The Decade of Pain Control and Research (DPCR) legislation passed by the 106th Congress helped promulgate an extraordinary number of scientific inquiries into the mechanisms and treatment of pain. In the decade before Senator Hatch introduced the Bill, a PubMed search of the term “pathophysiology of pain” retrieved 53 articles. In the DPCR, the same search yielded 32,283 articles. In the ten years since the publication of the second edition of this textbook in 2004, nearly 48,000 articles have been published. The accelerated rate of publications has begun to slow; but it was hoped that such research growth would continue for three reasons: (1) For humane considerations, we simply need more effective and safer medications for better pain management. (2) The cost of pain to society is colossal–nearly $55 billion per year by one estimate. (3) Improved scientific and technological knowledge about pain pathways drives pain drug discovery research and development to meet demand.1 However, by the end of the DPCR, many impediments to using evidence-based pain management methods still remained, and federal economic support was waning.2,3
Acute pain results from the complex convergence of many signals traveling up and down the neuraxis and serves to warn us of potential impending harm. For example, noxious stimuli activate nociceptors in the periphery. The signal travels centrally, carried by the axons of the primary sensory neurons, the dorsal root ganglia (DRG), which are relatively quiescent unless specifically stimulated by sensory input. The pain response can also harm rather than help the individual. Injured DRG may become hyperexcitable and display considerable spontaneous electrical activity. Such increased activity results from the expression of a dramatically different constellation of cell-specific molecules in injured cells compared with normal ones. Ultimately, the operation of complex neuronal circuits may be markedly altered. Chronic pain sensation can result from such injury.
Woolf describes a classification system that separates pain into two major divisions: adaptive and maladaptive. The former serves to protect the organism, but the latter can lead to pathological pain maintained as a disease state. Woolf’s framework then distinguishes four different types of pain: nociceptive, inflammatory, neuropathic, and functional (abnormal central processing). Investigators have delineated some, but far from all, of the many mechanisms operative in the generation of these types of pain and the etiologies that cause them.4
Considerable knowledge has accrued, which has given some insight into the mechanisms responsible for the development of acute and chronic pain. Understanding the changes that follow injury at a cellular and molecular level may help lead to new therapeutic interventions. This chapter highlights, rather than exhaustively chronicles, some of these findings. The peripheral sensitization changes seen in inflammatory pain are described first, then the central mechanisms of sensitization, followed by the role of neurotrophic factors, the effects on neuronal ionic channels, higher neural mechanisms, central pain, and genetics. The chapter concludes with some remarks.
Unmyelinated C or thinly myelinated Aδ afferent fibers convey nociceptive pain sensation. Minor irritation of tissue in a neuron’s receptive field also results in the release of inflammatory mediators, which is often accompanied by a reduction in the nociceptor threshold. Such a change, called peripheral sensitization, renders the nerve ending responsive to weak, normally nonpainful stimuli (allodynia). Stronger stimuli typically provoke exaggerated pain (hyperalgesia). Sensitization involves not only normal nociceptive fibers, but also the recruitment of so-called silent nociceptors, which are not usually sensitive to painful stimuli or inflammatory substrates such as prostaglandins or bradykinin.
Bradykinin can sensitize C and Aδ fibers to prostaglandins, protons, serotonin, heat, and mechanical stimuli.5–8 Because its algesic effect displays considerable tachyphylaxis, however, bradykinin alone cannot account for the hyperalgesia seen in inflammation.7 Bradykinin also appears to facilitate the production of prostaglandins.9 Similarly, prostaglandins sensitize nerve afferents to bradykinin action.10 Blockade of such sensitization accounts for the clinical efficacy of antiinflammatory drugs such as aspirin and cyclooxygenase-1 and -2 inhibitors.
