Chronic pain is an extremely complex and debilitating problem that presents itself in many ways in modern-day society. While there is clearly a biological substrate to chronic pain, there are also societal, psychosocial, financial, political, and public health components that bring far-reaching implications to research, diagnosis, and treatment of chronic pain disorders.
Remarkable advances in understanding pain and providing improved treatments have come through scientific discoveries, improved training and access to specialized clinics, organizations, national agendas, and industry and advocacy groups. However, our clinical armamentarium is relatively limited in providing relief in chronic pain conditions. In the past, the basic therapy has included, for the most part, (1) drugs, mostly belonging to three classes—opioids, nonsteroidal anti-inflammatory drugs, and local anesthetics; (2) interventional treatments; (3) psychological biobehavioral therapy; and (4) some adjuvant medications, as well as complementary and alternative therapies. For all of these efforts, the number of outcome studies of nonpharmacological trials is limited, and most pharmacological studies show poor efficacy of treatment in chronic pain.1 Pain researchers, pharmaceutical companies, and clinicians have struggled to break the barriers of finding treatments for pain that are both specific and efficient and have limited side effects.
Part of the problem we have faced is a new realization that chronic pain is a disease of the brain. Until recently, there has been a lack of ability to measure changes in the brain that are a consequence of chronic pain. Anatomic, functional, and chemical neuroimaging have opened the door to new vistas and new opportunities for a better understanding of chronic pain, better diagnostic possibilities, and, perhaps, better drug treatments to be developed. Although genetic and other molecular approaches in the pain field have shown tremendous advances, only in recent years has brain imaging contributed to the revolution in understanding pain, greatly changing the field of pain research.
The major insight that emerged from neuroimaging studies is that chronic pain is a disease of the brain, and, thus, all therapeutic modalities will need to take this into consideration. The ability to explore the human brain in human volunteers or patients has dramatically changed our understanding of pain. Imaging has the ability to define theoretical constructs of numerous thinkers in the field of brain processing in chronic pain in the human condition. Imaging has allowed unprecedented interrogation of brain systems in terms of brain circuitry, the effects of analgesics on neural networks, transition of acute into chronic pain, definition of brain regions that heretofore may not have been considered important (e.g., nucleus accumbens, striatal regions), brain plasticity including functional and morphological changes, networks that are involved in the placebo response, and alterations in neurochemistry in chronic pain (Fig. 12-1).
It is now increasingly understood that pain represents a multifaceted process shaped by a multitude of factors (somatosensory, emotional, cognitive, genetic) and, in turn, affecting behavioral responses as well as producing an altered brain state. Functional brain imaging may allow us to provide an objective measure of pain—one that may be complex and require taking into account sensory, emotional, and modulatory processes in the context of expectations and life experiences. Imaging pain has already produced far-reaching changes in the way we think about chronic pain2–5 and define a signature of changes in the brain that contribute or are part of the chronic pain syndrome, which will eventually result in better pain treatments.
The development of a number of noninvasive magnetic resonance imaging (MRI) methods, including morphological/anatomical imaging of gray matter (voxel-based morphometry [VBM]), white matter tract connectivity (diffusion tensor imaging [DTI]), functional magnetic resonance imaging (fMRI), and magnetic resonance spectroscopy (MRS), has paved the way to an unprecedented boom in brain research (Fig. 12-2). MRI methods, as well as other techniques like magnetic encephalography (MEG) and near-infrared spectroscopy (NIRS), are rapidly evolving as novel analytical methods and more sophisticated equipment become available. Because their noninvasive nature allows in vivo longitudinal studies of the dynamic structural and functional changes in the brain as a result of pain, these approaches (described in Fig. 12-2) have produced a shift in our understanding of chronic pain.
From the original definition as an “unpleasant sensory and emotional condition,” chronic pain is now understood to be a multidimensional “disease affecting the central nervous system,” influenced by a variety of biological and psychosocial factors, such as genetics, hormones, emotions, memories, or social expectations.6,7
VBM measures the local concentration of gray matter in different brain voxels. In the pain field, VBM has been used to measure changes in the volume of subcortical structures, including the hippocampus, basal ganglia, thalamus, and amygdale.8 Most recently, techniques that allow measurement of small changes in cortical thickness have been developed (http://surfer.nmr.mgh.harvard.edu). These techniques will allow documentation of alterations of gray matter that occur in chronic pain conditions.
