Patients
n
Diagnostic criteria
Prevalence (%)
Shi et al. [3]
Pancreatic surgery
65
Fasting blood glucose ≥126 mg/dl (7 mmol/l)
Or
Random blood glucose ≥200 mg/dl (11.1 mmol/l)
63
Chen et al. [8]
Hip fracture
1257
Fasting plasma glucose level ≥126 mg/dl (7 mmol/l)
48a
Richards et al. [9]
Orthopaedic injuries
790
Random glucose value ≥200 mg/dl (11.1 mmol/l)
37
Shi et al. [3]
Biliary tract or liver surgery (excluding cholecystectomy)
48
Fasting blood glucose ≥126 mg/dl (7 mmol/l)
Or
Random blood glucose ≥200 mg/dl (11.1 mmol/l)
32
Shi et al. [3]
Cholecystectomy
593
Fasting blood glucose ≥126 mg/dl (7 mmol/l)
Or
Random blood glucose ≥200 mg/dl (11.1 mmol/l)
21
Richards et al. [10]
Operative orthopaedic injuries
171
Fasting glucose level ≥126 mg/dl (7 mmol/l)
Or
Random blood glucose level ≥200 mg/dl (11.1 mmol/l)
20a
Whitcomb et al. [11]
Mixed intensive care units (medical & surgical)
2713
Random blood glucose level ≥200 mg/dl (11.1 mmol/l)
18.5
Umpierrez et al. [12]
Community hospital (medical & surgical wards)
2030
Fasting glucose level ≥126 mg/dl (7 mmol/l)
Or
Random blood glucose level ≥200 mg/dl (11.1 mmol/l)
12
Doenst et al. [4]
Cardiac surgery
4701
Intraoperative peak glucose >360 mg/dl (20 mmol/l)
7
Kerby et al. [13]
Trauma level I patients
6852
Serum glucose ≥200 mg/dl (11.1 mmol/l)
4a
The main studies addressing the clinical significance of SIH have been performed on patients with acute coronary syndrome with or without coronary surgery and in those in critical care settings, and they have reported prevalences ranging from 40 to 80 % [14]. However, most of these studies have not excluded patients with known diabetes [15]. We were unable to find any studies reporting the prevalence of SIH in non-diabetic subjects undergoing cardiac surgery except for that of Doenst et al. [4], which reported a very low prevalence (7 %). However, in this study, the cut-off value used for defining SIH was far higher than the commonly used values, resulting also in a low prevalence of hyperglycaemia in patients with diabetes (15 %).
Chen et al. [8] reported a high prevalence (48 %) of SIH, indicating that age is an important factor to consider when evaluating whether a particular patient may develop this condition. The mean age of the patients in the study of Chen et al. [8] was 75 years, whereas the mean ages in the remainder of the studies listed in Table 9.1 were between 38 and 60 years. In particular, in the study of Kerby et al. [13] of patients at a level 1 trauma centre, the mean age of those without diabetes was 38 years, and this value likely explains, along with the exclusion of patients with previously undiagnosed diabetes, the low prevalence of SIH found in this population (4 %).
Other factors that affect the prevalence of SIH during the perioperative period include the use of hyperglycaemic medications such as corticosteroids, although this factor may have a lesser impact than previously envisaged [16], and the use of total parenteral nutrition with a high infusion rate of glucose (>4 mg/kg/min) [17].
9.2 Pathophysiology of Stress-Induced Hyperglycaemia
9.2.1 The Response to Stress and Stress-Induced Hyperglycaemia
The response to stress is mediated through activation of the hypothalamic paraventricular nucleus and locus ceruleus, which in turn activate the pituitary-adrenal axis and sympatho-adrenal system. The responses of these systems are graded with higher levels of stress (e.g., the APACHE score, Glasgow Coma Scale, type of surgery, etc.), leading to higher levels of cortisol and catecholamines [18, 19]. A high cortisol level increases hepatic glucose production, stimulates protein catabolism and increases circulating amino acid concentrations, providing precursors for gluconeogenesis. Epinephrine stimulates glucagon secretion and inhibits insulin release by pancreatic β-cells.
