Chemical Structure of Opium Alkaloids
The active components of opium can be divided into two distinct chemical classes: phenanthrenes and benzylisoquinolines. The principal phenanthrene alkaloids present in opium are morphine, codeine, and thebaine (Fig. 7-1). The principal benzylisoquinoline alkaloids present in opium, which lack analgesic activity, are papaverine and noscapine. The three rings of the phenanthrene core are composed of 14 carbon atoms. The fourth piperidine ring includes a tertiary amine nitrogen and is present in most opioid agonists. At pH 7.4, the tertiary amine nitrogen is highly ionized, making the molecule water soluble. These are chiral molecules, with levorotatory isomers being biologically active at opioid receptors.

Semisynthetic Opioids
Simple modification of the morphine molecule yields many derivative compounds with differing properties. For example, substitution of a methyl group for the hydroxyl group on carbon 3 results in methylmorphine (codeine). Substitution of acetyl groups on carbons 3 and 6 results in diacetylmorphine (heroin). Thebaine has insignificant analgesic activity but serves as the precursor for etorphine (analgesic potency >1,000 times morphine).
Synthetic Opioids
Synthetic opioids contain the phenanthrene nucleus of morphine but are manufactured by synthesis rather than chemical modification of morphine. Morphine derivatives (levorphanol), methadone derivatives, benzomorphan derivatives (pentazocine), and phenylpiperidine derivatives (meperidine, fentanyl) are examples of groups of synthetic opioids. There are similarities in the molecular weights (236 to 326) and pKs of phenylpiperidine derivatives and amide local anesthetics.
Fentanyl, sufentanil, alfentanil, and remifentanil (Fig. 7-2) are synthetic opioids that are widely used to supplement general anesthesia or as primary anesthetic drugs in very high doses. There are important clinical differences between these opioids.2–4 The major pharmacodynamic differences between these drugs are potency and rate of equilibration between the plasma and the site of drug effect (biophase).

Mechanism of Action
Opioids act as agonists at specific opioid receptors at presynaptic and postsynaptic sites in the central nervous system (CNS) (mainly the brainstem and spinal cord) as well as in the periphery.5–7 These same opioid receptors normally are activated by three endogenous peptide opioid receptor ligands known as enkephalins, endorphins, and dynorphins. Opioids mimic the actions of these endogenous ligands by binding to opioid receptors, resulting in activation of pain-modulating (antinociceptive) systems.
Existence of the opioid in the ionized state appears to be necessary for strong binding at the anionic opioid receptor site. Only levorotatory forms of the opioids exhibit agonist activity. Indeed, the naturally occurring form of morphine is the levorotatory isomer. The affinity of most opioid agonists for receptors correlates well with their analgesic potency.
The principal effect of opioid receptor activation is a decrease in neurotransmission.8 This decrease in neurotransmission occurs largely by presynaptic inhibition of neurotransmitter release (acetylcholine, dopamine, norepinephrine, substance P), although postsynaptic inhibition of evoked activity may also occur. The intracellular biochemical events initiated by occupation of opioid receptors with an opioid agonist are characterized by increased potassium conductance (leading to hyperpolarization), calcium channel inactivation, or both, which produce an immediate decrease in neurotransmitter release.
All opioid receptor classes couple to intracellular guanine (G) proteins. Upon binding of an opioid agonist to the extracellular domain of the receptor, the receptor changes shape, which activates the G protein bound to its intracellular domain. The G protein replaces its bound guanine diphosphate (GDP) with guanine triphosphate (GTP) and dissociates into two active subunits. Subsequent mechanisms include inhibition of adenylate cyclase, decrease the conductance of voltage-gated calcium channels, or opening of inward-flowing potassium channels. Any of these effects ultimately results in decreased neuronal activity. Opioid receptors also modulate the phosphoinositide-signaling cascade and phospholipase C. The prevention of calcium ion inflow results in suppression of neurotransmitter release (substance P) in many neuronal systems. Hyperpolarization results from actions at potassium channels, thus preventing excitation or propagation of action potentials. Opioid receptors may regulate the functions of other ion channels including excitatory postsynaptic currents evoked by N-methyl-D-aspartate (NMDA) receptors.
Opioid receptor–mediated inhibition of adenylate cyclase is not responsible for an immediate effect but may have a delayed effect, possibly via a reduction in cyclic adenosine monophosphate (cAMP)–responsive neuropeptide genes and reduction in neuropeptide messenger RNA concentrations. Depression of cholinergic transmission in the CNS as a result of opioid-induced inhibition of acetylcholine release from nerve endings may play a prominent role in the analgesic and other side effects of opioid agonists. Opioids do not alter responsiveness of afferent nerve endings to noxious stimulation nor do they block conduction of nerve impulses along peripheral nerves (as opposed to local anesthetics).
Opioid Receptors
Opioid receptors are classified as µ, δ, and κ receptors8,9 (Table 7-2). The names of the three subtypes developed from the ligands originally found to bind to them or their tissue of origin (mu—morphine, kappa—ketocyclazocine, delta—isolated from mouse vas deferens). These opioid receptors belong to a superfamily of seven transmembrane-segment G protein–coupled receptors that includes muscarinic, adrenergic, γ-aminobutyric acid, and somatostatin receptors. The opioid receptors have been cloned and their amino acid sequences defined.10,11 A single µ-receptor gene has been identified and six distinct µ receptors subtypes have been characterized.

In the brain, opioid receptors are primarily found in the periaqueductal gray, locus ceruleus, and the rostral ventral medulla. In the spinal cord, opioid receptors are found both on interneurons and primary afferent neurons in the dorsal horn. Consequently, direct application of opioid agonists to the spinal cord can produce intense analgesia.12 Outside the CNS, opioid receptors are found on sensory neurons and immune cells. Immune cells recruited to sites of inflammation also secrete opioid peptides to provide local analgesia.13 For example, intraarticular morphine is known to produce analgesia after knee surgery, presumably through action on peripheral nerves.14
The µ receptors are principally responsible for supraspinal and spinal analgesia. Theoretically, activation of a subpopulation of µ receptors (mu1) is speculated to produce analgesia, whereas mu2 receptors are responsible for hypoventilation, bradycardia, and physical dependence. Nevertheless, cloning of the µ receptors does not support the existence of separate mu1 and mu2 receptor subtypes.9 It is possible that such subtypes result from posttranslational modification of a common precursor protein. Whether β-endorphins or even morphine itself is the endogenous ligand for µ receptors is unclear.15 Endomorphins are peptides with high affinity and selectivity for µ receptors that are present in the brain.
Activation of κ receptors results in inhibition of neurotransmitter release via N-type calcium channels. Respiratory depression characteristic of µ receptor activation is less prominent with κ receptor activation, although dysphoria and diuresis may accompany activation of these receptors. κ receptor–mediated analgesia may be less effective for high-intensity painful stimulation than µ opioid–mediated. Opioid agonist–antagonists often act principally on κ receptors. δ receptors respond to the endogenous ligands known as enkephalins, and these opioid receptors may serve to modulate the activity of the µ receptors.
Functional and physical interactions between these receptor subtypes have been noted.16,17 Heteromerization between µ and δ opioid receptors leads to distinct receptor pharmacology in that doses of δ receptor ligands (agonists and antagonists) too low to trigger signaling can potentiate the binding and signaling of µ receptor agonists. Chronic, but not acute, morphine treatment results in an increase in µ-δ heteromers in key areas of the CNS that are implicated in pain processing.18
Endogenous Pain Modulating Mechanisms
The logical reason for the existence of opioid receptors and endogenous opioid agonists is to function as an endogenous pain suppression system. Once pain is consciously perceived, it has served its purpose and it is reasonable to posit that the ability to dampen this perception would have a survival benefit. Opioid receptors are located in areas of the brain (periaqueductal gray matter of the brainstem, amygdala, corpus striatum, and hypothalamus) and spinal cord (substantia gelatinosa) that are involved with pain perception, integration of pain impulses, and responses to pain (Fig. 7-3).19 It is speculated that endorphins inhibit the release of excitatory neurotransmitters from terminals of nerves carrying nociceptive impulses. As a result, neurons are hyperpolarized, which suppresses spontaneous discharges and evoked responses. Analgesia induced by electrical stimulation of specific sites in the brain or mechanical stimulation of peripheral areas (acupuncture) most likely reflects release of endorphins.20 Even the analgesic response to a placebo may also involve the release of endorphins. Sustained pain and stress induces the regional release of endogenous opioids interacting with µ opioid receptors in a number of cortical and subcortical brain regions. The activation of the µ opioid receptor system is associated with reductions in the sensory and affective ratings of the pain experience, with distinct neuroanatomic involvement.21,22