Some light has been shed on cellular transduction mechanisms that may be involved in the development of this type of sensitization. For example, the afterhyperpolarization of primary afferent fibers decreases, which renders the cell more likely to fire repetitive action potentials in response to subsequent stimuli. The process appears to involve adenyl cyclase and the second messenger cyclic adenosine monophosphate (cAMP). The latter provokes the phosphorylation of potassium (K) channels, which is catalyzed by protein kinase A (PKA). Excitability increases as K conductance decreases. Some investigators have proposed a universal role for cAMP in producing sensitization in response to multiple physical and chemical noxious stimuli.11–13 Some evidence suggests the role of cAMP-PKA in decreasing the activation threshold and increasing the size and rate of activation of a tetrodotoxin-resistant sodium (Na) channel in nociceptive neurons, too.14 The decrease in inflammatory pain seen in mice that have a point mutation in a PKA subunit supports the importance of this cAMP-PKA mechanism.12
Phospholipase C induction by the activation of bradykinin or neurokinin (NK) receptors can ultimately lead to increased electrical activity of primary afferent nerve terminals. Intracellular calcium (Ca) increases, which may increase adenyl cyclase activity and cAMP levels. Phosphorylation of specific cation channels and their subsequent increased activity results.15
Primary afferent nerve fibers have many 5-HT2A receptors,16 the activation of which produces a G protein–mediated decrease in K currents.17,18 Hence, 5-HT2A receptor blockade can antagonize this component of inflammatory pain.17 Prostaglandins may also provoke a separate type of decrease in outward K current, mediated by cAMP-PKA.12
Inflammation leads to an upregulation of nitric oxide (NO) synthetase in DRG and other cells. Ultimately, neuropeptides may be released from nociceptive nerve terminals, which can produce inflammatory hyperalgesia.8 Whereas subcutaneous injection of NO produces pain,19 interruption of NO synthetase blocks the hyperalgesia.20 These findings oversimplify the role of NO in peripheral sensitization, however, because some data suggest an antinociceptive role for NO.8,21,22 Although NO generates cyclic guanosine monophosphate (cGMP) as an intracellular second messenger, the cyclic nucleotide does not seem to contribute to the sensitization of the nerve terminals.11,23 Recent data suggest that NO may exert its action on peripheral nerve terminals indirectly. Moreover, NO synthetase may be involved early in the inflammatory response, but NO itself does not participate in chronic inflammation.24 This indirect action, which may be mediated by its effect on blood vessels, coupled with its direct action on nerve terminals, has led some investigators to postulate a role for NO in triggering migraine headaches.25
Considerable evidence exists for the role of neurotrophic factors in the long-term development of neuropathic pain (see later discussion). Recent data have suggested that neurotrophins can also affect neuronal function in the short term. Although not studied directly in C-fiber terminals, changes in ionic current flow, intracellular Ca level, and protein phosphorylation have been shown to lead to altered neuronal excitability, neurotransmitter release, receptor distribution, and synaptic efficacy in other systems.8,26–30
The recruitment of previously mechanically silent nerve fibers that become sensitive to mechanical or thermal stimuli after exposure to inflammatory mediators such as bradykinin suggests another mechanism of peripheral sensitization.31,32 Investigators have proposed that primary afferent substance P–containing neurons are involved in developing hyperalgesia. The demonstration that prostaglandins can increase the number of bradykinin-responsive, substance P–containing fibers is consistent with this finding.33
Transient receptor potential (TRP) channels constitute a group of ligand-activated, nonselective cation channels. It has been known for some time that the transient receptor potential vanilloid 1 (TRPV1) receptor, a member of this family, plays a role in the transduction of noxious thermal and chemical stimuli such as acids and vanilloids (e.g., capsaicin) and inflammation-induced hyperalgesia.34–36 More recently, using a genetic knock-out mouse model, Vardanyan and colleagues demonstrated that TRPV1 receptors also play a role in opioid-induced hyperalgesia.37
In summary, multiple mechanisms modulate the functioning of primary afferent nerve terminals. The final common pathway appears to involve the increase in intracellular Ca and protein kinase levels. Both Ca and protein kinases exert a profound influence on the control of gene transcription (see later discussion).
Central sensitization refers to the plasticity displayed by central neural structures involved in pain perception. Some of these physiologic neuronal changes can occur within a few minutes. These may not be maintained once the stimulus is removed. Still other changes remain long after the noxious stimuli cease and may reflect irreversible processes. Two prominent features of peripheral tissue injury include pain and an exaggerated response to noxious stimuli, such as heat. However, the hallmark of clinical pain, mechanical allodynia, cannot easily be explained without invoking central neuronal plasticity. Central mechanisms must also be considered in describing referred pain.8
One clinical example of central sensitization is seen in amputees who exhibit phantom limb pain, which is similar to that experienced prior to the amputation. The pain can remain long after the inciting peripheral stimulus is removed. Surgical patients who have derived benefit from preemptive analgesia constitute another example. In these patients, the short- and long-term central neural structure changes that might lead to sensitization are blocked.
