (LTR) with application of mechanical pressure; yet, in spite of these conventionally accepted criteria, studies have shown weak interrater reliability in the identification of TPs.
TPs can be classified into active TPs or latent TPs. Active TPs are described as pain in a motor locus associated with spontaneous electrical activity, whereas the more common latent TPs do not cause spontaneous pain, but can be triggered by factors such as mechanical or emotional stressors, dysfunctional postures, changes in weather, and either excessive immobility or the exaggerated use of muscles. It has been suggested that a positive feedback cycle involving disproportionate acetylcholine release, sarcomere shortening, and increased concentrations of sensitizing substances leads to the formation of a TP circuit, which upon connection with other spinal dorsal horn neuronal pathways, activates latent TPs to become an active TP. Some studies have shown that latent TPs are present in the shoulder-girdle muscles of half of asymptomatic young adults and in 5%–45% of lumbogluteal muscles. Other hypotheses suggest that hyperactive muscle spindles and end plates, focal dystonia, and/or psychological morbidity may play a role in the formation of TPs.
Low Back Pain
LBP is a significant public health problem with a lifetime prevalence rate ranging between 50% and 80%. Spine structures serve many functions, including protecting the spinal cord, maintaining posture and truncal stability, and acting as a steadying force for movement of the extremities. Skeletal and ligamentous structures serve as a protective foundation from which attached muscles provide functional motor control, flexibility, and movement coordination. Lumbar muscle function is well known to play an important role in LBP. Correct neuromuscular control and lumbar muscle proprioceptive feedback is essential in preventing LBP and sustaining postural stability. Weakness in the core muscles (lumbo-pelvic-hip complex), unbalanced gait mechanics, or dysfunctional muscular proprioception can lead to tears, strains, sprains, or spasm within the paraspinal musculature. Multiple studies show EMG evidence of increased paraspinal muscle tone in patients suffering from chronic LBP, and muscle spasm may be superimposed on primary injuries such as acute disc herniation. However, attributing LBP to solely myofascial pathology requires excluding other causes. Among the three layers of lumbar paraspinal musculature, only the most superficial is palpable. Additional support for muscular dysfunction as the cause of LBP comes from controlled trials demonstrating efficacy for botulinum toxin and a variety of muscle relaxants, as well as the proven effectiveness of neuromuscular reeducation and lumbar stabilization.
Neck Pain
Neck pain is a common cause of disability, with an annual prevalence rate of about 37%. Among the various causes, myofascial pain comprises a significant percentage of cases, both as a primary and a secondary etiology. Individuals with nonspecific neck pain often manifest delays in the onset of neck muscle contractions, altered patterns of muscle activation, and elevated levels of myoelectric activity. Similar to LBP, posture, body mechanics, sleep patterns, nutrition, psychological stress, and environmental factors play an important role. Cervical dystonia, torticollis, acute whiplash injury, and other etiologies of neck pain may be masked or difficult to distinguish from myofascial pain. Neck pain can also present as referred pain from masticatory muscles, and primary headache conditions such as migraine and occipital neuralgia headaches. Active myofascial TPs in the neck and parascapular region are often superimposed on cervical pathology, including acute cervical disc herniation. A clinical trial of patients with cervical radiculopathy and healthy controls found a significant difference in the distribution of active and latent myofascial TPs. No active myofascial TPs were noted in the control group, while latent myofascial TPs in the upper trapezius muscles were found to be increased in patients with cervical radiculopathy.
Muscle Cramps
True muscle cramps are painful involuntary skeletal muscle contractions associated with electrical activity. EMG studies show fast rates of repetitive firing in motor units of affected muscles. True muscle cramps, which by definition occur in the absence of fluid or electrolyte imbalance, have diverse etiologies. They are more commonly found in patients with well-developed muscles, in the third trimester of pregnancy, and in metabolic disorders such as cirrhosis and renal disease. Other causes of muscle cramps include medications, lower motor neuron disease, hypothyroidism, and hereditary disorders. The precise origin of cramps is the subject of debate, but there is evidence that abnormal discharges come from both central origins and peripheral motor neurons. Arguments in favor of a peripheral origin include the variable EMG morphology of fasciculations, the fact that cramps can be induced by repetitive peripheral nerve stimulation, and their high-frequency discharge rates (>150 Hz). Painful cramps can often be terminated by stretching the cramped muscle.