In addition, a recent study demonstrated that positive treatment expectancy substantially enhanced (doubled) the analgesic benefit of remifentanil, whereas negative treatment expectancy abolished remifentanil analgesia.23 These subjective effects were substantiated by significant changes in the neural activity in brain regions involved with the coding of pain intensity. The positive expectancy effects were associated with activity in the endogenous pain modulation system, and the negative expectancy effects with activity in the hippocampus.23 On the basis of subjective and objective evidence, we contend that an individual’s expectation of a drug’s effect critically influences its therapeutic efficacy and that regulatory brain mechanisms differ as a function of expectancy.
Common Opioid Side Effects
An ideal opioid agonist would have a high specificity for receptors, producing desirable responses (analgesia) and little or no specificity for receptors associated with side effects. To date, however, all opioids possess similar side effects that vary only in degree. Therefore, a focus on the effects of morphine provides a suitable starting point.
Cardiovascular System
Morphine, even in large doses, given to supine and normovolemic patients is unlikely to cause direct myocardial depression or hypotension. The same patients changing from a supine to a standing position, however, may manifest orthostatic hypotension and syncope, presumably reflecting morphine-induced impairment of compensatory sympathetic nervous system responses. For example, morphine decreases sympathetic nervous system tone to peripheral veins, resulting in venous pooling and subsequent decreases in venous return, cardiac output, and blood pressure.24
Morphine can also evoke decreases in systemic blood pressure due to drug-induced bradycardia or histamine release. Morphine-induced bradycardia results from increased activity of the vagal nerves, which probably reflects stimulation of the vagal nuclei in the medulla. Morphine may also exert a direct depressant effect on the sinoatrial node and acts to slow conduction of cardiac impulses through the atrioventricular node. These actions, may, in part, explain decreased vulnerability to ventricular fibrillation in the presence of morphine. Administration of opioids (morphine, fentanyl) in the preoperative medication or before the induction of anesthesia tends to slow heart rate during exposure to volatile anesthetics with or without surgical stimulation.25
Opioid-induced histamine release and associated hypotension are variable in both incidence and severity. The magnitude of morphine-induced histamine release and subsequent decrease in systemic blood pressure can be minimized by (a) limiting the rate of morphine infusion to 5 mg per minute intravenously (IV), (b) maintaining the patient in a supine to slightly head-down position, and (c) optimizing intravascular fluid volume. Conversely, administration of morphine, 1 mg/kg IV, over a 10-minute period produces substantial increases in the plasma concentrations of histamine that are paralleled by significant decreases in systemic blood pressure and systemic vascular resistance (Fig. 7-4).26 It is important to recognize, however, that not all patients respond to this rate of morphine infusion with the release of histamine, emphasizing the individual variability associated with the administration of this drug. In contrast to morphine, the infusion of fentanyl 50 µg/kg IV over a 10-minute period does not cause release of histamine in any patient (see Fig. 7-4). Pretreatment of patients with H1 and H2 receptor antagonists does not alter release of histamine evoked by morphine but does prevent changes in systemic blood pressure and systemic vascular resistance.27

Morphine does not sensitize the heart to catecholamines or otherwise predispose to cardiac dysrhythmias as long as hypercarbia or arterial hypoxemia does not result from ventilatory depression. Tachycardia and hypertension that occur during anesthesia with morphine are not pharmacologic effects of the opioid but rather are responses to painful surgical stimulation that are not suppressed by morphine. Both the sympathetic nervous system and the renin-angiotensin axis contribute to these cardiovascular responses. Large doses of morphine or other opioid agonists may decrease the likelihood that tachycardia and hypertension will occur in response to painful stimulation, but once this response has occurred, administration of additional opioid is unlikely to be effective.
During anesthesia, however, opioids are commonly administered with inhaled or IV anesthetics to ensure amnesia. The combination of an opioid agonist such as morphine or fentanyl with nitrous oxide results in cardiovascular depression (decreased cardiac output and systemic blood pressure plus increased cardiac filling pressures), which does not occur when either drug is administered alone.28 Likewise, decreases in systemic vascular resistance and systemic blood pressure may accompany the combination of an opioid and a benzodiazepine, whereas these effects do not accompany the administration of either drug alone (Fig. 7-5).29