Considerable experimental data support the concept that mechanical allodynia results from the activation of Aβ nerve fibers, which normally subserve light touch or vibration, not pain. Some of the evidence is indirect but includes the following.8 Primary afferent nerve fiber injury leads to abnormal electrical excitability of both small and large fibers, including Aβ fibers. The latter exhibit exquisite sympathetic sensitivity after injury, and pharmacologic or surgical sympathectomy can ameliorate mechanical allodynia.38,39 High-threshold C or Aδ fibers do not seem responsible for the allodynia because the transduction sensitivity of these single fibers remains stable before and after injury.38,40 The time course of onset of allodynia correlates with the more rapid conduction velocity of the larger fibers.38 Blockade of only Aβ fibers extinguishes allodynia.41 Aβ fibers activate AMPA (DL-α–amino-2,3-dihydro-5-methyl-3-oxo-4-isoxazolepropanoic acid)/kainic acid receptors in the dorsal horn (DH) of the spinal cord. Such receptors have not been found on C fibers, and their specific antagonism reduces allodynia.42 The time course of the appearance of allodynia following nerve injury corresponds to that of the upregulation of AMPA receptors.8 Finally, without inflammation, normal touch does not lead to changes in gene expression.43 In summary, although some of the evidence is indirect, Aβ fibers appear to play a powerful role in the mediation of mechanical allodynia.
Reports have demonstrated secondary hyperalgesia to only mechanical stimuli. The investigators suggested that independent peripheral mechanisms accounted for the evoked pain and central sensitization.44 The involvement of Aβ fibers in mechanical allodynia, as noted earlier, also suggests that central, rather than peripheral, mechanisms must be operative.8
Data suggest that maintained pain can result from several specific processes that converge on the DH of the spinal cord. They include neuronal sensitization, reduction in inhibitory interneuron activity, and a modulation of descending pathway activity.8 Brief descriptions of each follow.
DH neuron changes resembling long-term potentiation and long-term depression can result from high-frequency stimulation of C fibers or low-frequency stimulation of Aδ fibers, respectively.45–47 Intense electrical or noxious stimulation of C fibers can promote wide-dynamic-range (WDR) neuron hyperexcitability in the DH. N-methyl-D-aspartate (NMDA) receptors and levels of intracellular Ca and protein kinases play a crucial role in this sensitization.45,48 Sensitization may involve two qualitatively different actions at synapses. At one type of synapse, transmission efficacy increases in a graded fashion. At the other, so-called silent synapses switch to become active.49,50
Sensitization can be demonstrated in DH neurons even after short periods of electrical or noxious stimulation. For example, repetitive electrical stimulation provokes increased excitability, lasting about an hour. Such action potential “wind-up” has been used to model “pain” at the cellular level. Wind-up refers to the slow, prolonged depolarization and ultimate burst of action potentials seen with stimulation.51 It can be demonstrated in only a few cells, which suggests a cell-specific mechanism of generation.48 During such hyperexcitability, several neuronal changes occur, with presumptive clinical correlates. First, subsequent stimuli evoke a longer and more intense period of action potential firing, which qualitatively resembles hyperalgesia. Second, receptive fields increase in size, which is consistent with secondary hyperalgesia. Finally, the threshold for firing action potentials is decreased and responses to Aβ fibers appear, which resembles allodynia.8
Temporal and spatial summation of fast and slow synaptic potentials and action potential wind-up can explain the period of DH neuronal hyperexcitability. Heterosynaptic facilitation also develops whereby the increased excitability derived from one synaptic input increases the response amplitude from a second, separate input.45
Most of the recent work on unraveling the molecular mechanisms of WDR neuron sensitization has focused on three classes of agents: excitatory amino acids, tachykinins, and calcitonin gene–related peptide (CGRP). Other compounds have been less well studied. These transmitters and neuromodulators affect DH neuron activity by directly increasing cation fluxes, impinging on intracellular transduction mechanisms, and modulating receptor and transmitter gene transcription. Synaptic transmission augmentation at NMDA receptors is the final common pathway.8,52–54
Because the central role of NMDA receptors in sensitization cannot be overemphasized, its following properties may lend some insight.8 Magnesium (Mg) blocks the ionic channel at rest but can be displaced by adequate depolarization.55,56 Ca readily permeates its ionic channel; hence its activation increases intracellular Ca levels.57 Increased intracellular Ca levels augment protein kinase activity.58 Phosphorylation antagonizes the Mg-channel blockade, which then can function at hyperpolarized levels.59 Bursts of action potentials can result from NMDA receptor activation, which greatly increases transmitter release at higher levels in the nervous system. Activation of presynaptic NMDA receptors causes increased release of excitatory amino acids and substance P.60 Hence, several mechanisms serve to amplify signals transmitted through NMDA receptors.