Activation of the pituitary-adrenal and sympatho-adrenal axes acts synergistically with proinflammatory cytokines (TNF-α, IL-1 and IL-6) to induce SIH via an increase in hepatic glucose production and the promotion of insulin resistance. Insulin resistance contributes to increased hepatic glucose production and decreased peripheral glucose uptake [2]. SIH seems to mainly depend on increased hepatic glucose production through the activation of hepatic gluconeogenesis. The TNF-α-mediated increase in glucagon synthesis is thought to play a main role in the increased hepatic gluconeogenesis.
9.2.2 Physiopathologic Consequences of Stress-Induced Hyperglycaemia
The increased plasma glucose level that occurs during stress alters the body’s homeostasis through multiple partially known mechanisms. The interpretation of how these changes affect homeostasis, either in a positive or negative manner, is the key to considering SIH either as a physiologic and protective adaptation [19] or as an exaggerated and deleterious response that must be reversed [14].
Some authors consider SIH as a means to improve the glucose gradient between the plasma and the intracellular medium in non-insulin-dependent tissues, especially the brain and the reticuloendothelial system, in situations of maldistributed microvascular flow [19]. In fact, a shift in membrane glucose transporters expression occurs during stress that favours the transport of glucose down a concentration gradient to brain cells and macrophages via up-regulation of GLUT-1 [20]. Conversely, expression of GLUT-4, which favours glucose transport to peripheral tissues, is down-regulated during stress [21].
Furthermore, it has been postulated that acute hyperglycaemia may protect against cell death following ischaemia by promoting antiapoptotic pathways and favouring angiogenesis [19]. Hence, endogenous cardioprotective mechanisms, although they have only been tested in animal models, have been demonstrated to become transiently activated by short-term hyperglycaemia through enhancement of vascular endothelial growth factor and nitric oxide synthase (eNOS) expression, NO formation, activation of cell survival signals, and decreased oxidative stress [22].
Conversely, increases in counterregulatory hormones and insulin resistance can be deleterious by increasing lipolysis and free fatty acid concentrations, which may be toxic to the ischaemic myocardium, leading to cardiac arrhythmias, sympathetic overactivity, increased blood pressure, oxidative stress and endothelial dysfunction [23]. In addition hyperglycaemia can impair immune system function by reducing phagocytic activity of macrophages, impairing chemotaxis of polymorphonuclear neutrophils, and increasing expression of adhesion molecules and free radical production in immune cells, which will ultimately increase the risk of infection [24].
In conclusion, the question of whether SIH is beneficial or detrimental cannot be definitively determined because evidence exists in support of both arguments. From a practical standpoint, it would be wise to permit a certain degree of hyperglycaemia, avoiding excessively high glucose levels that can affect immune function and induce osmotic diuresis, volume depletion and electrolyte loss.
9.3 Association Between Stress-Induced Hyperglycaemia and Outcomes and Benefits of Treatment in Surgical Patients
9.3.1 Stress-Induced Hyperglycaemia and Clinical Outcomes
Most observational and retrospective studies of hyperglycaemia conducted on patients during the perioperative period have demonstrated a strong association between SIH and poor clinical outcomes, including infections, cardiovascular morbidity, mortality, and overall complications.
9.3.1.1 Infections
The association of SIH, either preoperatively or postoperatively, with surgical site infections has been demonstrated across different surgical populations, including breast [25], colorectal [26], bariatric [27], hepatopancreaticobiliary [28], vascular [29], transplant [30] and orthopaedic patients [9], with adjusted odds ratios ranging from 1.4 to 9.4 [31]. Intraoperative SIH (glucose >200 mg/dl (11.1 mmol/l)) has also been associated with surgical site infections in liver transplantation patients [30]. This rather strong association is accompanied by evidence of a biological gradient and consistency, in support of hyperglycaemia as a causal factor for surgical site infections [31]. Moreover, there is also a strong biological plausibility for this association because hyperglycaemia impairs phagocyte function through different mechanisms, such as impairment of leukocyte chemotaxis [32] and reductions in superoxide formation [33], respiratory burst activity [34] and release of lysosomal enzymes [35].
