Operational Medicine Environmental Considerations
Bret T. Ackerman
Ian S. Wedmore
OBJECTIVES
After reading this section, the reader will be able to:
1. Describe the operational medical impactrelated to altitude effects and illness.
2. List presenting signs and symptoms and describe the initial treatment for cold environment injuries resulting from frostbite, trench foot, and hypothermia.
3. Discuss the pathophysiology, presentation and treatment of heat related illnesses from mild heat related illness to the life-threatening emergency of heat stroke.
HISTORICAL PERSPECTIVE
The environment and its effects on the body during tactical operations has always been a medical concern. Frostbite, for example, has accounted for over 1 million American casualties in World War I, World War II, and the Korean Conflict. The German army on the Eastern front performed over 15,000 amputations in 1942 solely for frostbite (1). The global war against terrorism has increased the requirement for providers to be knowledgeable in this area. Operations in Afghanistan occur at elevations well over 12,000 feet and potentially higher. The battle at Roberts Ridge occurred at 10,240 feet and most of operation Anaconda took place at elevations above 11,000 feet. Bagram Airfield itself is at a 5,000-foot elevation. This location provides challenges in altitude as well as cold. Operations in Iraq result in heat stress for many months of the year, as do operations for much of the year in Afghanistan, the Philippines, and Africa. Tactical operations in the continental United States (CONUS) can result in extremes of heat, cold, and altitude that can place the tactical provider at risk for an environmental injury or illness.
Altitude Effects and Illness
As altitude increases, barometric pressure and temperatures decrease. The decrease in barometric pressure results in a drop in the partial pressure of oxygen, and, therefore, hypoxia occurs in persons breathing ambient air at altitude. The relative concentration of oxygen remains the same 21% as that at sea level, but the relative drop in overall pressure of the ambient environment results in a decrease in partial pressure of oxygen at the alveolar level that results in a decrease in diffusion of oxygen across the alveolar arterial interface, and hypoxemia can ensue from this drop in pressure to cause oxygen diffusion. For example, at sea level barometric pressure is 760mm Hg, and oxygen is 21% of the composition of air. At 12,000 feet (3,658 meters), the barometric pressure is only 483mm Hg, resulting in a 40% drop in available oxygen per breath. Complicating the decrease in oxygen pressure to diffuse oxygen into the blood is an increased propensity to develop pulmonary edema secondary to a variety of factors that are not completely understood but are in part due to the relative drop in ambient pressure and changes in vascular tone in response to hypoxia and altitude changes. Similar changes in the brain can result in cerebral edema. Operators exposed to the low pressures and hypoxia of altitude will certainly suffer from a decrement in physical ability as well as the possibility of developing mild to severely debilitating or life-threatening illness.
Incidence of Altitude illness
The effects of altitude may be noticed at elevations as low as 1,500 meters (5,000 feet); the level at which the body begins to manifest a physiologic response to altitude. Symptoms of illness related to altitude, however, are rarely seen
below 2,000 meters (7,000 feet) (2). The incidence of altitude illness increases with higher elevations but is also dependent on the rate of ascent. Experience provides a guide as to what percentage of personnel can be expected to be affected by altitude illness and the reduction it will have on personnel efficacy.
below 2,000 meters (7,000 feet) (2). The incidence of altitude illness increases with higher elevations but is also dependent on the rate of ascent. Experience provides a guide as to what percentage of personnel can be expected to be affected by altitude illness and the reduction it will have on personnel efficacy.
▪ 20% to 25% of visitors to between 7,000 to 9,000 feet developed altitude illnesses (3).
▪ 67% of climbers of Mt. Rainier who ascend to 14,400 feet in 36 hours develop altitude illness (4).
▪ Indian soldiers flown rapidly to over 10,000 feet had a high incidence of acute mountain sickness (AMS) and a 2% to 15% incidence of high altitude pulmonary edema (HAPE) with an associated high mortality (5).
The incidence of altitude illness is also affected by individual susceptibility, level of exertion, and age. The only good predictor of the likelihood of developing altitude illness is a prior history of altitude exposure and previous altitude illness. If a person had a previous incidence of altitude related illness and the altitude and ascent rates do not change, then one is likely to become just as ill or do just as well as previously.
Pathophysiology of Altitude Adaptation
Pulmonary Response
The initial response of the body to exposure to altitude is to increase the respiratory rate in an attempt to normalize PaO2, resulting in a reduction of CO2. This is first noted at approximately 1,500 meters of elevation (6). This rapidly results in a respiratory alkalosis that is compensated by an excretion of bicarbonate through the kidneys to attempt normalization of the pH. At the summit of Mt. Everest (29,000 feet), resting respiratory rates of 50 to 60 have been recorded.