Opioids have been increasingly recognized as playing a role in protecting the myocardium from ischemia. Through several mechanisms, most prominently though σ and κ receptors, opioids enhance the resistance of the myocardium to oxidative and ischemic stresses. Mitochondrial adenosine triphosphate (ATP)–regulated potassium channels (KATP) appear to be central to this signaling pathway.30
Ventilation
All opioid agonists produce dose-dependent and gender-specific depression of ventilation, primarily through an agonist effect at mu2 receptors leading to a direct depressant effect on brainstem ventilation centers.8 Because analgesic and ventilatory effects of opioids occur by similar mechanisms, it is assumed that equianalgesic doses of all opioids will produce some degree of ventilatory depression and reversal of ventilatory depression with an opioid antagonist always involves some reversal of analgesia. Opioid-induced depression of ventilation is characterized by decreased responsiveness of these ventilation centers to carbon dioxide as reflected by an increase in the resting PaCO2 and displacement of the carbon dioxide response curve to the right. Opioid agonists also interfere with pontine and medullary ventilatory centers that regulate the rhythm of breathing, leading to prolonged pauses between breaths and periodic breathing. It is possible that opioid agonists diminish sensitivity to carbon dioxide by decreasing the release of acetylcholine from neurons in the area of the medullary ventilatory center in response to hypercarbia. In this regard, physostigmine, which increases CNS levels of acetylcholine, may antagonize depression of ventilation but not analgesia produced by morphine.
Depression of ventilation produced by opioid agonists is rapid and persists for several hours, as demonstrated by decreased ventilatory responses to carbon dioxide. High doses of opioids may result in apnea, but the patient remains conscious and able to initiate a breath if asked to do so. Death from an opioid overdose is almost invariably due to depression of ventilation.
Clinically, depression of ventilation produced by opioids manifests as a decreased frequency of breathing that is often accompanied by a compensatory increase in tidal volume. The incompleteness of this compensatory increase in tidal volume is evidenced by predictable increases in the PaCO2. Many factors influence the magnitude and duration of depression of ventilation produced by opioid agonists. For example, advanced age and the occurrence of natural sleep increase the ventilatory depressant effects of opioids. Conversely, pain from surgical stimulation counteracts depression of ventilation produced by opioids. Likewise, the analgesic effect of opioids slows breathing that has been rapid and shallow due to pain.
Opioids produce dose-dependent depression of ciliary activity in the airways. Increases in airway resistance after administration of an opioid are probably due to a direct effect on bronchial smooth muscle and an indirect action due to release of histamine.
Cough Suppression
Opioids depress cough by effects on the medullary cough centers that are distinct from the effects of opioids on ventilation. The greatest cough suppression occurs with opioids that have bulky substitutions at the number 3 carbon position (codeine). One useful property of dextrorotatory isomers (such as dextromethorphan) is that they can suppress cough but do not produce analgesia or depression of ventilation. Thus, in some cases, opioids can be safely sold over-the-counter.
Central Nervous System
In the absence of hypoventilation, opioids decrease cerebral blood flow and possibly intracranial pressure (ICP). These drugs must be used with caution in patients with head injury because of their (a) associated effects on wakefulness, (b) production of miosis, and (c) depression of ventilation with associated increases in ICP if the PaCO2 becomes increased. Furthermore, head injury may impair the integrity of the blood–brain barrier, with resultant increased sensitivity to opioids.
The effect of morphine on the electroencephalogram (EEG) resembles changes associated with sleep. For example, there is replacement of rapid α waves by slower δ waves. Recording of the EEG fails to reveal any evidence of seizure activity after administration of large doses of opioids (see the section “Fentanyl”). Opioids do not alter the responses to neuromuscular blocking drugs. Skeletal muscle rigidity, especially of the thoracic and abdominal muscles, is common when large doses of opioid agonists are administered rapidly and intravenously.31 Clonic skeletal muscle activity (myoclonus) occurring during administration of opioids may resemble grand mal seizures, but the EEG does not reflect seizure activity. Skeletal muscle rigidity may be related to actions at opioid receptors and involve interactions with dopaminergic and γ-aminobutyric acid–responsive neurons.
Miosis is due to an excitatory action of opioids on the autonomic nervous system component of the Edinger-Westphal nucleus of the oculomotor nerve. Tolerance to the miotic effect of morphine is not prominent. Miosis can be antagonized by atropine, and profound arterial hypoxemia in the presence of morphine can still result in mydriasis.
Rigidity
Rapid IV administration of large doses of an opioid (particularly fentanyl and its derivatives as used in cardiac surgery) can lead to generalized skeletal muscle rigidity. This can be severe enough to interfere with manual ventilation. Although generally termed chest wall rigidity, evidence supports the conclusion that the majority of resistance to ventilation is due to laryngeal musculature contraction. Inhibition of striatal release of γ-aminobutyric acid and increased dopamine production are the likely explanations for opioid-induced increased skeletal muscle tone.32 The reported incidence of difficult ventilation after a moderate dose of sufentanil ranges from 84% to 100%.33 Treatment is muscle relaxation with neuromuscular blocking drugs or opioid antagonism with naloxone.
Sedation
Postoperative titration of morphine frequently induces sedation that precedes the onset of analgesia.34 The usual recommendation for morphine titration includes a short interval between boluses (5 to 7 minutes) to allow evaluation of its clinical effect. Sedation occurs in up to 60% of patients during morphine titration and represents a common reason to discontinue morphine titration for postoperative analgesia. The assumption that sleep occurs when pain is relieved is not necessarily accurate and morphine-induced sedation should not be considered as an indicator of appropriate analgesia during IV morphine titration.
Biliary Tract
Opioids can cause spasm of biliary smooth muscle, resulting in increases in biliary pressure that may be associated with epigastric distress or biliary colic. This pain may be confused with angina pectoris. Naloxone will relieve pain caused by biliary spasm but not myocardial ischemia. Conversely, nitroglycerin will relieve pain due to either biliary spasm or myocardial ischemia. Equal analgesic doses of fentanyl, morphine, meperidine, and pentazocine increase common bile duct pressure 99%, 53%, 61%, and 15% above predrug levels, respectively.35 During surgery, opioid-induced spasm of the sphincter of Oddi may appear radiologically as a sharp constriction at the distal end of the common bile duct and be misinterpreted as a common bile duct stone. It may be necessary to reverse opioid-induced biliary smooth muscle spasm with naloxone so as to correctly interpret the cholangiogram. Glucagon, 2 mg IV, also reverses opioid-induced biliary smooth muscle spasm and, unlike naloxone, does not antagonize the analgesic effects of the opioid.36 However, biliary muscle spasm does not occur in most patients who receive opioids. Indeed, the incidence of spasm of the sphincter of Oddi is about 3% in patients receiving fentanyl as a supplement to inhaled anesthetics.37
Contraction of the smooth muscles of the pancreatic ducts is probably responsible for increases in plasma amylase and lipase concentrations that may be present after the administration of morphine. Such increases may confuse the diagnosis when acute pancreatitis is a possibility.
Gastrointestinal Tract
Commonly used opioids such as morphine, meperidine, and fentanyl can produce spasm of the gastrointestinal smooth muscles, resulting in a variety of side effects including constipation, biliary colic, and delayed gastric emptying.
Morphine decreases the propulsive peristaltic contractions of the small and large intestines and enhances the tone of the pyloric sphincter, ileocecal valve, and anal sphincter. The delayed passage of intestinal contents through the colon allows increased absorption of water. As a result, constipation often accompanies therapy with opioids and may become a debilitating problem in patients who require chronic opioid therapy, as little tolerance develops to this effect. Of interest, opium was used to treat diarrhea before its use as an analgesic was popularized.
Increased biliary pressure occurs when the gallbladder contracts against a closed or narrowed sphincter of Oddi. Passage of gastric contents into the proximal duodenum is delayed because there is increased tone at the gastroduodenal junction. In this regard, preoperative medication that includes an opioid could slow gastric emptying (potentially increase the risk of aspiration) or delay the absorption of orally administered drugs. All these effects may be reversed or prevented by a peripheral-acting opioid antagonist (see the section “Methylnaltrexone”).
Nausea and Vomiting
Opioid-induced nausea and vomiting are caused by direct stimulation of the chemoreceptor trigger zone in the floor of the fourth ventricle. This may reflect the role of opioid agonists as partial dopamine agonists at dopamine receptors in the chemoreceptor trigger zone. Indeed, apomorphine is a profound emetic and is also the most potent of the opioids at dopamine receptors. Stimulation of dopamine receptors as a mechanism for opioid-induced nausea and vomiting is consistent with the antiemetic efficacy of butyrophenones and phenothiazines. Morphine may also cause nausea and vomiting by increasing gastrointestinal secretions and delaying passage of intestinal contents toward the colon.
Morphine depresses the vomiting center in the medulla. As a result, IV administration of morphine produces less nausea and vomiting than the intramuscular (IM) administration of morphine, presumably because opioid administered IV reaches the vomiting center as rapidly as it reaches the chemoreceptor trigger zone. Nausea and vomiting are relatively uncommon in recumbent patients given morphine, suggesting that a vestibular component may contribute to opioid-induced nausea and vomiting.
Genitourinary System
Morphine can increase the tone and peristaltic activity of the ureter. In contrast to similar effects on biliary tract smooth muscle, the same opioid-induced effects on the ureter can be reversed by an anticholinergic drug such as atropine. Urinary urgency is produced by opioid-induced augmentation of detrusor muscle tone, but, at the same time, the tone of the urinary sphincter is enhanced, making voiding difficult.
Antidiuresis that accompanies administration of morphine to animals has been attributed to opioid-induced release of arginine vasopressin hormone (antidiuretic hormone). In humans, however, administration of morphine in the absence of painful surgical stimulation does not evoke the release of this hormone.38 Furthermore, when morphine is administered in the presence of an adequate intravascular fluid volume, there is no change in urine output.
Cutaneous Changes
Morphine causes cutaneous blood vessels to dilate. The skin of the face, neck, and upper chest frequently becomes flushed and warm. These changes in cutaneous circulation are in part caused by the release of histamine. Histamine release probably accounts for urticaria and erythema commonly seen at the morphine injection site. In addition, morphine-induced histamine release probably accounts for conjunctival erythema and pruritus. Localized cutaneous evidence of histamine release, especially along the vein into which morphine is injected, does not represent an allergic reaction.
Placental Transfer
Opioids are readily transported across the placenta. Therefore, depression of the neonate can occur as a consequence of administration of opioids to the mother during labor. In this regard, maternal administration of morphine may produce greater neonatal depression than meperidine does.39 This may reflect immaturity of the neonate’s blood–brain barrier. Chronic maternal use of an opioid can result in the development of physical dependence in the fetus. Subsequent administration of naloxone to the neonate can precipitate neonatal abstinence syndrome.
Drug Interactions
The ventilatory depressant effects of some opioids may be exaggerated by amphetamines, phenothiazines, monoamine oxidase inhibitors, and tricyclic antidepressants. For example, patients receiving monoamine oxidase inhibitors may experience exaggerated CNS depression and hyperpyrexia after administration of an opioid agonist, especially meperidine. This exaggerated response may reflect alterations in the rate or pathway of metabolism of the opioid. Sympathomimetic drugs appear to enhance analgesia produced by opioids. The cholinergic nervous system seems to be a positive modulator of opioid-induced analgesia in that physostigmine enhances and atropine antagonizes analgesia.
Hormonal Changes
Prolonged opioid therapy may influence the hypothalamic-pituitary-adrenal axis and the hypothalamic-pituitary-gonadal axis, leading to endocrine and immune effects.40,41 Morphine may cause a progressive decrease in plasma cortisol concentrations. The main effects of opioids on the hypothalamic-pituitary-gonadal axis involve modulation of hormone release including increased prolactin and decreased luteinizing hormone, follicle-stimulating hormone, testosterone, and estrogen concentrations.
Overdose
The principal manifestation of opioid overdose is depression of ventilation manifesting as a slow breathing frequency, which may progress to apnea. Pupils are symmetric and miotic unless severe arterial hypoxemia is present, which results in mydriasis. Skeletal muscles are flaccid, and upper airway obstruction may occur. Pulmonary edema commonly occurs, but the mechanism is not known. Hypotension and seizures develop if arterial hypoxemia persists. The triad of miosis, hypoventilation, and coma should suggest overdose with an opioid. Treatment of opioid overdose is mechanical ventilation of the patient’s lungs with oxygen and administration of an opioid antagonist such as naloxone. Administration of an opioid antagonist to treat opioid overdose may precipitate acute withdrawal in dependent patients.
Provocation of Coughing
Paradoxically, preinduction administration of fentanyl, sufentanil, or alfentanil may be associated with significant reflex coughing.42 The exact cause of opioid-induced cough is unclear but is thought to be due to imbalance between sympathetic and vagal innervation of the airways and/or stimulation of juxtacapillary irritant receptors.43 Morphine and hydromorphone do not appear to cause this reaction.
Pharmacodynamic Tolerance and Physical Dependence
Pharmacodynamic tolerance and physical dependence with repeated opioid administration are characteristics of all opioid agonists and are among the major limitations of their clinical use. Cross-tolerance develops between all the opioids. Tolerance can occur without physical dependence, but the reverse does not seem to occur.
Tolerance is the development of the requirement for increased doses of a drug (in this case, an opioid agonist) to achieve the same effect previously achieved with a lower dose. Such acquired tolerance usually takes 2 to 3 weeks to develop with analgesic doses of morphine, although acute tolerance can develop much more quickly with highly potent opioids.44 Tolerance develops to analgesic, euphoric, sedative, depression of ventilation, and emetic effects of opioids but not to their effects on miosis and bowel motility. The potential for physical dependence depends on the agonist effect of opioids. Indeed, physical dependence does not occur with opioid antagonists and is less likely with opioid agonist–antagonists. When opioid agonist actions predominate, there often develops, with repeated use, both psychological and physiologic need for the drug.
Physical dependence on morphine usually requires about 25 days to develop but may occur sooner in emotionally unstable persons. Some degree of physical dependence, however, occurs after only 48 hours of continuous medication. When physical dependence is established, discontinuation of the opioid agonist produces a typical withdrawal abstinence syndrome (Table 7-3).45 Initial symptoms of withdrawal include yawning, diaphoresis, lacrimation, or coryza. Insomnia and restlessness are prominent. Abdominal cramps, nausea, vomiting, and diarrhea reach their peak in 72 hours and then decline over the next 7 to 10 days. During withdrawal, tolerance to morphine is rapidly lost, and the syndrome can be terminated by a modest dose of opioid agonist. The longer the period of abstinence, the smaller the dose of opioid agonist that will be required.