9.3.1.2 Cardiovascular Morbidity
In patients with hip fracture, the incidence of acute myocardial infarction is almost doubled in those with SIH (12.5 % vs. 6.4 %) in the absence of diabetes [8]. In patients with SIH, the peak incidence of acute myocardial infarction parallels the peak in the blood glucose level. Moreover, SIH is the sole significant independent risk factor for the development of acute myocardial infarction. Hyperglycaemia increases platelet activation, thereby enhancing platelet-mediated thrombogenesis [37]. Hence, it is plausible that SIH increases the risk of acute coronary disease in at-risk patients. It must be noted, however, that hip fracture is a specific situation in which the risk of AMI is increased [38]. An association of SIH with cardiovascular events has also been demonstrated in other high-risk situations, such as cardiac surgery [4].
9.3.1.3 Mortality
SIH is clearly associated with mortality in critically ill patients with unstable angina, acute myocardial infarction, congestive heart failure, arrhythmia, ischaemic and haemorrhagic stroke, gastrointestinal bleeding, acute renal failure, pneumonia, pulmonary embolism, and sepsis [39], independent of the illness severity score and length of hospital stay. The mortality rate for each predefined glucose category of above 110 mg/dl is higher in subjects without diabetes compared with those with diabetes, highlighting the importance of SIH as a risk factor for these diseases. However, in this same study, the association between SIH and mortality was not found to be significant or was highly attenuated for most surgical procedures performed on patients admitted to intensive care units.
SIH has also been associated with mortality in non-critically ill surgical patients at a community hospital [12]. Again, the mortality rate was higher for patients with SIH without known diabetes.
In trauma patients [13], SIH has been associated with mortality in those who are non-diabetic (39.7 ± 18.1 years), also excluding those with undiagnosed diabetes, whereas no association between hyperglycaemia and mortality has been found in diabetic patients (mean age 57.5 ± 15.7).
Conversely, in a retrospective study of 1574 patients undergoing coronary artery bypass surgery, hyperglycaemia was not found to be associated with mortality after adjusting for diabetes status and the calculated preoperative mortality or mediastinitis risk scores [40].
Although most studies showing an association between SIH and mortality have adjusted for disease severity, a causal association between SIH and mortality has not been completely established. It can be argued that since the association between the glucose level and mortality depends on the presence or absence of previously diagnosed diabetes, it is not glucose per se that causes the increase in mortality because if hyperglycaemia was the cause, the mortality rates would be rather similar in patients with and without diabetes, if not worse in diabetic patients, who are usually older and have a worse health status. On the other hand, some authors have argued that this stronger association might reflect a less intensive treatment of hyperglycaemia in patients without diabetes [12]. However, studies of critically ill and sepsis patients have shown that the relationship between SIH and mortality is lost once the lactate level is taken into account [41, 42]. In a previously described study of trauma patients [13], lactate levels were found to be significantly higher in non-diabetic patients with SIH.
9.3.1.4 Other Outcomes
The modulating effect of hyperglycaemia on functional recovery in patients with ischaemic cerebral injury has been extensively studied [43]. In surgical patients, an association between intraoperative hyperglycaemia and neuropsychological (for glucose concentrations ≥129 mg/dl) and gross neurological (for glucose concentrations ≥152 mg/dl) recovery has been demonstrated in those undergoing cerebral aneurysm surgery [44]. However, it is difficult to discern from the data of this study whether a high level of glucose is a causal factor or is merely a marker of more extensive neurologic injury occurring during the procedure.