Treatment
Tricyclic Antidepressants
Tricyclic antidepressants (TCAs)—amitriptyline, nortriptyline, desipramine, and imipramine—provide analgesia independent of their antidepressive effects by multiple mechanisms, which include norepinephrine (NE) and serotonin reuptake inhibition in inhibitory descending pathways ( Table 53.1 ). Among the two, serotonergic reuptake inhibition may be less important in analgesia, although combined serotonin–NE reuptake inhibitors are generally better analgesics than selective NE reuptake inhibitors. The former point may explain the comparative decreased efficacy of selective serotonin reuptake inhibitors (SSRIs). Other active mechanisms include blockade of peripheral neural sodium channels, muscarinic and nicotinic acetylcholine receptors, alpha adrenergic receptors, NMDA receptors, dopamine receptors, and decreased substance P release.
Drug | Serotonin | Norepinephrine | Dopamine | Sedative | Antimuscarinic |
---|---|---|---|---|---|
Amitriptyline | +++ | + | — | +++ | +++ |
Nortriptyline | +++ | ++ | — | ++ | ++ |
Desipramine | — | +++ | — | + | + |
Imipramine | +++ | ++ | — | ++ | ++ |
Multiple studies have shown TCAs to be effective in reducing the frequency and intensity of TTHs and facial pain/TMD. The typical doses of amitriptyline in these studies ranged between 20 and 100 mg, which is considerably lower than that required to treat depression. Yet, even at these low doses, their use is limited secondary to myriad side effects, which include dry mouth, constipation, fluid retention, weight gain, difficulty concentrating, and cardiotoxicity.
Multiple systematic reviews have concluded TCAs to be effective at reducing the frequency and intensity of TTH ( Table 53.2 ). Of note, a double-blind, placebo-controlled, three-way crossover study in TTH found significant reductions in scalp tenderness and headache intensity with intermediate doses of amitriptyline (75 mg/day) compared with the serotonin-specific reuptake inhibitor citalopram and placebo. For TMD, the evidence appears more modest, with two randomized controlled trials (RCTs) demonstrating efficacy for amitriptyline and a sulfur-containing analog of amitriptyline, dothiepin. One double-blind, RCT assessing the efficacy of dothiepin in chronic atypical facial pain and arthromyalgia found 71% of the 93 patients in the treatment group became pain-free after 9 weeks versus 47% in the placebo group. Sixty-eight (81%) of the 84 patients who elected to continue treatment with dothiepin were pain-free at their 1-year follow-up. A subsequent study found significantly reduced pain intensity in patients with chronic facial pain who received amitriptyline compared with placebo. No dose-response relationship measuring analgesia between low-dose (≤30 mg) and high-dose (≤150 mg) amitriptyline was deemed significant, suggesting either a low ceiling effect or a high percentage of placebo responders.
Drug | Trade Name | Mechanism of Action | Typical Dose Range, mg/day | Pharmacology | Evidence for Efficacy | Common Adverse Side Effects |
---|---|---|---|---|---|---|
Amitriptyline | Elavil, Endep | Inhibits NE and 5HT reuptake, muscarinic acetylcholine receptor antagonist, H1 receptor antagonist, α1 adrenergic receptor antagonist, blocks Na + channels | 10–150 in evening/bedtime dosing; starting dose 25–75 mg oral; starting dose in elderly 10–25 mg (oral) | Liver metabolism and urine excretion primarily and feces; half-life 10–26 h | Strong—tension headache Moderate—facial pain with myofascial component and TMD | Dry mouth, constipation, fluid retention, weight gain, difficulty concentrating, and cardiotoxicity |
Nortriptyline | Pamelor | Inhibits NE and 5HT reuptake, muscarinic acetylcholine receptor antagonist, blocks Na + channels | 25–150 in evening/bedtime dosing; starting dose 25–50 mg (oral) | Liver metabolism, urine excretion primarily and feces; half-life 18–44 h | Moderate—chronic tension-type headache | Drowsiness, dizziness, nausea, vomiting, insomnia, sweating, dry mouth, tachycardia, pruritus, weight gain, constipation |
Imipramine | Tofranil | Inhibits NE and 5HT reuptake, M2 muscarinic acetylcholine receptor antagonist, histamine H1 receptors antagonist, blocks Na + channels, enhances dopaminergic activity | 25–150, 0.2–3 mg/kg, starting dose 0.2–0.4 mg/kg (oral) | Liver metabolism, urine excretion primarily and bile/feces; half-life 11–25 h | Moderate—tension-type headache | Drowsiness, dizziness, nausea, vomiting, headache, insomnia, sweating, confusion, dry mouth, tachycardia, constipation |
Desipramine | Norpramin | Inhibits NE reuptake, muscarinic acetylcholine receptor antagonist, blocks Na + channels | 25–150; starting dose 25–75 mg daily (oral) | Liver metabolism, urine excretion primarily; half-life 12–27 h | Weak—tension-type headache | Drowsiness, dizziness, nausea, vomiting, blurry vision, diaphoresis, confusion, dry mouth, tachycardia, constipation |
Anticonvulsants and Calcium Channel Antagonists
Pregabalin and gabapentin, an analog of gamma-aminobutyric acid (GABA), exert their analgesic effects by acting on the α 2 -δ 1 subunit of cellular calcium channels and blocking neurotransmitter release. Their binding to calcium channels results in suppression of abnormal neuronal discharges and an increased threshold for nerve activation. Although generally well tolerated, the most common side effects of gabapentin and pregabalin include dizziness, sedation, lightheadedness, somnolence, and weight gain.