Pharmacodynamic tolerance has been related to neurologic changes that take place after long-term exposure to the opioid.45 The classic explanation for tolerance to a receptor agonist involved changes occurring at the level of the receptors and involve receptor desensitization. Opioid receptors on the cell membrane surfaces become gradually desensitized by reduced transcription and subsequent decreases in the absolute numbers of opioid receptors (downregulation). A second mechanism proposed to explain pharmacodynamic tolerance involves upregulation of the cAMP system. Acutely, opioids inhibit functional activity of cAMP pathways by blocking adenylate cyclase, the enzyme that catalyzes the synthesis of cAMP. Long-term opioid exposure is associated with gradual recovery of cAMP pathways and tolerance develops. Increased synthesis of cAMP may be responsible for physical dependence and physiologic changes associated with withdrawal. Upregulation of cAMP has been most clearly demonstrated in the locus ceruleus of the brain. Clonidine, a centrally acting α2-adrenergic agonist that diminishes transmission in sympathetic pathways in the CNS, is an effective drug in suppressing withdrawal signs in persons who are physically dependent on opioids. Tolerance is not due to enzyme induction, because no increase in the rate of metabolism of opioid agonists occurs.
Long-term pharmacodynamic tolerance characterized by opioid insensitivity may persist for months or years in some individuals and most likely represents persistent neural adaptation.45 In this regard, NMDA glutamate receptors are important in the development of opioid tolerance and increased pain sensitivity. Prolonged exposure to opioids activates NMDA receptors via second messenger mechanisms and also downregulates spinal glutamate transporters. The resultant high synaptic concentrations of glutamate and NMDA receptor activation contribute to opioid tolerance and abnormal pain sensitivity (pronociceptive or sensitization process). The observation that treatment with small doses of ketamine (an NMDA receptor antagonist) abolishes the acute opioid tolerance seen with remifentanil supports this hypothesis.46
Opioid Agonists
Opioid agonists include but are not limited to morphine, meperidine, fentanyl, sufentanil, alfentanil, and remifentanil (see Table 7-1).47 The most notable feature of the clinical use of opioids is the extraordinary variation in dose requirements for effective treatment of pain.48 This interindividual variation emphasizes that usual doses of opioids may produce inadequate or excessive opioid effects. Opioid rotation may be useful when dose escalation is not effective in treating pain.
Morphine
Isolated in 1806 and named after Morpheus, the Greek god of dreams, morphine is the prototype opioid agonist to which all other opioids are compared. In humans, morphine produces analgesia, euphoria, sedation, and a diminished ability to concentrate. Other sensations include nausea, a feeling of body warmth, heaviness of the extremities, dryness of the mouth, and pruritus, especially in the cutaneous areas around the nose. The cause of pain persists, but even low doses of morphine increase the threshold to pain and modify the perception of noxious stimulation such that it is no longer experienced as pain. Continuous, dull pain is relieved by morphine more effectively than is sharp, intermittent pain. In contrast to nonopioid analgesics, morphine is effective against pain arising from the viscera as well as from skeletal muscles, joints, and integumental structures. Analgesia is most prominent when morphine is administered before the painful stimulus occurs.49 In the absence of pain, however, morphine may produce dysphoria rather than euphoria.
Pharmacokinetics
Morphine is well absorbed after IM administration, with onset of effect in 15 to 30 minutes and a peak effect in 45 to 90 minutes. The clinical duration of action is about 4 hours. Morphine can be administered orally for treatment of chronic pain recognizing that absorption from the gastrointestinal is limited by significant first-pass metabolism in the liver, which limits the bioavailability of an orally administered dose to approximately 25% (1 mg of IV morphine ~4 mg of oral morphine). Morphine is usually administered IV in the perioperative period, thus eliminating the unpredictable influence of drug absorption. The peak effect (equilibration time between the blood and brain) after IV administration of morphine is delayed compared with opioids such as fentanyl and alfentanil, requiring about 15 to 30 minutes (Table 7-4). Morphine inhaled as an aerosol from a nebulizer may act on afferent nerve pathways in the airways to relieve dyspnea as associated with lung cancer and associated pleural effusion.50 However, profound depression of ventilation may follow aerosol administration of morphine.51 The onset and duration of the analgesic effects of morphine are similar after IV administration or inhalation via a pulmonary drug delivery system that produces a fine aerosol.52