9.3.2 Benefits of Treatment of Stress-Induced Hyperglycaemia
Association does not indicate causality; therefore, before recommending a specific glucose-lowering strategy to surgical patients, the beneficial effects of this strategy must be assessed in randomized controlled trials.
The traditional view on glycaemic control in critically ill patients was that acute hyperglycaemia represented a normal, and perhaps beneficial, adaptive response that promoted cellular glucose uptake [45]. In fact, this view is still defended by some experts [19] and supported by some recent publications [46]. With this approach, the tolerant management of SIH (glucose <200 mg/dl) is recommended to avoid the adverse effects of hyperglycaemia and increased osmolarity on granulocyte function [45].
The Leuven trial, conducted in 2001 [47] on critically ill patients in a single centre intensive care unit with predominantly surgical patients, showed benefits of intensive insulin therapy (aiming for a glucose level of between 80 and 110 mg/dl) vs. a conventional approach (aiming for a glucose level of below 215 mg/dl) on mortality, mainly due to improvements in the rates of multiorgan failure with a proven septic focus and morbidity (bloodstream infections, acute renal failure, critical illness polyneuropathy and prolonged mechanical ventilation). However, these positive results have not been reproduced in subsequent trials [14]. Randomized controlled trials conducted after the Leuven trial have mainly evaluated the benefits and risks of strict blood glucose control (between 80 and 110 mg/dl) vs. somewhat more permissive blood glucose control (less than 180 mg/dl (10 mmol/l)) in a mixed surgical and medical population of patients showing no benefits of the intensive strategy [48] or even an increase in 90-day mortality [49]. Interestingly, another randomized, although not blinded, study [50] comparing the intensive approach vs. an even more permissive approach (blood glucose level of between 180 mg/dl and 200 mg/dl (10–11.1 mmol/l)) [10] has also found no benefit of the intensive strategy on either 28-day mortality or morbidity. Furthermore, all of these studies have uniformly shown robust increases in hypoglycaemic events in the intensive arm [51], a fact that might be important in view of the association found between hypoglycaemic events and mortality in critically ill patients [52].
Notably, all of these studies were performed on a mixed population of patients with and without previously diagnosed diabetes, with the proportion of non-diabetic patients ranging from 70 to 87 % [14]. This approach to a complex problem might cause confusion because the treatment effects cannot be assumed to be the same in both groups of patients. Patients with already established diabetes may harbour clinical or subclinical vascular damage that can affect the outcome of acute disease and the response to insulin treatment. Additionally, a certain degree of hyperglycaemia may reflect a substantially different degree of stress, depending on the glycaemic status before the onset of the acute disease. In fact, it can be inferred that patients with diabetes have a higher level of glycaemia than non-diabetic patients experiencing the same level of stress, making it difficult to interpret studies reporting that SIH is particularly detrimental in patients without previously established diabetes [12, 39].
9.4 Treatment of Stress-Induced Hyperglycaemia
9.4.1 Preoperative Control
Worldwide epidemiological data indicate that 30–50 % of the population has impaired glycaemic control (diabetes or prediabetes), and up to 30 % of individuals are unaware of their status [53, 54]. Thus, the glucose levels of patients undergoing preoperative evaluation must be checked because unrecognized impaired glycaemia may lead to hyperglycaemia during the intraoperative or postoperative period. Patients especially predisposed to diabetes should be carefully evaluated prior to surgery.