NMDA receptors consist of the subunit NR1 and at least one of the subunits NR2A-D. Recently, Qu and colleagues reported that intrathecal application of a selective NR2B-containing NMDA (NMDA-2B) receptor antagonist both alleviated neuropathic pain selectively, without affecting motor function, and inhibited the induction of long-term potentiation (LTP) in dorsal horn WDR neurons.61
AMPA, NK, metabotropic glutamate (mGlu), and CGRP receptors also play roles in the sensitization of DH neurons.8 Activation of AMPA receptors leads to increased intracellular Ca and depolarization. In other areas of the nervous system, AMPA and NMDA receptors reciprocally activate one another. AMPA receptors are ultimately phosphorylated over a time course of 10 to 15 minutes, which increases their responsiveness. Some investigators have proposed a role for them in maintaining long-term potentiation.62,63
Substance P and neurokinin A (NKA), which are released by stimulation of C fibers, exert their actions at neurokinin-1 (NK-1) and neurokinin-2 (NK-2) receptors, respectively.64 Activation of these receptors supplements DH neuron sensitization. Moreover, NK-1 receptors appear to strengthen the sensitizing actions mediated by NMDA receptors. Consequently, administering specific antagonists of both receptors can ameliorate certain painful states.65,66
Subtypes of mGlu receptors are coupled to phospholipase C, which suggests that their activation increases intracellular Ca levels. In the cerebral cortex, evidence exists that mGlu receptors augment the sensitizing actions of NMDA receptors.67,68 They also increase AMPA receptor excitability.69 Their appearance at high density in lamina II of the DH indirectly suggests a role for them in pain transmission. The action of CGRP in the spinal cord remains poorly understood, but investigators have suggested that it potentiates NMDA and NK-1 receptor opening.70
The preceding cell-surface receptor actions suggest that intracellular Ca levels, Ca influx, and protein kinase activation play an important intracellular role in DH neuron sensitization.8 Different intracellular stores of Ca may promote different events in the neurons. On balance, the increased levels of intracellular Ca generate sensitization, but suitable negative feedback mechanisms are activated to minimize the likelihood of cell death resulting from toxic intracellular levels of free Ca. Calcium levels induce protein kinase activity in the neuronal soma, which leads to phenotypic and ultimately genotypic changes. For example, under the influence of high intracellular Ca, protein kinase C phosphorylates NMDA receptors, which promotes long-term potentiation.58,71 Activation of nuclear transcription factors and gene transcription also occurs.72,73
Diminution of the inhibitory influences supplied by inhibitory interneurons results in increased WDR neuron excitability consistent with clinical hyperalgesia and mechanical allodynia.74 The loss of γ-aminobutyric acidergic (GABAergic) and glycinergic activity in the DH produces a state of neuronal hyperexcitability. Such inhibitory loss amplifies the excitability of WDR projections.75,76 As would be expected, pharmacologic antagonism of GABAergic and glycinergic receptors with bicuculline and strychnine produces a functional state resembling clinical allodynia. Conversely, the allodynia produced by injury is ameliorated by GABA agonist administration.77
Considerable research has addressed the possible mechanisms responsible for the reduction in inhibitory interneuron activity.8 If a decrease in GABAergic and glycinergic activity can mimic allodynia, does such a diminution actually cause chronic pain? Although the final verdict is not yet in, it appears that primary afferent fiber injury leads not only to NMDA receptor activation, with consequent sensitization of WDR neurons, but also to a huge outpouring of excitatory amino acid neurotransmitters at inhibitory interneuron NMDA synapses. Such release has been postulated to cause massive accumulation of intracellular Ca and NO, which ultimately leads to cell death.78,79 Other speculation attributes the decrease in inhibitory interneuron activity to loss of neurotrophic factors from injured primary afferent fibers.80 In this regard, the role of GABA attracts attention because primary afferent terminal degeneration provokes a biphasic response of GABAergic neurons, which suggests a trophic role for GABA itself in the reorganization of spinal networks.81
For some time, neuroscientists have envisaged a role for descending input from supraspinal pathways in controlling nociceptive signaling in the DH of the spinal cord.82 Signals transmitted to higher centers depend on tremendous afferent and efferent integration of information processing at lower centers.83 Investigators have examined the role of supraspinal structures in the development of secondary allodynia.84 Blocking allodynia with precisely localized microinjections of lidocaine, they concluded that brainstem pathways immediately adjacent to the raphe magnus contribute to the development of secondary allodynia.