Gabapentin and pregabalin are first-line agents for the treatment of neuropathic pain, but have also demonstrated some effectiveness in conditions characterized by muscle pathology ( Table 53.3 ). A randomized, placebo-controlled study conducted in 133 patients with chronic daily headache, which can involve increased muscle tone, demonstrated a modest improvement in the severity and frequency of headaches in gabapentin-treated patients compared to controls. Another recent prospective open-label study performed in patients with myofascial facial pain found that using a two-tiered approach utilizing TCAs and then gabapentin in nonresponders resulted in 54% of patients who obtained a ≥50% decrease in pain scores. This and other studies have led some experts to recommend gabapentin as a first-line treatment for headache prophylaxis. Placebo-controlled studies have also shown benefit for gabapentin in reducing spasticity in patients with multiple sclerosis and spinal cord injury (SCI), and for chronic masticatory myalgia.
Drug | Trade Name | Mechanism of Action | Typical Dose Range, mg/day | Pharmacology | Evidence for Efficacy | Common Adverse Side Effects |
---|---|---|---|---|---|---|
Gabapentin | Neurontin | Binds α 2 -δ 1 subunit of calcium channels blocking neurotransmitter release | 300–3600 in TID dosing; starting dose 100 daily or TID (oral) | Urine excretion; half-life 5–7 h | Strong—neuropathic pain Moderate—spasticity in patients with multiple sclerosis and spinal cord injury, fibromyalgia Weak—chronic daily headache, myofascial pain, low back pain, muscle cramps | Dizziness, sedation, lightheadedness, somnolence, nausea, vomiting, weight gain |
Pregabalin | Lyrica | Binds α 2 -δ 1 subunit of calcium channels blocking neurotransmitter release | 50–450 in BID or TID dosing; starting 50 mg BID (oral) | Negligible metabolism; urine excretion (90%); half-life 6.3 h | Strong—neuropathic pain, fibromyalgia | Dizziness, somnolence, ataxia, weight gain, peripheral edema, headache, dry mouth, blurred vision |
Weaker evidence supports use of gabapentinoids for other myofascial pain disorders. A retrospective review of gabapentin treatment in patients with neuropathic pain, myofascial pain, and chronic LBP found significant decreases in pain scores in both the neuropathic pain and myofascial groups, but not for LBP. In an open-label study evaluating gabapentin in patients with muscle cramps associated with a variety of different medical conditions, Serrao et al. noted a significant reduction in muscle cramps at the first 2-week follow-up visit after gabapentin treatment. Cramps had resolved in all patients by 3 months, lasting for the 6-month treatment period.
Other membrane stabilizers have been studied in muscle pain. Both placebo-controlled and open-label studies evaluating sodium valproate, an anticonvulsant that acts via a variety of mechanisms including the blockade of T-type calcium and sodium channels, and facilitation of GABA, have shown benefit in TTH and chronic daily headaches. An earlier randomized, placebo-controlled trial also found phenytoin pretreatment to be an effective means of reducing succinylcholine-induced postoperative myalgias.
Skeletal Muscle Relaxants
Skeletal muscle relaxants such as cyclobenzaprine (Flexeril), chlorzoxazone (Paraflex), carisoprodol (Soma), methocarbamol (Robaxin, Robaxisal), tizanidine (Zanaflex), and baclofen (Lioresal) are believed to exert their mechanism of action primarily within the brain and, in some cases, spinal motor neurons. Cyclobenzaprine, structurally related to first-generation TCAs, inhibits the reuptake of NE in the locus coeruleus and inhibits descending serotonergic pathways in the spinal cord. The latter effect may have an inhibitory effect on alpha motor neurons in the spinal cord, resulting in decreased firing and a reduction in monosynaptic and polysynaptic spinal reflexes. Tizanidine acts as a weak agonist at alpha-2 adrenergic receptors, and enhances presynaptic inhibition at spinal motor neurons. Carisoprodol, a precursor of the sedative-hypnotic meprobamate, is believed to produce muscle relaxation by blocking interneuronal activity in the descending reticular formation and spinal cord. Baclofen activates GABA-B receptors in the brain and reduces the release of excitatory neurotransmitters in both the brain and spinal cord. Baclofen also acts by inhibiting the release of substance P in the spinal cord. In addition to muscle spasm, the muscle relaxants dantrolene, baclofen, and tizanidine have been shown to be effective for spasticity.