Plasma morphine concentrations after rapid IV injections do not correlate closely with the drug’s pharmacologic activity, likely due to the delay in transit of morphine across the blood–brain barrier. Cerebrospinal fluid (CSF) concentrations of morphine peak 15 to 30 minutes after IV injection and decay more slowly than plasma concentrations (Fig. 7-6).53 As a result, the analgesic and ventilatory depressant effects of morphine may not be evident during the initial high plasma concentrations after IV administration of the opioid. Likewise, these same drug effects persist despite decreasing plasma concentrations of morphine. Moderate analgesia probably requires maintenance of plasma morphine concentrations of at least 0.05 µg/mL.54

Only a small amount of administered morphine gains access to the CNS. For example, it is estimated that less than 0.1% of morphine that is administered IV has entered the CNS at the time of peak plasma concentrations. Reasons for poor penetration of morphine into the CNS include (a) relatively poor lipid solubility, (b) high degree of ionization at physiologic pH, (c) protein binding, and (d) rapid conjugation with glucuronic acid. Alkalinization of the blood, as produced by hyperventilation of the patient’s lungs, will increase the nonionized fraction of morphine and thus enhance its passage into the CNS. Nevertheless, respiratory acidosis, which decreases the nonionized fraction of morphine, results in higher plasma and brain concentrations of morphine than are present during normocarbia (Fig. 7-7).55 This suggests that carbon dioxide–induced increases in cerebral blood flow and enhanced delivery of morphine to the brain are more important than the fraction of drug that exists in either the ionized or nonionized fraction. In contrast to the CNS, morphine accumulates rapidly in the kidneys, liver, and skeletal muscles. Morphine, unlike fentanyl, does not undergo significant first-pass uptake into the lungs.56

Metabolism
Metabolism of morphine is primarily conjugation with glucuronic acid in hepatic and extrahepatic sites, especially the kidneys. About 75% to 85% of a dose of morphine appears as morphine-3-glucuronide, and 5% to 10% as morphine-6-glucuronide (a ratio of 9:1). Morphine-3-glucuronide is detectable in the plasma within 1 minute after IV injection, and its concentration exceeds that of unchanged drug by almost 10-fold within 90 minutes (Fig. 7-8).53 An estimated 5% of morphine is demethylated to normorphine, and a small amount of codeine may also be formed. Metabolites of morphine are eliminated principally in the urine, with only 7% to 10% undergoing biliary excretion. Morphine-3-glucuronide is detectable in the urine for up to 72 hours after the administration of morphine. A small fraction (1% to 2%) of injected morphine is recovered unchanged in the urine.

Morphine-3-glucuronide is pharmacologically inactive, whereas morphine-6-glucuronide produces analgesia and depression of ventilation via its agonist actions at µ receptors.57 In fact, the ventilatory response to carbon dioxide is impacted similarly by morphine and morphine-6-glucuronide (Fig. 7-9).58 The duration of action of morphine-6-glucuronide is greater than that of morphine, and it is possible that the majority of analgesic activity attributed to morphine is actually due to morphine-6-glucuronide, especially with long-term administration of morphine.59 Morphine and morphine-6-glucuronide bind to µ opioid receptors with comparable affinity, whereas the analgesic potency of morphine-6-glucuronide is 650-fold higher than morphine.60

Renal metabolism makes a significant contribution to the total metabolism of morphine, which offers a possible explanation for the absence of any decrease in systemic clearance of morphine in patients with hepatic cirrhosis or during the anhepatic phase of orthotopic liver transplantation.61
Elimination of morphine glucuronides may be impaired in patients with renal failure, causing an accumulation of metabolites and unexpected ventilatory depressant effects of small doses of opioids (Fig. 7-10).62 Indeed, prolonged depression of ventilation (>7 days) has been observed in patients in renal failure after administration of morphine.63 Formation of glucuronide conjugates may be impaired by monoamine oxidase inhibitors, which is consistent with exaggerated effects of morphine when administered to patients being treated with these drugs.

Elimination Half-Time
After IV administration of morphine, the elimination of morphine-3-glucuronide is somewhat longer than for morphine (see Table 7-4 and Fig. 7-8).53 The decrease in the plasma concentration of morphine after initial distribution of the drug is principally due to metabolism because only a small amount of unchanged opioid is excreted in the urine. Plasma morphine concentrations are higher in the elderly than in young adults (Fig. 7-11).54 In the first 4 days of life, the clearance of morphine is decreased and its elimination half-time is prolonged compared with that found in older infants.64 This is consistent with the observation that neonates are more sensitive than older children to the respiratory depressant effects of morphine. Patients with renal failure exhibit higher plasma and CSF concentrations of morphine and morphine metabolites than do normal patients, reflecting a smaller volume of distribution (Vd).65 Possible accumulation of morphine-6-glucuronide suggests the need for caution when administering morphine to patients with renal dysfunction. Concentrations of morphine in the colostrum of parturients receiving patient-controlled analgesia with morphine are low and it is unlikely that significant amounts of drug will be transferred to the breast-fed neonate.66

