Andrea D. Furlan1,2,3 & Laura Murphy1,4,5 1 KITE, Toronto Rehabilitation Institute, University Health Network, Toronto, Ontario, Canada 2 Division of Physical Medicine & Rehabilitation, Department of Medicine, Faculty of Medicine, University of Toronto, Toronto, Ontario, Canada 3 Institute for Work & Health, Toronto, Ontario, Canada 4 Department of Pharmacy, University Health Network, Toronto, Ontario, Canada 5 Leslie Dan Faculty of Pharmacy, University of Toronto, Toronto, Ontario, Canada We acknowledge Dawn A. Sparks & Gilbert Fanciullo as authors of the previous version of this chapter. Opioids are front‐line treatment in the management of acute and cancer pain. In chronic pain, they are behind other non‐pharmacological options and are a third‐line pharmacological option, but not appropriate for all situations. The role of opioids in the management of chronic pain has been more controversial and recently impacted by an emerging global opioid crisis, mostly documented in North America, Germany and Australia [1, 2]. In this context, the scientific and clinical communities in these countries have developed guidelines regarding the use of opioids in chronic pain [3–6]. These guidelines have some variation and the Canadian guideline has been referenced here for purposes of illustration [3]. With increased awareness of risks and harms associated with opioids, actions have been taken by government, regulatory bodies and some clinicians [7]. These actions have unfortunately led to unintended consequences for people living with pain who were using opioids as part of their overall pain management [7, 8]. This illustrates the importance of maintaining access to appropriate and safe use of opioids for patients with chronic pain while balancing individual and societal harms. The aim of this chapter is to review the pharmacology and physiology of opioids, as well as to provide rationale from which to base clinical decision‐making when using opioids in the management of patients with chronic pain. Opioids activate the endogenous opioid receptors in the body. Opioids available for clinical use include both naturally occurring substances (e.g. morphine or codeine), synthetic substances (e.g. methadone, fentanyl) and semi‐synthetic substances (e.g. oxycodone and hydromorphone). Opioid receptors and endogenous opioids or peptides (e.g. endorphins, enkephalins, dynorphins) form an intricate neurotransmitter arrangement known as the endogenous opioid system. The opioid receptors are found within the cellular membranes and are comprised of numerous glycoproteins. Opioid receptors belong to a superfamily of guanine (G) protein‐coupled receptors [9, 10]. These G proteins act as second messengers and assist in regulating cell activities. Table 18.1 Opioid receptors and physiological effects DOP= delta opioid receptor; KOP=kappa opioid receptor; MOP=mu opioid receptor; NOP= nociception orphanin FQ peptide receptor; ORL‐1= opioid‐receptor‐like receptor 1. There are multiple opioid receptor subtypes: mu (MOP), delta (DOP) kappa (KOP) and the nociception orphanin FQ peptide receptor (NOP), initially named the opioid‐receptor‐like receptor 1 (ORL‐1) [9, 10]. Table 18.1 [11] outlines opioid receptor distribution and physiological effects. Most opioids can be classified by their action and affinity at the receptor. They can be