DTI investigates white matter tract integrity by measuring microstructural changes in directional water diffusion in the brain.9,10 In the pain field, the technique has been used in fibromyalgia to document alterations in the brain’s microcircuitry in the thalamus, insula, amygdala, hippocampus, and frontal and anterior cingulate cortex.11 By overlaying DTI and fMRI brain maps, future studies may help advance our understanding of functional anatomical mapping in chronic pain conditions.
Blood oxygen level–dependent (BOLD) fMRI measures changes in the local concentration of deoxyhemoglobin and provides an indirect index of neuronal activity. Several BOLD methods have been applied to pain research and have revealed the neural correlates of pain perception and modulation by characterizing the brain response to evoked stimuli (e.g., pain, allodynia), task-driven responses, or drugs (phMRI).
Evoked-stimuli fMRI has been commonly used in the pain field because of the relative ease of presenting well-characterized objective stimuli during the imaging session (i.e., cold and hot temperatures, somatosensory stimulation). Functional imaging has helped uncover the neural circuitry involved in pain processing and modulation and described the brain areas that reflect sensory, cognitive, and affective dimensions of pain.12
This approach uses low-frequency BOLD signal fluctuations to evaluate the functional brain connectivity during resting states as opposed to task performance. These default mode networks are consistent across healthy subjects13 and can be used to define disease phenotypes by differentiating disease states (i.e., chronic back pain);14 from healthy states. Simultaneous imaging of structural and functional connectivity may provide a better understanding of pathological processes by uncovering changes in specific brain networks as a result of disease.
Pharmacological MRI (phMRI) investigates the functional effects of drugs on the brain and links levels of drug exposure to the changes in evoked responses or RSN activity. More recently, arterial spin labeling (ASL) methodology, which measures blood flow changes with improved contrast and signal-to-noise ratio through magnetization of the blood, has been used to measure the regional dose-related effects of drugs on brain function.15 These measures can be used to monitor the functional effects of drug receptor binding and the dose relationship of central responses and to provide objective indices of therapeutic efficacy in pain conditions.
MRS is used to noninvasively assess different metabolites and neurotransmitters in the brain,16 to characterize the composition of neuronal and synaptic markers (e.g., glutamate, glutamine, and g-aminobutyric acid [GABA]) in different brain regions and to identify relationships between disease states and changes in the brain metabolic or chemical composition. MRS techniques have been widely applied in the study of psychiatric diseases as well as pain syndromes.17 Recently, analytic technologies such as 13C-based flux analysis have been developed. This fluxometric method allows real-time analysis of metabolic changes in brain networks. Although this technology has so far been applied to mammalian cells grown in tissue culture but not to the human brain in vivo, it represents a potentially promising technique that, in the future, could aid the understanding of the disease-associated metabolic changes in the brain.
A novel approach that could aid the localization of functionally activated brain regions in the brain is mapping of multiple neurotransmitter receptors sites.18 Although not yet applied to pain conditions, this approach may provide a better understanding of the underlying basis of neurotransmission in healthy and disease states by correlating brain data obtained through different techniques (anatomical, functional) with cytoarchitectonical and molecular brain maps.
NIRS or diffuse optical tomography (DOT) is a noninvasive technique that can detect changes in blood hemoglobin concentrations associated with neural activity and, therefore, assess the brain function through an intact skull in human subjects.19 NIRS has a great potential in measuring pain effects on the brain. Recent research has shown that it is possible to record a pain-specific signal using NIRS, and this signal was similar to that observed in previous fMRI studies.20
MEG is a noninvasive imaging technique that measures the magnetic field produced by synchronized synaptic currents in the brain. Similar to electroencephalography (EEG), MEG measures parameters of neuronal activity directly. A rich literature exists that describes the correlation between neuronal oscillations as recorded by MEG and different brain functions, including attention, visual processing, or motor planning.21 Recently, a growing number of studies have employed both MEG and fMRI, taking advantage of the strengths of each method (i.e., excellent spatial resolution of fMRI and the millisecond temporal resolution of MEG) to help uncover the mechanisms of cortical processing.22
The early pain imaging studies used positron emission tomography (PET) and reported on pain responses to noxious heat. Since then, functional imaging studies in healthy volunteers have either confirmed brain regions involved in pain processing (thalamus, somatosensory cortex, anterior cingulate cortex) or added important new components of pain processing (e.g., nucleus accumbens, insula, dorsolateral prefrontal cortex, basal ganglia, and cerebellum). Thus, there is a new complexity in understanding brain function in pain that allows for sensory, emotional/affective, modulatory, and cognitive responses to pain.