Gender
Gender may affect opioid analgesia but the direction and magnitude of these differences depend on many interacting variables including the opioid used.67 Morphine exhibits greater analgesic potency and slower speed of offset in women than men.68 This observation is consistent with higher postoperative opioid consumption in men compared with women. Likewise, morphine decreases the slope of the ventilatory response to carbon dioxide in women, whereas in men, there was no significant effect.69 Morphine has no demonstrated effect on the apneic threshold in women but causes an increase in men. Hypoxic sensitivity is decreased by morphine in women but not men.
Side Effects
Side effects described for morphine are also characteristic of other opioid agonists, although the incidence and magnitude may vary.
Meperidine
First synthesized in 1939, meperidine (also referred to as pethidine) is a synthetic opioid agonist at µ and κ opioid receptors and is derived from phenylepiperidine (Fig. 7-2). There are several analogues of meperidine, including fentanyl, sufentanil, alfentanil, and remifentanil. Meperidine shares several structural features that are present in local anesthetics including a tertiary amine, an ester group, and a lipophilic phenyl group. Indeed, meperidine administered intrathecally blocks sodium channels to a degree comparable with lidocaine. Structurally, meperidine is similar to atropine, and it possesses a mild atropine-like antispasmodic effect on smooth muscle.
Pharmacokinetics
Meperidine is about one-tenth as potent as morphine. The duration of action of meperidine is 2 to 4 hours, making it a shorter acting opioid agonist than morphine. In equianalgesic doses, meperidine produces equivalent sedation, euphoria, nausea, vomiting, and depression of ventilation to morphine. Meperidine is absorbed from the gastrointestinal tract, but extensive first-pass hepatic metabolism (up to 80%) limits its oral usefulness.
Metabolism
Hepatic metabolism of meperidine is extensive, with about 90% of the drug initially undergoing demethylation to normeperidine and hydrolysis to meperidinic acid.70 Normeperidine subsequently undergoes hydrolysis to normeperidinic acid. Urinary excretion is the principal elimination route and is pH dependent. For example, if the urinary pH is <5, as much as 25% of meperidine is excreted unchanged. Indeed, acidification of the urine can be considered in an attempt to speed elimination of meperidine. Decreased renal function can predispose to accumulation of normeperidine.
Normeperidine has an elimination half-time of 15 hours (35 hours in patients in renal failure) and can be detected in urine for as long as 3 days after administration of meperidine. This metabolite is about one-half as active as meperidine as an analgesic. In addition, normeperidine produces CNS stimulation. Normeperidine toxicity manifesting as myoclonus and seizures is most likely during prolonged administration of meperidine as during patient-controlled analgesia, especially in the presence of impaired renal function.70 Normeperidine may also be important in meperidine-induced delirium (confusion, hallucinations), which has been observed in patients receiving the drug for longer than 3 days, corresponding to accumulation of this active metabolite.
Elimination Half-Time
The elimination half-time of meperidine is 3 to 5 hours (see Table 7-4). Because clearance of meperidine primarily depends on hepatic metabolism, it is possible that large doses of opioid would saturate enzyme systems and result in prolonged elimination half-times. Nevertheless, elimination half-time is not altered by doses of meperidine up to 5 mg/kg IV. About 60% of meperidine is bound to plasma proteins. Elderly patients manifest decreased plasma protein binding of meperidine, resulting in increased plasma concentrations of free drug and an apparent increased sensitivity to the opioid. The increased tolerance of alcoholics to meperidine and other opioids presumably reflects an increased volume of distribution, resulting in lower plasma concentrations of meperidine for a given dose.
Clinical Uses
The clinical use of meperidine has declined greatly in recent years. Meperidine is the only opioid considered adequate for surgery when administered intrathecally, owing to its ability to block sodium channels in a way similar to local anesthetics in addition to its µ-mediated opioid acitivity.71 An IM injection of meperidine for postoperative analgesia results in peak plasma concentrations that vary three- to fivefold as well as a time required to achieve peak concentrations that varies three- to sevenfold among patients.72 The minimum analgesic plasma concentration of meperidine is highly variable among patients; however, in the same patient, differences in concentrations as small as 0.05 µg/mL can represent a margin between no relief and complete analgesia. A plasma meperidine concentration of 0.7 µg/mL would be expected to provide postoperative analgesia in about 95% of patients.73 Normeperidine toxicity has been described in patients receiving meperidine for patient-controlled analgesia.70 Therefore, because there are other effective agents, patient-controlled analgesia with meperidine cannot be recommended.
Meperidine may be effective in suppressing postoperative shivering that may result in detrimental increases in metabolic oxygen consumption. The antishivering effects of meperidine may reflect stimulation of κ receptors (estimated to represent 10% of its activity) and a drug-induced decrease in the shivering threshold (not present with alfentanil, clonidine, propofol, or volatile anesthetics).74–76 In addition, meperidine is a potent agonist at α2 receptors, which might contribute to antishivering effects.77 Indeed, clonidine is even more effective than meperidine in reducing postoperative shivering. Butorphanol (a κ receptor agonist-antagonist) stops shivering more effectively than opioids with a predominant µ opioid receptor agonist effect. Evidence for a role of κ receptors in the antishivering effects of meperidine and butorphanol is the failure of naloxone to completely inhibit this drug-induced effect.
Unlike morphine, meperidine is not useful for the treatment of diarrhea and is not an effective cough suppressant. During bronchoscopy, the relative lack of antitussive activity of meperidine makes this opioid less useful. Meperidine is not used in high doses because of significant negative cardiac inotropic effects plus histamine release in a substantial number of patients.78
Side Effects
The side effects of meperidine generally resemble those described for morphine. Meperidine, in contrast to morphine, rarely causes bradycardia but instead may increase heart rate, reflecting its modest atropine-like qualities. Large doses of meperidine result in decreases in myocardial contractility, which, among opioids, is unique for this drug. Delirium and seizures, when they occur, presumably reflect accumulation of normeperidine, which has stimulating effects on the CNS.
Serotonin syndrome (autonomic instability with hypertension, tachycardia, diaphoresis, hyperthermia, behavioral changes including confusion and agitation, and neuromuscular changes manifesting as hyperreflexia) occurs when drugs capable of increasing serotonin administration are administered. In severe cases, coma, seizures, coagulopathy, and metabolic acidosis may develop. Administration of meperidine to patients receiving antidepressant drugs (monoamine oxidase inhibitors, fluoxetine) may elicit this syndrome.79
Meperidine readily impairs ventilation and may be even more of a ventilatory depressant than morphine. This opioid promptly crosses the placenta, and concentrations of meperidine in umbilical cord blood at birth may exceed maternal plasma concentrations.39 Meperidine may produce less constipation and urinary retention than morphine. After equal analgesic doses, biliary tract spasm is less after meperidine injection than after morphine injection but greater than that caused by codeine.35 Meperidine does not cause miosis but rather tends to cause mydriasis, reflecting its modest atropine-like actions. A dry mouth and an increase in heart rate are further evidence of the atropine-like effects of meperidine. Transient neurologic symptoms have been described following the administration of intrathecal meperidine for surgical anesthesia.80
The pattern of withdrawal symptoms after abrupt discontinuation of meperidine differs from that of morphine in that there are few autonomic nervous system effects. In addition, symptoms of withdrawal develop more rapidly and are of a shorter duration compared with those of morphine.
Fentanyl
Fentanyl is a phenylpiperidine-derivative synthetic opioid agonist that is structurally related to meperidine (see Fig. 7-2). As an analgesic, fentanyl is 75 to 125 times more potent than morphine. It was first synthesized by Janssen Pharmaceutica in 1960 during an assay of meperidine derivatives and subsequently released as the citrate salt under the trade name Sublimaze.81
Pharmacokinetics
A single dose of fentanyl administered IV has a more rapid onset and shorter duration of action than morphine. Despite the clinical impression that fentanyl produces a rapid onset, there is a distinct time lag between the peak plasma fentanyl concentration and peak slowing on the EEG. This delay reflects the effect-site equilibration time between blood and the brain for fentanyl, which is 6.4 minutes. The greater potency and more rapid onset of action reflect the greater lipid solubility of fentanyl compared with that of morphine, which facilitates its passage across the blood–brain barrier. Consequently, plasma concentrations of fentanyl (unlike morphine) correlate well with CSF concentrations. Likewise, the short duration of action of a single dose of fentanyl reflects its rapid redistribution to inactive tissue sites such as fat and skeletal muscles, with an associated decrease in the plasma concentration of the drug (Fig. 7-12).82

