The pituitary gland lies in the sella turcica at the base of the brain and is connected to the hypothalamus by the pituitary stalk. Physiologically, the gland is outside the blood–brain barrier and is divided into the anterior pituitary (adenohypophysis) and posterior pituitary (neurohypophysis). The anterior pituitary synthesizes, stores, and secretes six tropic hormones. ACTH, prolactin, and HGH are polypeptides; thyroid-stimulating hormone (TSH), luteinizing hormone (LH), and follicle-stimulating hormone (FSH) are glycoproteins. The anterior pituitary also secretes β-lipotropin, which contains the amino acid sequences of several endorphins that bind to opioid receptors. The posterior pituitary stores and secretes two hormones—arginine vasopressin (AVP), formerly designated antidiuretic hormone (ADH) and oxytocin. Both are initially synthesized in the hypothalamus and subsequently transported (via axons) to the posterior pituitary (Table 37-2). During the perioperative period, secretion of pituitary hormones increases with activation of the sympathetic nervous system.1

The response to pituitary stalk destruction differs in the anterior and posterior pituitary gland. Stalk destruction causes axonal atrophy and subsequent loss of posterior pituitary function. After stalk destruction, the anterior pituitary can still respond to hypothalamic hormones in the peripheral blood via the inferior hypophyseal artery.
Anterior Pituitary
Anterior pituitary cells have been traditionally classified on the basis of their staining characteristics as agranular chromophobes or granular chromophils. Chromophils are subdivided into acidophils and basophils depending on the staining response to acidic or basic dyes. With more modern techniques, including electron microscopy and immunochemistry, it is possible to identify at least five types of cells, some of which secrete more than one tropic hormone (see Table 37-2).
Growth Hormone (Somatotropin)
Growth hormone (GH) is the most abundant anterior pituitary hormone. GH stimulates growth of all tissues in the body and evokes intense metabolic effects (Fig. 37-1).2 The most striking and specific effect is stimulation of linear bone growth that results from GH action on the epiphyseal cartilage plates of long bones. Excess secretion of GH before epiphyseal closure occurs causes gigantism. When excess GH secretion is excessive after epiphyseal closure and long bones can no longer increase in length but only in thickness, acromegaly results. The metabolic effects of GH include increased rates of protein synthesis (anabolic effect), increased mobilization of free fatty acids (ketogenic effect), antagonism of insulin action (diabetogenic effect) and sodium and water retention. Many of the activities of GH require the generation of a family of peptides known as somatomedins.

Releasing (growth hormone–releasing hormone) and inhibitory (somatostatin) hormones, physiologic events, and medications regulate GH secretion (Table 37-3). For example, perioperative anxiety and stress may evoke the release of GH.1 Plasma concentrations of GH characteristically increase during physiologic sleep. Drugs may influence the secretion of GH, presumably via effects on the hypothalamus. In this regard, large doses of corticosteroids suppress secretion of GH, which may be responsible for the inhibitory effects on growth observed in children receiving high doses of corticosteroids for prolonged periods of time. Conversely, dopaminergic agonists acutely increase the secretion of GH.

Prolactin
Prolactin has little metabolic activity but it prompts the growth and development of the breast in preparation for breast-feeding. Pregnancy stimulates the release of prolactin; dopamine inhibits its release (Table 37-4). Preoperative anxiety also increases plasma concentrations of prolactin.1 Prolactin secretion in response to suckling inhibits ovarian function, explaining the usual lack of ovulation and resulting infertility during breast-feeding.

Gonadotropins
LH and FSH are gonadotropins responsible for pubertal maturation and secretion of steroid sex hormones by the gonads of either sex. These hormones bind to cell membrane receptors in the ovaries or testes to stimulate the synthesis of cAMP.
Adrenocorticotrophic Hormone
ACTH regulates secretions of the adrenal cortex, especially cortisol, and stimulates the formation of cholesterol in the adrenal cortex. Cholesterol is the initial building block for the synthesis of corticosteroids. Secretion of ACTH responds dramatically to stress under the control of corticotropin-releasing hormone from the hypothalamus, as well as a negative feedback mechanism from the circulating plasma concentration of cortisol (Table 37-5).3 Secretory rates of corticotropin-releasing hormone and ACTH are high in the morning and low in the evening. This diurnal variation results in high plasma cortisol concentrations in the morning (~20 µg/dL) and low levels (~5 µg/dL) around midnight. For this reason, plasma concentrations of cortisol are interpreted in terms of the time of day of the measurement. Surgical incision, reversal of anesthesia, and postoperative pain stimulate ACTH release.1,4,5