Several neurotransmitter systems appear to be involved in the increases or decreases in descending facilitation or inhibition, respectively.8,85 Recent data suggest that augmentation of serotonergic systems contributes to DH neuron sensitization. Primary afferent nerve terminals, excitatory interneurons, and projection neurons exhibit multiple types of 5-HT receptors over their plasmalemmae. Evidence suggests that the 5-HT3 receptor subtype accounts for the facilitatory action of 5-HT on the evoked release of substance P–like immunoreactive materials. NO mediates the intracellular signal transduction by promoting an increase in cGMP production.86 Stimulation of the 5-HT1A subtype enhances K currents and suppresses Ca currents.87 These data have led to the suggestion that inhibition of inhibitory interneuron 5-HT1A receptors reinforces DH neuron sensitization and, therefore, allodynia.85
Recently, using a spinal nerve ligation (SNL) rat model of neuropathic pain, Wang and colleagues reported that the 5HT3 receptor antagonist ondansetron blocked SNL-induced pain.88
Descending dopaminergic systems also play a role in regulating spinal neuron excitability. Two families of dopamine receptors have been defined based on their properties. One includes dopaminergic receptors that are D1-like, which consists of D1 and D5, and another that are D2-like, which consists of D2, D3, and D4. Stimulation of members of the first group increases adenyl cyclase and potentiates neuronal excitability.89 Stimulation of members of the second group inhibits neuronal excitability by inhibiting adenyl cyclase and Ca currents and augmenting K currents. Such changes may play a role in the sensitization of WDR neurons. D1 agonists provoke release of CGRP and substance P from the spinal cord, which correlates with their ability to increase nociception at the level of the projection neurons.90
Inhibition of nociception involves mesolimbic, mesocortical, and nigrostriatal dopaminergic circuits.91–93 An understanding of how these functionally impinge on lower centers remains incomplete.94,95
Descending adrenergic pathways innervating the spinal cord derive primarily from the locus ceruleus, subceruleus, and medullary raphe nuclei.96–99 They exert descending inhibitory and facilitatory influence on spinal neurons by action on specific adrenoreceptors. The three major classes of such receptors, α1, α2, and β, can be further divided into ten subtypes, which are designated α1A, α1B, α1D, α2A, α2B, α2C, β1, β2, β3, and β4. The first three promote mobilization of intracellular Ca by coupling to phospholipase C and voltage-dependent Ca channels. The α2 class inhibits adenyl cyclase, facilitates K currents, and inhibits Ca currents, and the β class stimulates adenyl cyclase and, hence, neuronal excitability.
Under normal conditions, descending noradrenergic pathways display little spontaneous activity. Various events can provoke considerable change in their activity, which plays a significant role in the modulation of descending inhibition.85,100 For example, noxious stimuli activate release of noradrenaline in the DH of the spinal cord, which excites α2 receptors to effect “pain-induced analgesia.” Some investigators have developed a model of allodynia in which locus ceruleus neurons become activated.101 Such activation also potentiates descending noradrenergic inhibitory circuits.