Numerous studies conducted over many years have evaluated various skeletal muscle relaxants in conditions associated with muscle pain ( Table 53.4 ). Three separate randomized trials evaluating cyclobenzaprine in patients with cervical and lumbar spinal muscle spasm demonstrated efficacy in short-term follow-up. In two of these studies, cyclobenzaprine was superior to diazepam. A meta-analysis review found that cyclobenzaprine is more effective than placebo for LBP associated with muscle spasm, especially in the first 4 days of treatment. However, a recent randomized study found that adding cyclobenzaprine or oxycodone/acetaminophen in emergency department patients presenting with acute LBP did not improve function 1 week after discharge. Another randomized, placebo-controlled trial found cyclobenzaprine to be more effective than both placebo and clonazepam in patients with TMD. Of note, two randomized, controlled trials that evaluated dose-response in patients with acute muscle spasm found that 2.5 mg three times per day of cyclobenzaprine was not better than placebo, while 5 mg three times daily was equivalent to 10 mg three times per day.
Drug | Trade Name | Mechanism of Action | Typical Dose Range, mg/day | Pharmacology | Evidence for Efficacy | Common Adverse Side Effects |
---|---|---|---|---|---|---|
Cyclobenzaprine | Flexeril | Exact mechanism unknown, likely primary action on brain stem (central-acting) | 5–30 in divided doses; starting 5 mg daily (oral) | Liver metabolism and urine excretion primarily; half-life 18 h | Strong—cervical and lumbar spinal pain, muscle spasm Moderate—TMD with myofascial pain | Dry mouth, drowsiness, headache, diarrhea, constipation, dizziness, nausea, confusion |
Chlorzoxazone | Paraflex, Parafon Forte | Exact mechanism unknown, likely inhibits polysynaptic reflex pathways in spinal cord (central-acting) | 750–3000; starting 250 mg TID (oral) | Liver metabolism and urine excretion; half-life 1.1 h | Moderate—acute musculoskeletal pain, back pain, acute lumbosacral muscle strain | Drowsiness, dizziness, headache, lightheadedness, hepatotoxicity, GI upset, malaise, paradoxical CNS stimulation |