classified as full agonists (e.g. codeine, fentanyl, heroin, hydromorphone, morphine, oxycodone, tramadol), partial agonists (e.g. buprenorphine) or antagonists (e.g. naloxone, naltrexone). Partial agonists are able to compete with a full agonist, such as morphine, by lowering its efficacy when acting at the same receptor. Some opioids have mixed agonist–antagonist activity (e.g. buprenorphine, pentazocine). In general, agonists selective for MOP or DOP receptors are analgesic and rewarding, whereas KOP agonists are dysphoric. There are no clinically available selective drugs available that work via DOP, KOP, or NOP receptors. Opioid receptors vary widely in their distribution between individuals. On a cellular level, receptors are located in both presynaptic and postsynaptic positions. The dorsal horn of the spinal cord, medulla oblongata, thalamus and cortex are involved in ascending pain transmission whereas the periaqueductal gray matter, nucleus raphe magnus and ventral medulla utilize the descending pain pathways, all of which possess opioid receptors. These receptors are activated by endogenous opioids as well as exogenous opioids and, in turn, modify nociceptive transmission, modulation and perception. Activation of opioid receptors leads to closing of voltage sensitive calcium channels, stimulation of potassium efflux and hyperpolarization of the nerve and reduction of cyclic adenosine monophosphate (cAMP) production via inhibition of adenylyl cyclase, resulting in reduced neuronal cell excitability [9]. This action also inhibits the transmission of nerve impulses along with inhibition of excitatory neurotransmitter release [9]. Clinicians should consider pharmacological properties, routes of administration and formulations available and potency of opioids. The type of pain that responds better to opioids are acute nociceptive and neuropathic pain. There is controversy in using opioids to treat nociplastic chronic pain, defined as pain arising from altered nocicepton [12], because opioids are implicated in inducing paradoxical hyperalgesia [13], which can be a complicating factor. Opioid potency is generally described in comparison to morphine. Hydromorphone, for example is 5 times more potent than morphine, and oxycodone is 1.5 times more potent. An oral opioid conversion table from the Canadian Guideline for Opioids for Chronic Non‐cancer Pain, is provided as Table 18.2. It also includes equivalent doses per day for threshold morphine equivalent doses (MED) described in the Canadian guideline. There is variability in opioid equivalence calculations, particularly for buprenorphine, methadone and from fentanyl transdermal to other oral opioid formulations [14]. Side effect profiles may also be considered in selection of the appropriate opioid. Differences exist depending on opioid receptor activity and other mechanisms of action. For example, morphine should be prescribed with caution to people with impaired kidney function, and tramadol can lead to serotonin syndrome in combination with other serotonergic medications. The potential for pharmacodynamic drug interactions is present across the opioid class of medications, in particular with central nervous system depressants such as benzodiazepines, gabapentinoids and alcohol, which in combination increase risk of respiratory depression and death [15–18]. Table 18.2 Oral opioid conversion table Source: Busse JW. The 2017 Canadian Guideline for Opioids for Chronic Non‐Cancer Pain. Hamilton (ON): McMasterUniversity; 2017. Reproduced with permission of CMA Joule Inc. MED=Milligram morphine equivalent dose. * The maximum recommended daily dose of tramadol is 300mg‐400mg depending on the formulation. Opioids vary in their pharmacokinetics, i.e. absorption, distribution, metabolism and elimination from the body. Opioids are well absorbed when administered orally, however their onset and duration of action depend on numerous factors including lipid solubility, protein binding, ionization state, molecular size and membrane physiochemical properties. Differences in opioid metabolism in the liver also have clinical implications. As an example, morphine and hydromorphone undergo metabolism through glucuronidation and are therefore unlikely to have pharmacokinetic drug interactions. Morphine and hydromorphone are converted to active metabolites and excreted in the urine. In the presence of renal impairment, a morphine metabolite has significant risk of accumulation and toxicity. Codeine, tramadol and tapentadol are considered less potent opioids; codeine and tramadol are approximately 6 times less potent than morphine, tapentadol is 3 times less potent [19]. Pharmacokinetic differences within the population may have significant impact on the efficacy and safety of codeine and tramadol, both prodrugs. Codeine must be metabolized to morphine by the cytochrome P450 system in the liver, specifically CYP2D6. There are high levels of polymorphism in the population for CYP2D6, impacting many people’s ability to convert it to its active metabolite. Tramadol is a dual action analgesic blocking reuptake of norepinephrine and serotonin as well as acting as a full agonist MOP receptors. It has low affinity for opioid receptors, but similar to codeine, tramadol is also converted by CYP2D6 to a MOP full agonist, O‐desmethyl tramadol with a higher binding affinity, and so is affected in a similar way by the polymorphism in the population [20]. Tapentadol also has a dual mechanism of action, in addition to activity as a MOP receptor agonist with less affinity than morphine, and it also inhibits norepinephrine reuptake. Tapentadol exerts effects without a pharmacologically active metabolite. Fentanyl is a potent MOP receptor agonist, 100 times more potent than morphine. It is available as a transdermal formulation for use in chronic pain [21]. A rule of thumb for morphine to fentanyl conversion is that 2 mg of oral morphine is approximately equivalent to 1 mcg/hour transdermal fentanyl patch [22]. When switching to or from fentanyl patches, conservative dosing is suggested because of the range of inter‐individual absorption from the patch and equianalgesic ratio with oral opioids. Attention to timing related to patch application and removal is also important in this switch [22]. Dose titration and tapering are limited by availability of patch strengths. Methadone is a MOP receptor agonist and N‐methyl‐D aspartate (NMDA) antagonist. Its analgesic duration of action is approximately 6–8 hours, as a result of being highly lipophilic and having a long elimination half‐life. It is important for clinicians to be familiar with the unique pharmacokinetics of methadone [23]. There