Nevertheless, there is no consensus among scientific societies with regard to the criteria to use to screen for diabetes or prediabetes in asymptomatic patients. The American Diabetes Association recommends screening for diabetes or prediabetes in all adults aged 45 years and at even younger ages in overweight patients with other risk factors such as physical inactivity, a first-degree relative with diabetes, a high-risk ethnicity (African-American, Pacific Islander, or Latino), delivery of a baby weighing >9 lb for females, or the presence of polycystic ovarian syndrome, dyslipidaemia, a history of impaired glycaemia or glycosylated haemoglobin (HbA1c), the presence of hypertension with other clinical conditions associated with insulin resistance, such as obesity or acanthosis, or a history of cardiovascular disease [55]. The US Preventive Services Task Force only recommends screening for diabetes in adults with a blood pressure of >135/80 mmHg [56]. The Endocrine Society Guidelines for the management of hyperglycaemia in hospitalized patients in non-critical care settings recommends laboratory blood glucose testing in all patients upon admission and bedside glucose monitoring with a point-of-care glucose metre in all non-diabetic patients with a blood glucose level of >140 mg/dl (7.8 mmol/l) for at least 24–48 h. The same recommendation applies to patients receiving therapies that promote hyperglycaemia, such as corticosteroids, octreotide and enteral or parenteral nutrition [57]. However, current perioperative recommendations do not advocate testing for diabetes mellitus prior to surgery, except for cardiac surgery [58].
Patients diagnosed with diabetes during the preoperative period must be evaluated by their general practitioner or diabetologist before surgery and treated during the perioperative period, as discussed in a specific chapter of this book on the perioperative management of patients with diabetes.
9.4.2 Intraoperative Control
There is an absence of prospectively controlled studies specifically comparing patients with and without SHI; thus, no consensus has been reached on the intraoperative management of this condition in non-critical patients because no studies have distinguished these patients from previously diabetic patients. Regular monitoring of blood glucose and intravenous insulin therapy with variable rate infusion (VRIII) are the best methods for controlling hyperglycaemia in non-critically ill surgical patients. These methods result in added responsibilities and tasks for anaesthesiologists and nurses during surgical procedures. Guidelines for perioperative glycaemic control in all types of patients recommend defining and implementing a standard protocol for glucose control that must be implemented only after intensive training of all relevant staff [55, 59, 60].
It must be considered that multiple transient factors, such as pain, the type of surgery and the use of some drugs and anaesthetic agents, could modify glycaemia [61]. This issue is addressed in the chapter on the perioperative management of diabetic patients.
9.4.3 Glucose Monitoring
Monitoring glycaemia during anaesthesia is the only method available for diagnosing SIH during surgery. Ideally, blood glucose monitoring should be started while the patient is awaiting elective surgery and repeated intraoperatively, although there are no recommendations on the frequency of glycaemic monitoring on the operative day if insulin therapy is not prescribed. When VRIII is used hourly, blood glucose monitoring is mandatory.
Glycaemia may be monitored using a point-of-care glucose metre, which tests for capillary blood glycaemia using a blood gas/glucose analyser, or in the laboratory by performing measurements in whole arterial or venous blood, which is the recommended method by some scientific societies for critically patients and can be used if an arterial line is placed intraoperatively [62]. Levels measured in capillary samples using glucometers may differ by up to 20 % from those reported in laboratory results, and such a discrepancy is acceptable for hyperglycaemia, but not for hypoglycaemia. The accuracy of blood glucose monitoring is affected by various potential patient conditions, such as anaemia, oedema, skin hypoperfusion or concomitant use of drugs, such as dopamine, mannitol or noradrenaline. In these circumstances, the laboratory blood glucose level must be checked regularly.