Carisoprodol | Soma | Exact mechanism unknown, likely interneuronal inhibition in spinal cord and descending reticular activating system (central-acting) | 1050–1400; starting 250–350 mg QID (oral) | Liver metabolism and urine excretion; half-life 2.4 h | Moderate—acute musculoskeletal pain, not for spasticity Weak—TMD | Drowsiness, dizziness, headache, ataxia, insomnia, confusion, tremor, irritability |
Methocarbamol | Robaxin | Exact mechanism unknown (central-acting) | 3000–8000; starting 750 mg Q6H (oral) | Liver metabolism and urine excretion; half-life 1–2 h | Moderate—nocturnal leg cramps, acute muscle spasm | Dizziness, drowsiness, lightheadedness, nausea, rash, headache, somnolence, hypotension |
Tizanidine | Zanaflex, Sirdalud | Binds to α2-adrenergic receptors, reducing presynaptic neurotransmitter release (central-acting) | 2–36; starting 2 mg (oral) | Liver metabolism and urine excretion (60%) and feces (20%); half-life 2.5 h | Moderate—spasticity, paravertebral muscle spasm Weak—TTH | Dry mouth, somnolence, asthenia, headache, dizziness, hallucinations, hypotension, constipation |
Baclofen | Kemstro, Lioresal | Binds to GABA-B receptors, inhibiting neurotransmitter release (central-acting) | 15–80; starting 5 mg TID (oral) | Liver metabolism (15%) and urine excretion (70%–80%) and feces; half-life 5.5 h | Strong—spasticity of spinal cord origin Moderate—cervical dystonia, upper motor neuron disease, stiff-person syndrome, acute back pain | Drowsiness, nausea, weakness, somnolence, dizziness, confusion, ataxia, constipation, headache, hypotension, weight gain |
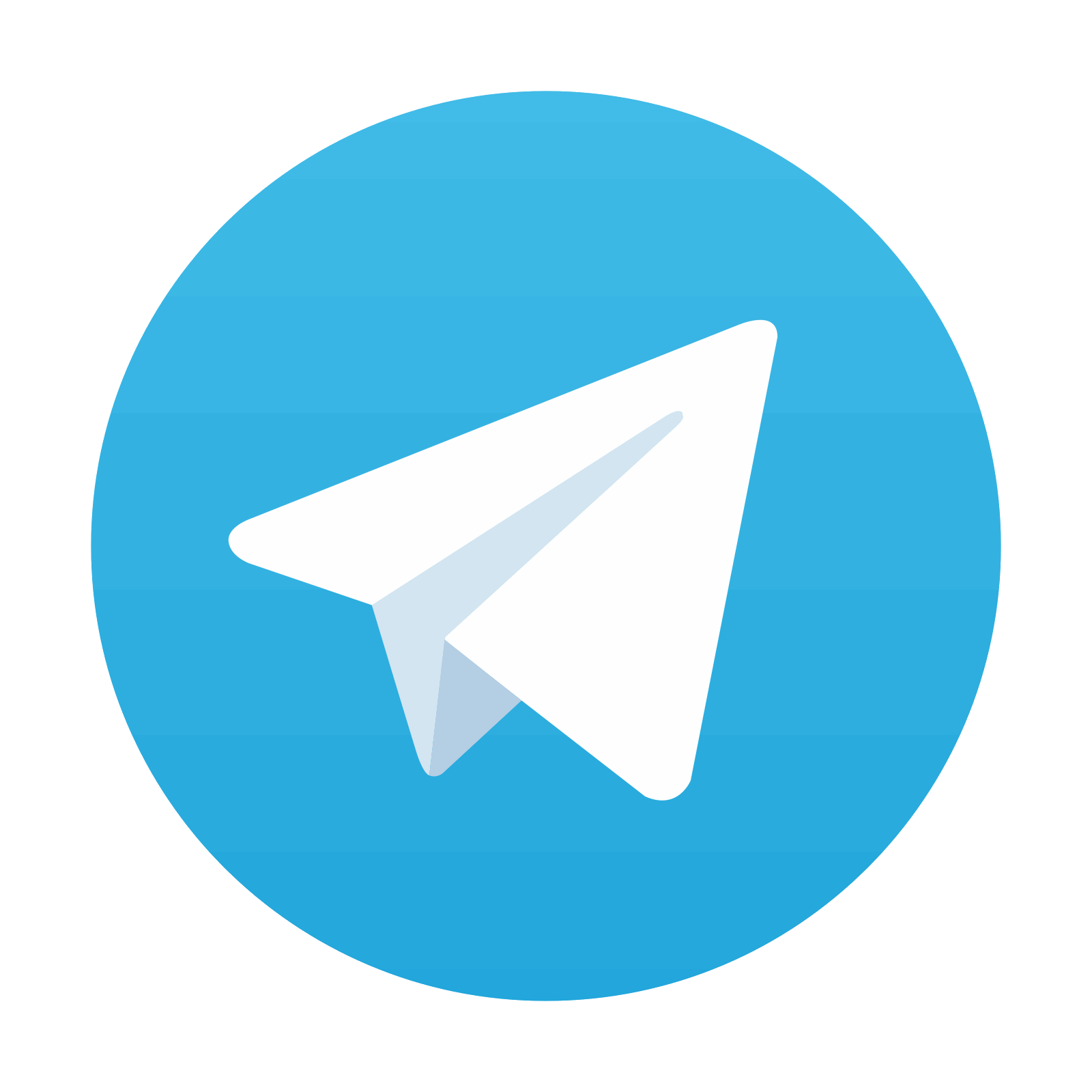
Stay updated, free articles. Join our Telegram channel

Full access? Get Clinical Tree
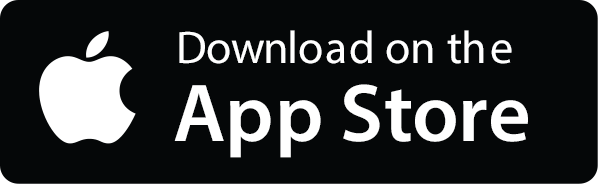
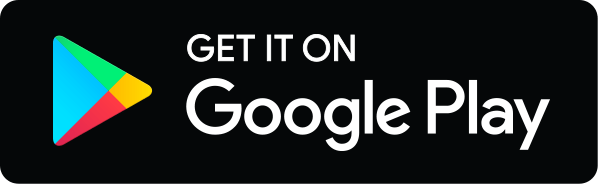