Functional imaging research in healthy subjects has provided new insights into these regions and their possible role in pain. As such, these studies have been invaluable in providing a basis to explore changes in the clinical condition and evaluation of analgesic drugs on brain function. (See Fig. 12-3.)
In the first imaging study of pain, “Multiple Representations of Pain in the Human Cerebral Cortex,” Talbot and colleagues reported on activation in several brain regions in response to noxious heat, including the contralateral anterior cingulate cortex and primary and sensory somatosensory cortices.23 This study opened up the path for brain imaging of pain, which initially focused on “expected areas” such as the thalamus.
What these studies did is raise issues of pain processing in regions beyond the primary somatosensory cortex.24,25 Subsequently, numerous cortical regions have been shown across pain imaging studies to be activated by painful stimuli (reviewed in Treede et al., Peyron et al., and Apkarian et al.26–28).
Overall, these meta-analyses reported that pain produced activation in the primary and secondary somatosensory, insular, anterior cingulate and prefrontal cortices and the thalamus. Some regions such as the cerebellum,29 the anterior cingulate, and insular cortices, are consistently activated across most functional imaging pain studies but have remained an enigma as to a specific role in pain processing. More recent studies have begun to dissect the pain-induced brain activation as it relates to specific functions, such as sensory processing, emotional/affective and cognitive processing, and pain modulatory processing. The more focused studies have allowed for a better understanding of these regions in pain function.