In the absence of ACTH, the adrenal cortex undergoes atrophy, but the zona glomerulosa, which secretes aldosterone, is least affected. Indeed, hypophysectomy has minimal effects on electrolyte balance because of the continued release of aldosterone from the adrenal cortex. Pigmentary changes that may accompany certain endocrine diseases most likely reflect changes in plasma concentrations of ACTH, revealing the melanocyte-stimulating effects of this hormone. For example, pallor is a hallmark of hypopituitarism. Conversely, hyperpigmentation in patients with adrenal insufficiency from primary adrenal gland disease reflects high concentrations of ACTH circulating in plasma as the anterior pituitary attempts to stimulate corticosteroid secretion.
Chronic administration of corticosteroids suppresses corticotropin-releasing hormone and leads to atrophy the hypothalamic-pituitary axis. Several months may be required for recovery of this axis after removal of the suppressive influence. In such patients, stressful events during the perioperative period might evoke life-threatening hypotension. For this reason, it is a common practice to administer supplemental exogenous corticosteroids (based on the magnitude of stress) to patients considered at risk for suppression of the hypothalamic-pituitary axis. There is little evidence, however, that supplemental corticosteroids in excess of normal daily physiologic secretion are necessary or beneficial intra- or postoperatively.6 See Chapter 40 for dosing of corticosteroids during the perioperative period.
Thyroid-Stimulating Hormone
TSH accelerates all the steps in the formation of thyroid hormones, including initial uptake of iodide into the thyroid gland. TSH causes proteolysis of thyroglobulin in the follicles of thyroid cells to release thyroid hormones into the circulation. Secretion of TSH from the anterior pituitary is under the control of thyrotropin-releasing hormone from the hypothalamus as well as a negative feedback mechanism, depending on the concentrations of thyroid hormones circulating in plasma. Sympathetic nervous system stimulation and corticosteroids also suppress the secretion of TSH and thus diminish activity of the thyroid gland. Thyrotropin-releasing hormone is widely distributed in the central nervous system and is a potent analeptic. TRH stimulates respiratory rate, induces tremor, and reduces sleep time.
A long-acting thyroid stimulator is an immunoglobulin A antibody that binds to receptor sites on thyroid cells. Binding can mimic the effects of TSH and account for hyperthyroidism, and patients with hyperthyroidism often have detectable circulating concentrations of these proteins. Hypothyroidism with increased plasma concentrations of TSH indicates a primary defect at the thyroid gland (primary hypothyroidism) and an attempt by the anterior pituitary to stimulate hormonal output by releasing TSH. A defect at the hypothalamus or anterior pituitary is indicated by low concentrations of both TSH and thyroid hormones circulating in plasma (secondary hypothyroidism).
Posterior Pituitary
The cells in the posterior pituitary act as supports for the terminal nerve endings of fibers from the supraoptic and paraventricular nuclei of the hypothalamus. AVP is synthesized in the supraoptic nuclei and oxytocin in the paraventricular nuclei. These hormones are transported in secretory granules along axons from corresponding nuclei in the hypothalamus to the posterior pituitary for subsequent release in response to appropriate stimuli.
Arginine Vasopressin
The physiologic functions of AVP include vasoconstriction, water retention, and corticotropin secretion. Decreases in blood volume, increased plasma osmolality, and decreased arterial pressure stimulate AVP release7 (Table 37-6). With hydration and establishment of an adequate blood volume before induction of anesthesia, urine output is maintained by blunting the release of AVP associated with painful stimulation or fluid deprivation before surgery. Concentrations of AVP in the plasma in response to acute decreases in the volume of extracellular fluid may exert direct vasopressor effects on arterioles and thus contribute to maintenance of systemic blood pressure. Administration of morphine, or other opioids, in the absence of painful stimulation does not evoke the release of AVP. Ethanol inhibits the secretion of AVP. Decreases in urine output and fluid retention previously attributed to release of AVP during positive pressure ventilation of the lungs are more likely the result of changes in cardiac filling pressures that impair the release of atrial natriuretic hormone.