The lungs also serve as a large inactive storage site, with an estimated 75% of the initial fentanyl dose undergoing first-pass pulmonary uptake.56 This nonrespiratory function of the lungs limits the initial amount of drug that reaches the systemic circulation and may play an important role in determining the pharmacokinetic profile of fentanyl. When multiple IV doses of fentanyl are administered or when there is continuous infusion of the drug, progressive saturation of these inactive tissue sites occurs. As a result, the plasma concentration of fentanyl does not decrease rapidly, and the duration of analgesia, as well as depression of ventilation, may be prolonged. Cardiopulmonary bypass causes clinically insignificant effects on the pharmacokinetics of fentanyl despite associated hemodilution, hypothermia, nonphysiologic blood flow and cardiopulmonary bypass–induced systemic inflammatory responses.83
Metabolism
Fentanyl is extensively metabolized by N-demethylation, producing norfentanyl, hydroxyproprionyl-fentanyl, and hydroxyproprionyl-norfentanyl. Norfentanyl is structurally similar to normeperidine and is the principal metabolite of fentanyl in humans. It is excreted by the kidneys and can be detected in the urine for 72 hours after a single IV dose of fentanyl. Less than 10% of fentanyl is excreted unchanged in the urine. The pharmacologic activity of fentanyl metabolites is believed to be minimal.84 Fentanyl is a substrate for hepatic P450 enzymes (CYP3A) and is susceptible to drug interactions that reflect interference with enzyme activity (less likely than with alfentanil).85
Elimination Half-Time
Despite the clinical impression that fentanyl has a short duration of action, its elimination half-time is longer than that for morphine (see Table 7-4). This longer elimination half-time reflects a larger Vd of fentanyl because clearance of both opioids is similar (see Table 7-4). The larger Vd of fentanyl is due to its greater lipid solubility and thus more rapid passage into tissues compared with the less lipid-soluble morphine. After an IV bolus, fentanyl distributes rapidly from the plasma to highly vascular tissues (brain, lungs, heart). More than 80% of the injected dose leaves the plasma in <5 minutes. The plasma concentrations of fentanyl are maintained by slow reuptake from inactive tissue sites, which accounts for persistent drug effects that parallel the prolonged elimination half-time. In animals, the elimination half-time, Vd, and clearance of fentanyl are independent of the dose of opioid between 6.4 and 640 µg/kg IV.86
A prolonged elimination half-time for fentanyl in elderly patients is due to decreased clearance of the opioid because Vd is not changed in comparison with younger adults.87 This change may reflect age-related decreases in hepatic blood flow, microsomal enzyme activity, or albumin production, as fentanyl is highly bound (79% to 87%) to protein. For these reasons, it is likely that a given dose of fentanyl will be effective for a longer period of time in elderly patients than in younger patients. A prolonged elimination half-time of fentanyl has also been observed in patients undergoing abdominal aortic surgery requiring infrarenal aortic cross-clamping.88 Somewhat surprising, however, is the failure of hepatic cirrhosis to prolong significantly the elimination half-time of fentanyl.89
Context-Sensitive Half-Time
As the duration of continuous infusion of fentanyl increases beyond about 2 hours, the context-sensitive half-time of this opioid becomes greater than sufentanil (Fig. 7-13).3,90 This reflects saturation of inactive tissue sites with fentanyl during prolonged infusions and return of the opioid from peripheral compartments to the plasma. This tissue reservoir of fentanyl replaces fentanyl eliminated by hepatic metabolism so as to slow the rate of decrease in the plasma concentration of fentanyl when the infusion is discontinued.

Cardiopulmonary Bypass
All opioids show a decrease in plasma concentration with initiation of cardiopulmonary bypass.91 The degree of this decrease is greater with fentanyl because a significant proportion of the drug adheres to the surface of the cardiopulmonary bypass circuit. The decrease is least with opioids that have a large Vd such that the addition of prime volume is less important. In this respect, sufentanil and alfentanil may provide more stable plasma concentrations during cardiopulmonary bypass. Elimination of fentanyl and alfentanil has been shown to be prolonged by cardiopulmonary bypass.
Clinical Uses
Fentanyl is administered clinically in a wide range of doses. For example, low doses of fentanyl, 1 to 2 µg/kg IV, are injected to provide analgesia. Fentanyl, 2 to 20 µg/kg IV, may be administered as an adjuvant to inhaled anesthetics in an attempt to blunt circulatory responses to (a) direct laryngoscopy for intubation of the trachea, or (b) sudden changes in the level of surgical stimulation. Timing of the IV injection of fentanyl to prevent or treat such responses should consider the effect-site equilibration time, which for fentanyl is prolonged compared with alfentanil and remifentanil. Injection of an opioid such as fentanyl before painful surgical stimulation may decrease the subsequent amount of opioid required in the postoperative period to provide analgesia.49 Administration of fentanyl 1.5 or 3 µg/kg IV 5 minutes before induction of anesthesia decreases the subsequent doses of isoflurane or desflurane with 60% nitrous oxide needed to block the sympathetic nervous system response to surgical stimulation (Fig. 7-14).92 Large doses of fentanyl, 50 to 150 µg/kg IV, have been used alone to produce surgical anesthesia. Large doses of fentanyl as the sole anesthetic have the advantage of stable hemodynamics due principally to the (a) lack of direct myocardial depressant effects, (b) absence of histamine release, and (c) suppression of the stress responses to surgery. Disadvantages of using fentanyl as the sole anesthetic include (a) failure to prevent sympathetic nervous system responses to painful surgical stimulation at any dose, especially in patients with good left ventricular function; (b) unpredictable amnestic effects potentially leading to recall; and (c) postoperative depression of ventilation.93–95 Intrathecal fentanyl (maximal benefit achieved with 25 µg) can produce rapid, profound analgesia for early labor with minimal side effects.96