Evidence exists for the facilitation of efferent antinociceptive adrenergic systems provoked by acute and chronic noxious stimuli. Much of this research has focused on the inhibition of nociception mediated by α2A receptors, which are negatively coupled to adenyl cyclase.97 On the contrary, data also suggest that higher nervous centers cause hyperexcitation of spinal neurons by increasing intracellular Ca, an effect that is mediated by the activation of α1 adrenoreceptors.97,102 Recent data corroborate these findings. Destruction of p75 nerve growth factor (NGF) receptor afferents (see later discussion) does not interfere with the hypersensitivity to mechanical stimuli that results from nerve injury. Such injury does disrupt the inhibitory action of descending α2 circuits, however, which exerts net reinforcement of hypersensitivity and neuropathic pain states.103
Descending facilitation also plays an important role in the pathophysiology of pain. For example, diminished cerebral GABAergic tone, such as may occur with direct brain injury, can lead to the disinhibition of descending facilitation.104 The rostromedial area of the ventral medulla seems to be involved because blocking electrical transmission there with lidocaine ameliorates experimental allodynia.8
Neurotensin (NT) is a 13–amino acid neuropeptide that is widely distributed throughout the central nervous system. It exerts its actions via NT receptors (NTRs), which consist of three subtypes: high affinity NTS1, low affinity NTS2, and NTS3. NT and NTRs regulate nociception at several levels in the descending CNS pathways. From a molar perspective, NT is a more potent analgesic than morphine. Investigators have reported that spinal injection of NT or one of its analogs modulates pain perception in rodent models of both persistent and neuropathic pain.105,106 Other studies have shown NT analog effectiveness in treating thermal, visceral, and persistent inflammatory pain, but there appears to be variability of efficacy depending on NTS1 or NTS2 selectivity, the animal pain model, and animal species.107–110
In addition to the rapid (msec time course) electrical signaling that occurs in the nervous system, neuron-neuron, neuron-glia, and neuron-muscle communication also occurs over a much longer time course. This has been perhaps most apparent at the skeletal neuromuscular junction, where disruption of the nerve innervating the muscle not only causes absence of voluntary movement, but also leads to profound changes in the muscle cell. We say that the nerve exerts a “trophic,” or nourishing effect, on the muscle. Without activity and the so-called neurotrophic influences of the nerve, the muscle loses many specialized features and becomes “atrophic.” Profound molecular changes occur, for example, in the muscle membrane’s electrical properties; the number, type, and distribution of acetylcholine receptors over the surface of the muscle fiber; and the proteins of the contractile mechanism in the sarcomeres.
A number of so-called trophic factors have been studied. Considerable information exists about the mechanisms and actions of NGF. More than 50 years ago, the requirement of NGF for the development of sensory and sympathetic neurons was described by Rita Levi-Montalcini.111–113 According to the theory, the neurotrophic factors made by target postsynaptic cells are transported retrogradely to the presynaptic neuronal soma, where they are required for the survival, development, and differentiation of the presynaptic neurons. Over the last half-century, many lines of research have tended to support Levi-Montalcini’s seminal theory.
Many neurotrophic factors besides NGF have been identified as playing a role in the integrity of nerve and muscle cells. More recently, investigators have realized the importance of such factors not only in the growth and development of immature cells, but also in the maintenance of differentiated properties of mature cells. Hence, the definition of neurotrophic factors in the sensory nervous system has been broadened to include those factors that permit the long-term growth, survival, or differentiation of neurons. The current knowledge of factors important in sensory nerve integrity focuses on the neurotrophin family and the glial cell line–derived neurotrophic factor (GDNF) family of factors.
The neurotrophin family consists of NGF, brain-derived neurotrophic factor (BDNF), neurotrophin-3 (NT3), neurotrophin-4/5 (NT4/5), and, in teleost fish Xiphophorus, neurotrophin-6 (NT6). The factors are basic proteins of about 120 amino acids and weigh about 13 kDa. Family members display considerable (~50%) homology of amino acids and exist as homodimers. The GDNF family of trophic factors belongs to the transforming growth factor-β (TGF-β) superfamily and includes GDNF, neurturin, persephin, and artemin. They signal by way of a complex composed of a tyrosine kinase transduction domain (RET) and a ligand-binding domain, the GDNF family receptor-α (GFR-α).114
All neurotrophins bind specifically, selectively, and with high affinity to one or more of the three known tyrosine kinase (Trk) receptors: TrkA, TrkB, and TrkC. NGF binds preferentially to TrkA, NT3 binds to all three Trks but exerts its effects mainly through TrkC, and both BDNF and NT4/5 bind preferentially to TrkB. In general, high-affinity binding (Kd ~ 10−11 M) constitutes only 10% to 30% of the total binding to Trk receptors, with the remainder being low affinity. Moreover, all members of the family bind with low affinity (Kd ~ 10−8–10−9 M) and somewhat different characteristics to a so-called low-affinity nerve growth factor receptor (LNGFR) or p75, which is also a member of the tumor necrosis factor receptor family.114,115
Structurally, Trk receptors consist of four main regions: The first is extracellular for ligand binding; the second is immunoglobulin G–like; the third spans the membrane (transmembrane domain); and the fourth is the kinase domain.115–117 Different isoforms can be distinguished on the basis of different amino acid residues of the extracellular domain.115,117 Still other isoforms have been identified that lack the kinase domain.115 The significance of these differences remains to be determined.
Based largely on studies of the action of NGF on PC-12 cells (a pheochromocytoma cell line), some of the steps of signal transduction following Trk activation have been described. Neurotrophin-receptor interaction activates multiple pathways. After ligand binding, receptor autophosphorylation of tyrosine residues occurs, which is likely mediated by dimerization as an obligatory step in the activation process.118,119 Other substrates such as phospholipase C (PLC-γ) are also phosphorylated.114 Interaction between phosphatidylinositol-3 kinase and the Trk domain occurs, but its significance is not clear.