There are three subtypes of AVP receptors, V1, V2, and V3. Stimulation of V1 receptors (found on vascular smooth muscle) causes vasoconstriction. AVP is used as a vasopressor during intraoperative hypotension, sepsis, and cardiopulmonary resuscitation. Activation of the V2 receptors, which are located on collecting duct cells in the kidney, increases reabsorption of water. AVP is transported in the blood to the kidneys, where it attaches to receptors on the capillary side of epithelial cells lining the distal convoluted renal tubules and collecting ducts of the renal medulla. The receptor-hormone interaction results in the formation of large amounts of cAMP, which causes insertion of aquaporin-2 into the collecting duct walls for exit of water to minimize osmolality. Hypokalemia, hypercalcemia, cortisol, and lithium also interfere with renal responsiveness to AVP. AVP binds to V3 receptors in the adenohypophysis to release corticotropin, which suggests that this hormone affects the stress response.7
Destruction of neurons in or near the supraoptic and paraventricular nuclei of the hypothalamus from pituitary surgery, trauma, cerebral ischemia, or malignancy may decrease vasopressin release to cause central diabetes insipidus.7 If the posterior pituitary alone is damaged, however, the transected fibers of the pituitary stalk can still continue to secrete AVP. Diabetes insipidus from lack of vasopressin release during pituitary surgery is usually transient. (See Chapter 40 for hormonal treatment of central diabetes insipidus.)
Unnecessary or excessive secretion of AVP with subsequent retention of water and dilutional hyponatremia may result from head injuries, intracranial tumors, meningitis, or pulmonary infections. Aberrant production of AVP is observed most commonly in patients with cancer, especially oat cell carcinoma, in which the tumor itself produces AVP. In cancer patients, the antibiotic demeclocycline promotes diuresis by antagonizing the effects of AVP on renal tubules.
Oxytocin
Breast suckling and cervical and vaginal dilation stimulate oxytocin secretion. Oxytocin ejects milk from the lactating mammary gland via contraction of the myoepithelial cells that surround the alveoli of the mammary glands. Oxytocin binds to G proteins on the surface of uterine myocytes to trigger the release of calcium from the sarcoplasmic reticulum, exerting a contracting effect on the pregnant uterus.8 Oxytocin also augments the action potential of the uterine smooth muscle.9 Large amounts of oxytocin cause sustained uterine contraction as necessary for postpartum hemostasis. Oxytocin has only 0.5% to 1.0% the antidiuretic activity of AVP and can be released abruptly and independently of AVP.
Thyroid Gland
The thyroid gland maintains optimal metabolism for normal tissue function.10 The principal hormonal secretions of the thyroid gland are thyroxine (T4) and triiodothyronine (T3) (Fig. 37-2). T4, a prohormone synthesized from tyrosine, represents 80% of the body’s thyroid hormone production. T3, five times more active than T4, is produced directly from tyrosine metabolism or from conversion of T4 in peripheral tissues. Two distinct deiodases (located in the liver, kidneys, and central nervous system) metabolize T4 and T3 to inactive compounds. The half-lives of endogenously or exogenously administered T3 and T4 are 1.5 and 7 days, respectively. T3 and T4 are both highly protein bound to albumin, thyroid-binding prealbumin, and thyroid-binding globulin with only 0.2% of T3 and 0.3% of T4 freely circulating unbound and pharmacologically active.10 It is of interest that iodine present in thyroid hormones is not necessary for biologic activity (see Fig. 37-2). In addition to thyroid hormones, the thyroid gland secretes calcitonin, which is important for calcium ion use.

The thyroid hormones increase oxygen consumption in nearly all tissues, except for the brain. Failure of thyroid hormones to greatly alter the oxygen consumption of the brain is consistent with the minimal changes in anesthetic requirements (MAC) that accompany hyperthyroidism or hypothyroidism.11 Cardiovascular changes are often the earliest clinical manifestations of abnormal thyroid hormone levels. Absence of thyroid gland hormones decreases minute oxygen consumption to approximately 40% less than normal; excesses of thyroid hormones can expand oxygen consumption as much as 100% more than normal. Thyroid hormones stimulate carbohydrate metabolism and facilitate the mobilization of free fatty acids. Despite the latter effect, plasma concentrations of cholesterol usually decrease, reflecting stimulation of low-density lipoprotein receptor synthesis by thyroid hormones.
Anatomically, the thyroid gland consists of two lobes connected by a bridge of tissues known as the thyroid isthmus (Fig. 37-3). The gland is highly vascularized and receives innervation from the autonomic nervous system. Structurally, the gland consists of multiple follicles (acini) that are filled with colloid, which consists principally of thyroglobulin. Thyroid hormones are stored in combination with thyroglobulin. Stimulation of proteases by TSH results in cleavage of hormones from thyroglobulin and their release into the systemic circulation.