Fentanyl may be administered as a transmucosal preparation (oral transmucosal fentanyl) in a delivery device (several formulations are available, including a lozenge mounted on a handle or a film or rapid-dissolving preparation applied to the buccal mucosa) designed to deliver 5 to 20 µg/kg of fentanyl. The goal is to decrease preoperative anxiety and facilitate the induction of anesthesia, especially in children.97,98 In children 2 to 8 years of age, the preoperative administration of oral transmucosal fentanyl, 15 to 20 µg/kg 45 minutes before the induction of anesthesia, reliably induces preoperative sedation and facilitates induction of inhalation anesthesia.99 These same patients, however, are likely to experience decreases in breathing frequency and arterial oxygenation and an increased incidence of postoperative nausea and vomiting that is not influenced by prophylactic administration of droperidol. In children 6 years of age and younger, the preoperative administration of oral transmucosal fentanyl, 15 µg/kg, is associated with an unacceptably high incidence of preoperative vomiting.100 Conversely, another report did not observe an increased incidence of vomiting or arterial oxygen desaturation after premedication with oral transmucosal fentanyl.101 For treatment of postoperative pain after orthopedic surgery, 1 mg of oral transmucosal fentanyl is equivalent to 5 mg of IV morphine.102 Patients experiencing pain due to cancer may self-administer this opioid to the extent necessary to produce a desirable level of analgesia.
Transdermal fentanyl preparations delivering 75 to 100 µg per hour result in peak plasma fentanyl concentrations in about 18 hours that tend to remain stable during the presence of the patch, followed by a decreasing plasma concentration for several hours after removal of the delivery system, reflecting continued absorption from the cutaneous depot. These transdermal delivery systems were designed to produce stable, long-term fentanyl plasma concentrations in efforts to provide adequate, sustained analgesia for chronic, cancer-related pain. Each transdermal patch contains a depot of fentanyl that provides adequate drug to produce stable plasma fentanyl concentrations for 3 consecutive days. Transdermal fentanyl systems applied before the induction of anesthesia and left in place for 24 hours decrease the amount of parenteral opioid required for postoperative analgesia.103 Acute toxic delirium has been observed in patients with chronic pain due to cancer being treated with transdermal fentanyl for prolonged periods of time.104 It is possible that renal failure and accumulation of norfentanyl contributes to the possible toxic effects of prolonged use of transdermal fentanyl.
In dogs, maximal analgesic, ventilatory, and cardiovascular effects are present when the plasma concentration of fentanyl is approximately 30 ng/mL.105 Thus, the analgesic actions of fentanyl cannot be separated from its effects on ventilation and heart rate. The fact that all receptor-mediated effects are similar at the same plasma concentration of fentanyl suggests saturation of the opioid receptors. Evidence of opioid overdose has been observed when an upper body warming blanket was placed intraoperatively and came into contact with the fentanyl patch and has been linked to increased dermal blood flow and resultant drug uptake caused by the warming.106
Side Effects
The side effects of fentanyl resemble those described for morphine. Persistent or recurrent depression of ventilation due to fentanyl is a potential postoperative problem.107 Secondary peaks in plasma concentrations of fentanyl and morphine have been attributed to sequestration of fentanyl in acidic gastric fluid (ion trapping). Sequestered fentanyl could then be absorbed from the more alkaline small intestine back into the circulation to increase the plasma concentration of opioid and cause depression of ventilation to recur. This, however, may not be the mechanism for the secondary peak of fentanyl, because reabsorbed opioid from the gastrointestinal tract or skeletal muscles, as evoked by movement associated with transfer from the operating room, would be subject to first-pass hepatic metabolism. An alternative explanation for the secondary peak of fentanyl is washout of opioid from the lungs as ventilation to perfusion relationships are reestablished in the postoperative period.
Cardiovascular Effects
Unlike morphine, fentanyl, even in large doses (50 µg/kg IV), does not evoke the release of histamine (see Fig. 7-4).26 As a result, dilatation of venous capacitance vessels leading to hypotension is unlikely. Carotid sinus baroreceptor reflex control of heart rate is markedly depressed by fentanyl, 10 µg/kg IV, administered to neonates (Fig. 7-15).108 Therefore, changes in systemic blood pressure occurring during fentanyl anesthesia have to be carefully considered because cardiac output is principally rate dependent in neonates. Bradycardia is more prominent with fentanyl than morphine and may lead to occasional decreases in blood pressure and cardiac output.

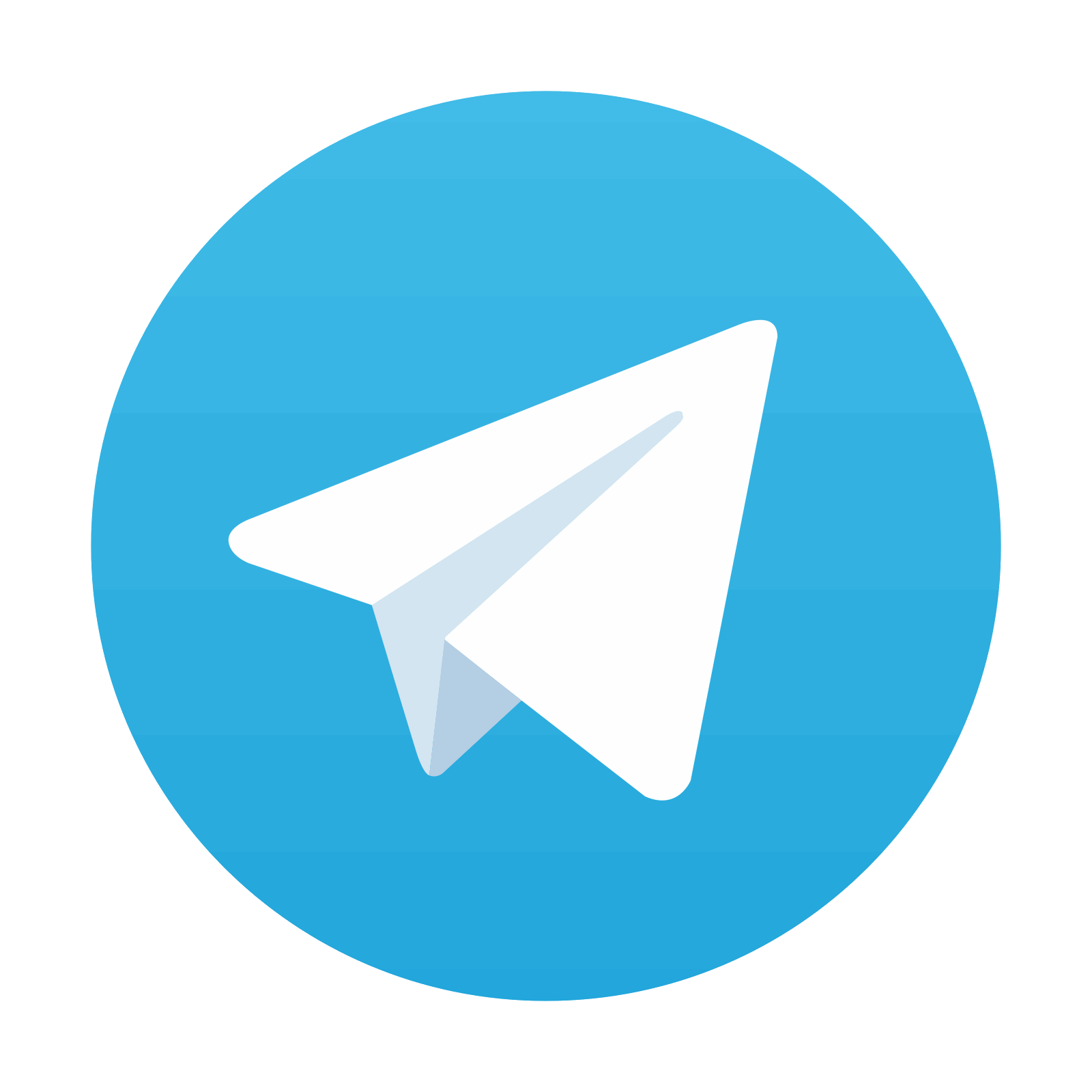
Stay updated, free articles. Join our Telegram channel

Full access? Get Clinical Tree
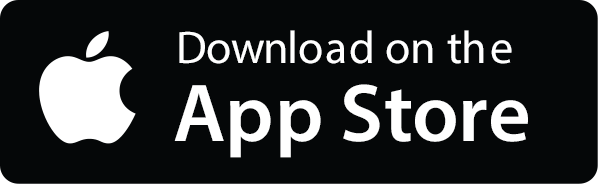
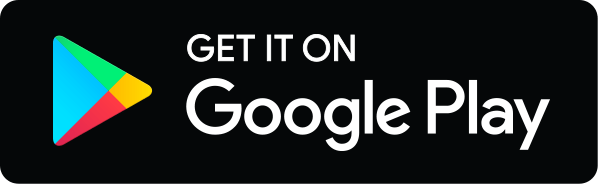