The p75 receptor spans the cell membrane and contains both an intracellular region and an extracellular glycoprotein moiety. It appears to act, at least in part, to facilitate high-affinity neurotrophin-Trk binding. Although some investigators refute the role of p75 alone in neurotrophin signaling, others suggest that it may mediate NGF signal transduction through TrkA receptors.115,116 In cultured Schwann cells, the induction of NF-κB nuclear staining by NGF depends on the presence of the p75 receptor.120 NF-κB is a transcription factor, which translocates to the nucleus and regulates gene transcription after suitable activation.121 Such activation also enables NF-κB to bind particular DNA chains.120 It has also been hypothesized that NGF-p75-NF-κB activation is involved in the generation of hyperalgesia.120,122
Neurotrophin receptors are distributed widely and specifically throughout the nervous system. For example, so-called small, dark DRG, which display the neuropeptide marker CGRP, express predominantly TrkA receptors. So-called large, light DRG, which stain with the neurofilament antibody RT97, express TrkC. TrkB receptors appear only on certain types of DRG, which are not distinguishable by any histochemical staining property. For the most part, DRG expressing a Trk also express a p75. About one-third of DRG do not express either a Trk or a p75.
More recent investigation has promoted the concept that NGF also plays an important role in persistent pain states. Some studies suggest that NGF can not only modulate the sensitivity of sensory neurons, but can also mediate inflammatory pain. The precise mechanisms remain unclear, but some of the data are presented in the discussion that follows.
Different neurotrophins affect functionally different groups of DRG. Small-diameter DRG require NGF for survival. Two methods have been used to eliminate NGF during development: gene deletion and in utero antibody application.123,124 The resultant absence of NGF during development renders animals virtually devoid of small-diameter DRG, which express the nociceptive mediators CGRP and substance P. Such animals display significant hypoalgesia.114
Adult DRG do not possess the developmental requirement of NGF for survival. However, they are still exquisitely sensitive to NGF. For example, exogenous NGF added to cultured DRG elicits prolific neurite outgrowth125 and regulates the expression of both CGRP and substance P as well as the chemosensitivity of the ganglion cells.28 Interestingly, in the absence of NGF, capsaicin sensitivity, a specific proton-evoked current, and GABA sensitivity were diminished, but adenosine triphosphate (ATP) sensitivity was unaffected. NGF has recently been shown to increase selectively the expression of bradykinin-binding sites on cultured DRG mediated by a mechanism, which requires the p75 receptor.126 Such an increase has been associated with the increased excitability of nociceptors seen in chronic pain.15
Injection of minute amounts of NGF can produce pain and hyperalgesia at the site of injection.122,127 Because of the localization of these effects, some investigators have suggested that the NGF may also exert its actions on the peripheral terminals of nociceptors.127
Nerve activity regulates both neurotrophin synthesis and release from dendrites. Neurotrophins also promote transmitter release, which is mediated by the specific Trk receptor, from corresponding neurons. Hence, the suggestion has been made that neurotrophins act as retrograde messengers and regulate synaptic efficacy and neuronal plasticity.128
An interaction between NGF and sympathetic neurons during inflammation has been proposed. Sympathectomy leads to reduction of the short latency hyperalgesia produced by NGF.129,130 NGF may also cause peripheral sensitization by activating 5-lipoxygenase to generate leukotrienes. Martin and colleagues131 showed that leukotriene B4 sensitizes pain afferents to thermal and mechanical stimuli. Conversely, inhibition of 5-lipoxygenase prevents NGF-injection–produced hyperalgesia.132 Curiously, indomethacin does not prevent such hyperalgesia.133
Although systemically administered NGF does not penetrate the spinal cord, it does appear to affect central pain pathways.114 NGF may also produce increased neurotransmitter release from nociceptive afferents.134 Finally, NGF appears able to upregulate BDNF synthesis in some pain sensory cells. Hence, NGF seems to induce central sensitization in resetting the sensitivity of spinal processing of noxious stimuli.