Mechanism of Action
When thyroid hormones enter cells, T3 binds to nuclear receptors. T4 also binds to these receptors but not as avidly. Indeed, T4 serves principally as a prohormone for T3, so that the biologic effects of T4 are largely a result of its intracellular conversion to T3.
Thyroid hormones exert most, if not all, of their effects through control of protein synthesis. Thyroid hormones activate the DNA transcription process in the cell nucleus to form new cell proteins and enzymes. Sympathomimetic effects that accompany thyroid hormone stimulation most likely reflect a greater number and sensitivity of β-adrenergic receptors to release of T4 and T3. It has been proposed that thyroid hormones modulate conversion of α-adrenergic to β-adrenergic receptors. Cardiac cholinergic receptor numbers are decreased by thyroid hormones, which is consistent with an increase in heart rate that is out of proportion to the increase in cardiac output.
When thyroid hormones accelerate metabolism, tissues vasodilate and blood flow delivers necessary oxygen and carries away metabolites and heat. As a result, cardiac output often increases but systemic blood pressure is unchanged because peripheral vasodilation offsets the impact of more blood flow. Excess protein catabolism associated with greater secretion of thyroid hormones is the mechanism behind skeletal muscle weakness characteristic of hyperthyroidism. The fine muscle tremor that accompanies hyperthyroidism stems from the sensitivity of neuronal synapses in the area of the spinal cord that controls skeletal muscle tone. Diarrhea reflects additional motility in the gastrointestinal tract with excessive activity of the thyroid gland.
Calcitonin
Calcitonin, a polypeptide hormone secreted by the thyroid gland, decreases the concentration of calcium ions in plasma by weakening the activity of osteoclasts and strengthening the activity of osteoblasts. Calcitonin works in the early moments after ingestion of high-calcium meals. A total thyroidectomy and subsequent absence of calcitonin, however, does not measurably influence the plasma concentration of calcium, because of the predominance of parathyroid hormone.
Parathyroid Glands
The four parathyroid glands secrete parathyroid hormone (PTH), an amino and acid polypeptide that regulates plasma concentration of calcium ions. Secretion of PTH is inversely related to plasma ionized calcium concentration. Small declines in the plasma concentration of calcium ions stimulate the release of PTH. PTH promotes mobilization of bone calcium (osteoclastic activity); enhances conversion of vitamin D to its active form, 1,25-dihydroxycholecalciferol, to increase gastrointestinal absorption of calcium; increases renal tubular absorption of calcium; and inhibits renal reabsorption of phosphate to increase calcium and to decrease phosphate concentrations in plasma.
Parathyroid hormone exerts its effect on target cells in bones, renal tubules, and the gastrointestinal tract by stimulating the formation of cAMP. Because a portion of cAMP synthesized in the kidneys escapes into the urine, its assay serves as a measure of parathyroid gland activity.
Adrenal Cortex
The adrenal cortex secretes three major classes of corticosteroids: mineralocorticoids, glucocorticoids, and androgens. The precursor of all corticosteroids is cholesterol. More than 30 different corticosteroids have been isolated from the adrenal cortex, but only two are important: aldosterone, a mineralocorticoid, and cortisol, the principal glucocorticoid (Table 37-7). The corticosteroids are not stored in the adrenal cortex; the rate of synthesis determines the subsequent plasma concentration. Anatomically, the adrenal cortex is divided into three zones designated the (a) zona glomerulosa that secretes mineralocorticoids, (b) zona fasciculata that secretes glucocorticoids, and (c) zona reticularis that secretes androgens and estrogens.