Somatosensory processing of pain stimuli classically includes the thalamus and somatosensory cortices. Other studies have used imaging to trace a pain pathway (trigeminal) from the periphery (ganglion) to the dorsal horn (trigeminal nucleus in the brainstem) and traditional sensory pathways through the thalamus and to the cortex.30,31 Insula is a recent addition to brain regions involved in the evaluation of pain intensity, and imaging studies have uncovered the somatosensory representation of pain in the insular cortex.32 Still, investigations of the insular functions warrant a broader look at the potential involvement in sensory and emotional evaluative components, as well as interoception (i.e., sensing the physiological condition of the body). As it turns out, this region is intricately involved in complex pain and analgesic processing.
The notion that pain is not only a sensory but also an emotional experience required neuroimaging to help define an underlying brain circuitry that contributes to the emotional processing of pain. Generally, greater acute and chronic pain intensity is associated with higher negative emotional state. The experience of pain is able to trigger emotional responses, and the emotional state can also affect the perception of pain. Studies indicated that the classic reward circuitry, which includes regions such as the amygdala, nucleus accumbens, and orbitofrontal lobe, were all activated by noxious heat.33 Specific connectivity between entorhinal cortex and cingulate regions involved in anxiety and anticipation of pain was also described.34 Such studies contributed to the characterization of a brain network that could underlie, in addition to emotional responses to pain, the placebo and nocebo responses,35,36 as well as empathy of pain in others.37
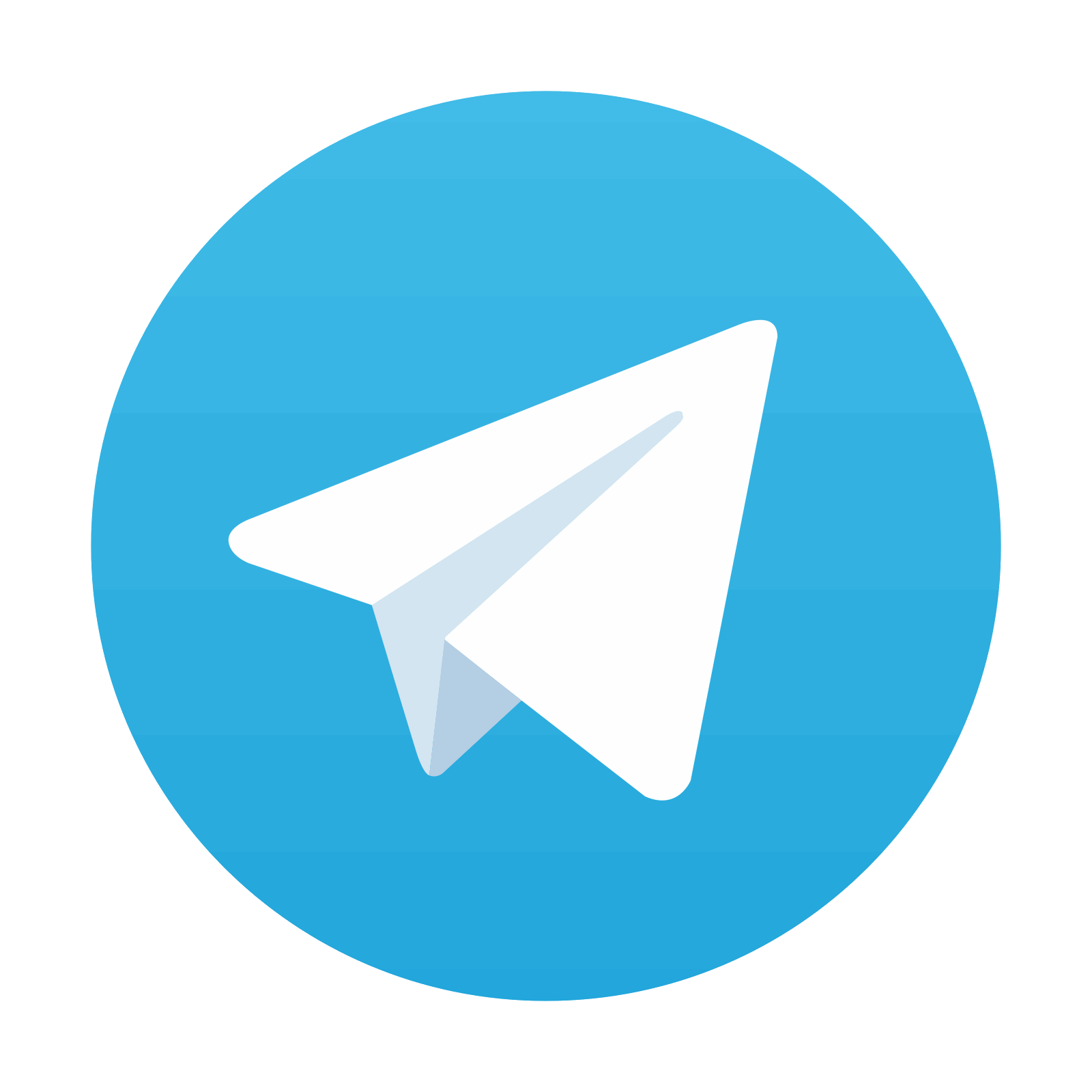
Stay updated, free articles. Join our Telegram channel

Full access? Get Clinical Tree
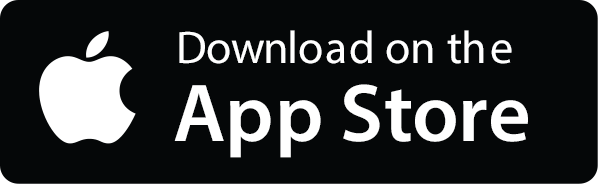
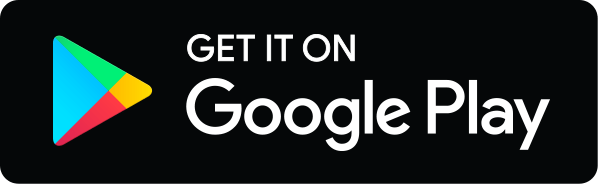