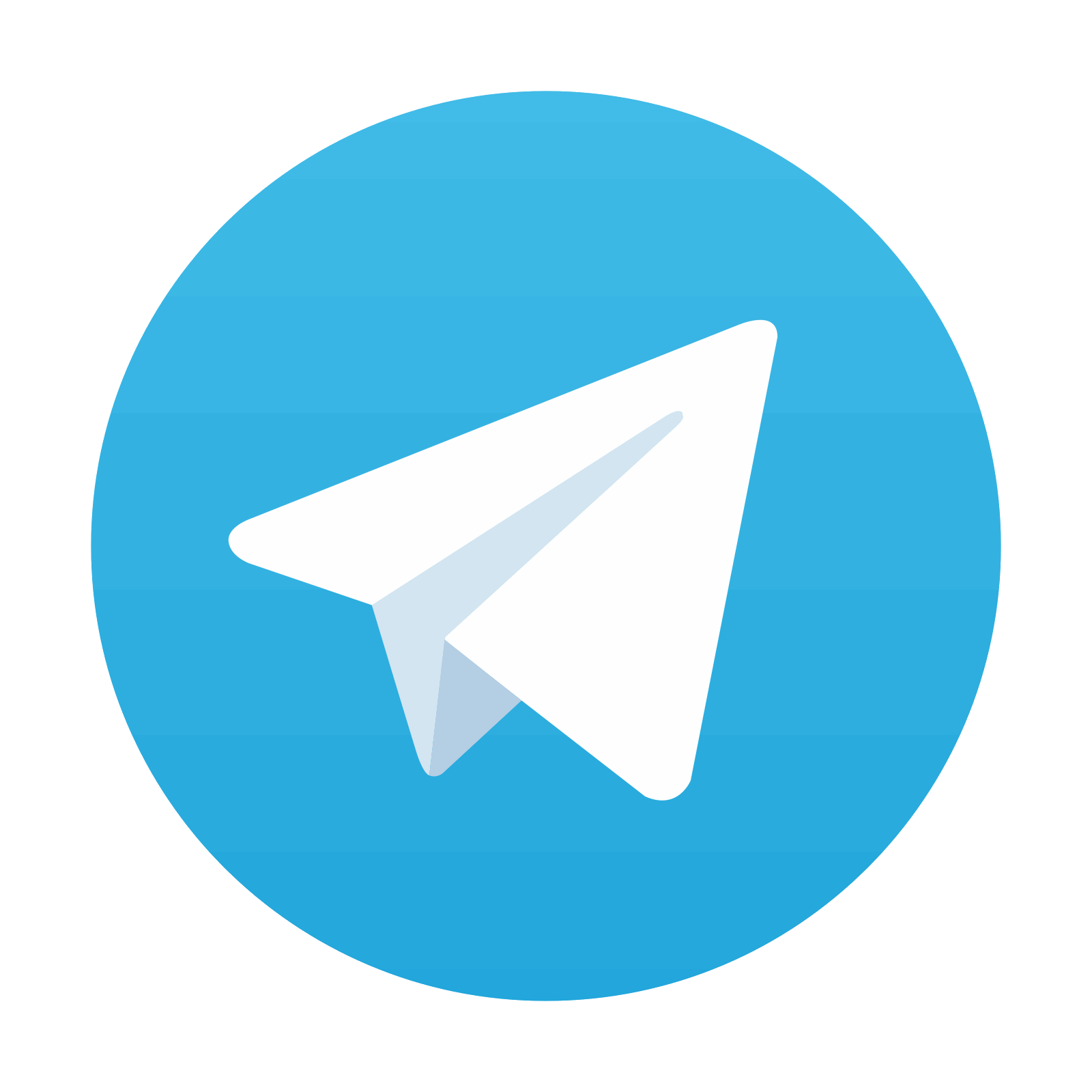
Stay updated, free articles. Join our Telegram channel

Full access? Get Clinical Tree
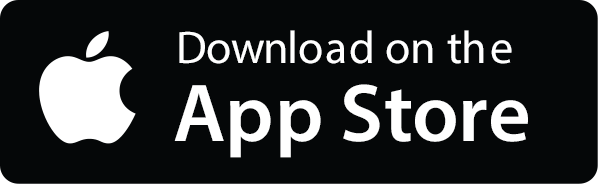
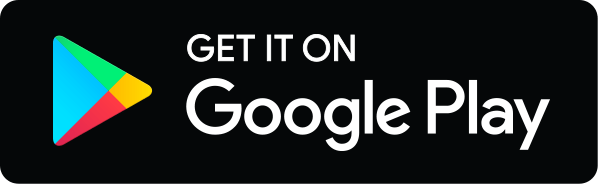