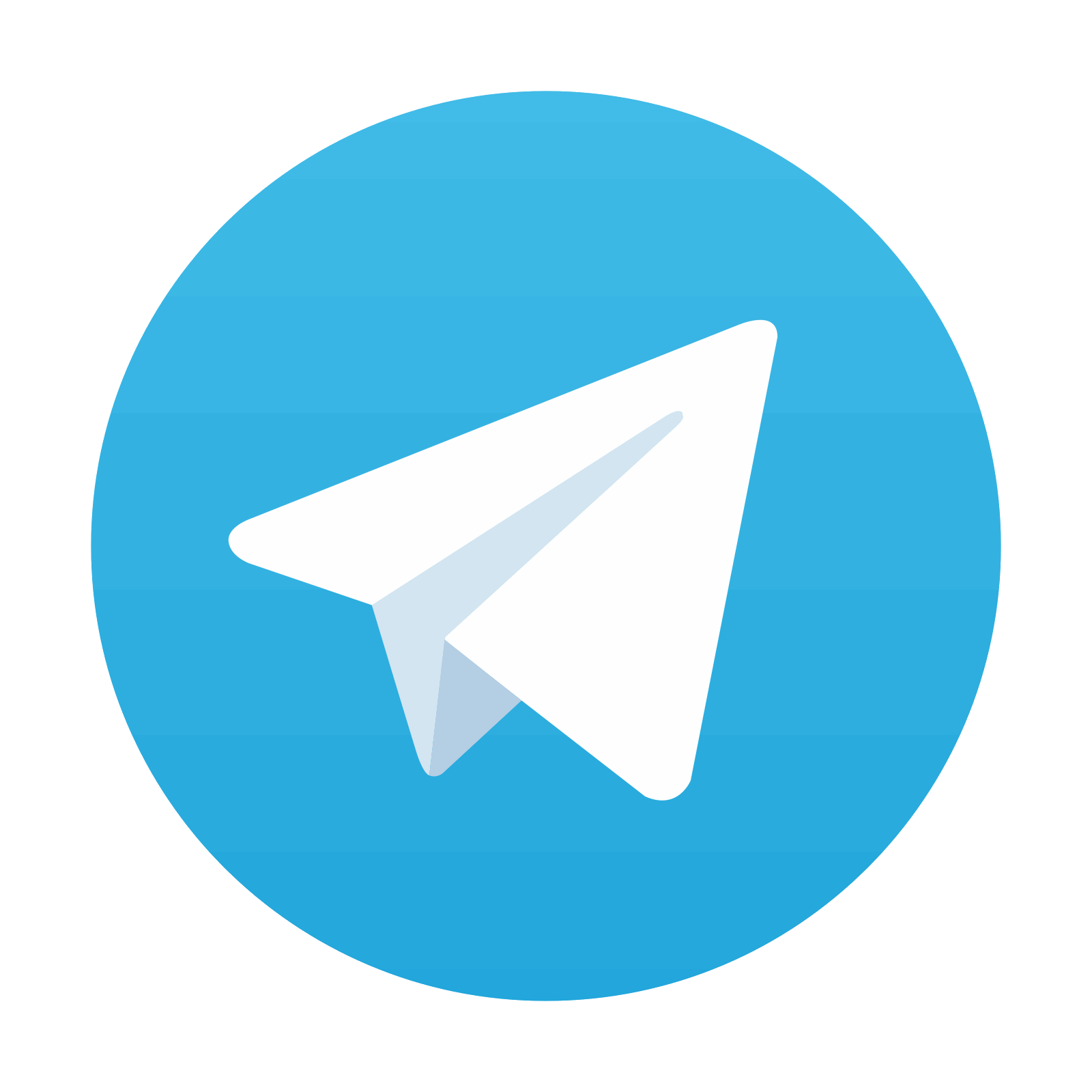
Stay updated, free articles. Join our Telegram channel

Full access? Get Clinical Tree
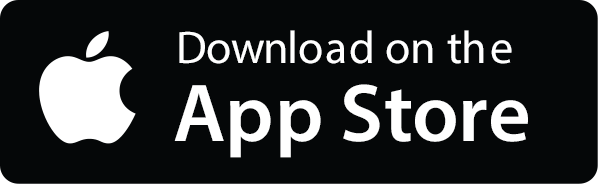
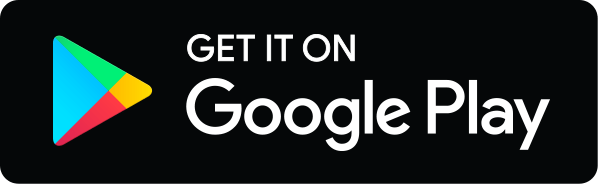