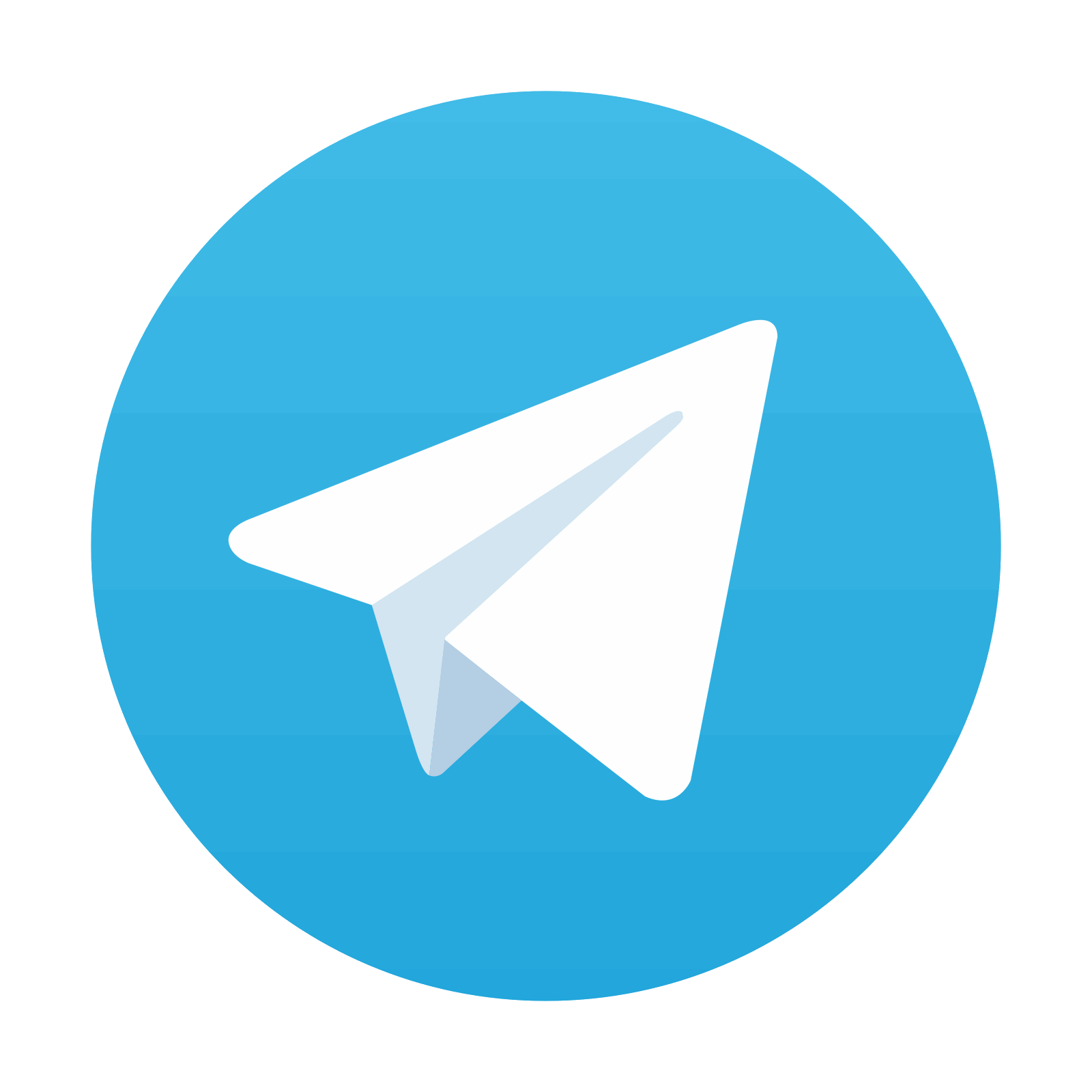
Stay updated, free articles. Join our Telegram channel

Full access? Get Clinical Tree
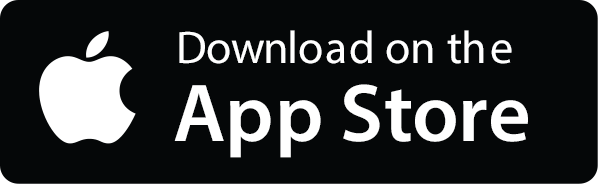
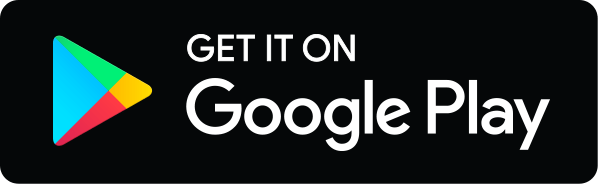