is considerable inter‐individual variation in estimates of oral bioavailability and elimination half‐life. The oral bioavailability of methadone is different at treatment initiation compared to later on in treatment when the individual is stable on the methadone dose. This may be explained by auto‐induction of methadone metabolism, which predominantly occurs by the cytochrome P450 3A4 enzyme system. The approximate morphine:methadone equivalence ratio fluctuates with increasing doses. Methadone is relatively more potent at higher dose. Thus, at morphine dosage of less than 100 mg/day the approximate equivalence ratio of morphine to methadone is 4:1; at dosage between 100 and 300 mg MED per day it becomes 8:1 and at morphine dosage greater than 300 mg/day the ratio is between 12:1 and 20:1. These ratios are intended only as a guideline. Repeated dosing and accumulation of opioids at the receptor as well as their efficacy at the receptor can alter the analgesic interval and the dosing regimen needed. P450 system, and have the potential to possibly increase or decrease methadone’s effects, requiring clinical assessment and potentially dose adjustment if these medications are started and stopped. In addition to CYP 3A4, methadone is also metabolized to a lesser extent by CYP1A2, 2B6, 2C8, 2C9, 2C19, and 2D6 enzymes, and is also a weak inhibitor of CYP 2D6, drugs involved in these systems have the potential to interact. Buprenorphine is a partial agonist at the MOP and NOP receptors, and a weak KOP receptor antagonist and DOP receptor agonist. For pain, it is used as a transdermal patch [24], and for opioid use disorder it is available as a sublingual formulation in combination with naloxone, and as an injection for subcutaneous use. It is important to note that the classification of buprenorphine as a partial agonist does not translate to partial analgesic effects [25]. Its complex receptor interactions have the potential for other beneficial effects; it has been shown to effectively reduce depressive symptoms in patients unresponsive to conventional antidepressants [26], however these effects require further study.
Chapter 18
Opioids
Introduction
Pharmacology
Receptor
Location
Physiological effects
MOP
Brain, spinal cord, peripheral nociceptors in the gastrointestinal tract, others.
induce reactions at the supraspinal level
Analgesia, euphoria, respiratory depression, emesis, immune suppression
DOP
Peripheral nociceptors, spinal cord, brain
Analgesia, immune stimulation, respiratory depression
KOP
Peripheral nociceptors brain, spinal cord
Analgesia, sedation, dysphoria, diuresis, miosis
NOP (ORL‐1)
Peripheral nociceptors, brain, spinal cord
Hyperalgesia, sedation, pain responses, physical tolerance to MOP agonists
Opioids
To convert to oral morphine equivalent, multiply by:
To convert from oral morphine, multiply by:
50 MED equivalent dose
90 MED equivalent dose
Codeine
0.15 (0.1‐0.2)
6.67
334mg/day
600mg/day
Hydromorphone
5
0.2
10mg/day
18mg/day
Morphine
1
1
50mg/day
90mg/day
Oxycodone
1.5
1
33mg/day
60mg/day
Tapentadol
0.3‐0.4
2.5‐3.33
160mg/day
300mg/day
Tramadol
0.1‐0.2
6
300mg/day
540* mg/day
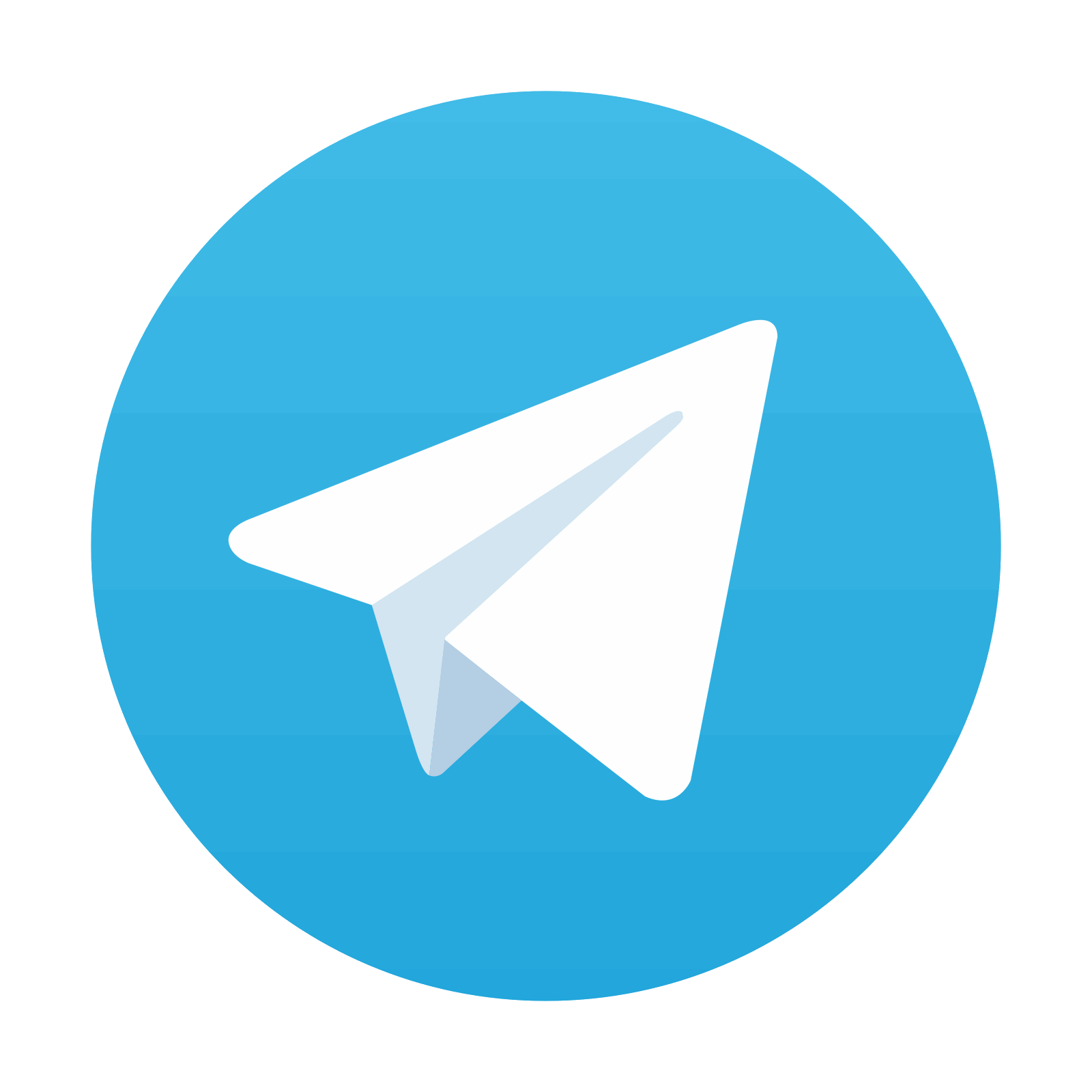
Stay updated, free articles. Join our Telegram channel

Full access? Get Clinical Tree
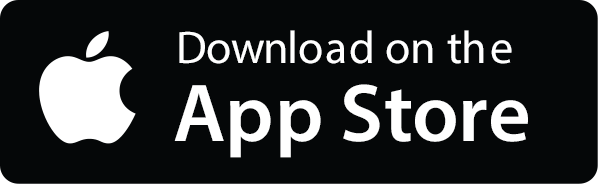
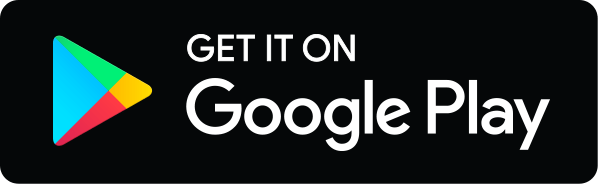