Cardiovascular Response
The resting heart rate increases with altitude until the body is acclimatized, at which point it returns to normal. The maximum heart rate decreases with altitude. At very extreme altitudes, the two approach each other impacting the maximum work effort of the individual. Changes that occur in the vascular are poorly understood. The most important changes that occur are that of the pulmonary vasculature and cerebral blood flow. The hypoxia that results from the drop in ambient pressure results in pulmonary vasoconstriction, and an idiopathic inflammatory response in the pulmonary capillary bed. This inflammatory response results in leaky capillaries and resulting pulmonary edema. A similar inflammatory response may occur in the cerebral vasculature resulting in cerebral edema.
Hematopoietic Response
There is an increase in relative concentration of red blood cells (RBC) at altitude, initially due to hemoconcentration from the bicarbonate induced diuresis from the kidneys. When a person is exposed to high altitudes, there is also an increased production of erythropoietin, which leads to a true increase in RBC mass about 5 to 7 days after altitude exposure.
Immunologic Response
Cellular immunity is compromised at altitude and all individuals are to some extent immunocompromised because of this. Any pre-existing infection will tend to be somewhat refractory to treatment while at altitude.
Renal Response
Due to the respiratory alkalosis created from hyperventilation, a diuresis is normal on arrival at altitude as the kidneys excrete bicarbonate. Most people will exhibit polyuria at altitude, which is an indication of proper adaptation.
Ocular Effects
Altitude exposure negatively effects color vision, most predominantly in the blue-yellow range (7). Individuals who have undergone radial keratotomy (RK) should not go to elevation as the ocular hypoxia causes a distortion the RK scar. PRK does not appear to have this same issue (8).
At extreme elevations over 17,000 feet high, altitude retinopathy is common. On funduscopic exam, numerous ruptured blood vessels can be seen. Fortunately, there is typically no effect on the vision and the retinopathy usually resolves after return to sea level.
Prevention of Altitude Illness
Prevention of altitude illness is preferred to treatment. Those with altitude illness will be impaired to carry out operational missions. Staged acclimatization is the most effective technique of preventing altitude illness. When an altitude of 2,500 meters is reached, the altitude where an individual sleeps should be increased no more than 600 meters a night. Intermittent daytime altitude exposure is also effective in acclimatizing personnel to altitude.
Pharmacologic Prophylaxis
Acetazolamide
Acetazolamide is the most extensively studied of pharmacologic prophylactic agents for prevention of altitude illness. It inhibits carbonic anhydrase, which results in bicarbonate excretion by the kidneys and decreased production
of cerebrospinal fluid. It is 75% effective in preventing altitude illness in individuals who rapidly ascend between 4,000 and 4,500 meters (9,10). Acetazolamide’s method of action enhances the bodies’ acclimatization to altitude by assisting in the excretion of bicarbonate.
of cerebrospinal fluid. It is 75% effective in preventing altitude illness in individuals who rapidly ascend between 4,000 and 4,500 meters (9,10). Acetazolamide’s method of action enhances the bodies’ acclimatization to altitude by assisting in the excretion of bicarbonate.
The prophylactic dose is 125 to 250 mg PO twice a day starting 24 hours prior to ascent and continuing for 48 hours after altitude arrival. This is a lower dose than previously mentioned in the literature, but it is effective. The lower dose is designed to reduce the side effects while maintaining effective prophylaxis. The side effects of acetazolamide include polyuria, peripheral paraesthesias, nausea, and myopia (due to its effect of reducing intraocular pressure). It is also a sulfa-based drug and, as such, should not be utilized by those with a sulfa allergy. Reactions to acetazolamide have been reported in persons with no prior allergy and who are not allergic to sulfa drugs and, therefore, a test dose should be considered before travel (11).
Dexamethasone
Dexamethasone is also useful for the prevention of altitude illness and is as effective initially as acetazolamide (12,13). It does not facilitate acclimatization as acetazolamide does and has the significant side effects common to all steroids such as withdrawal rebound, emotional liability, immune suppression and others. It may be used by individuals with sulfa allergies. Discontinuation of dexamethasone while at altitude often results in the rapid return of altitude illness symptoms. There are anecdotal reports of death due to sudden discontinuation (14).
The combination of dexamethasone and acetazolamide appears to be the best prophylaxis for unacclimatized individuals who must ascend quickly to high altitudes and function well on arrival for a short duration mission, such as a rescue or raid (15). This combination has been shown to be effective in reducing illness in individuals going from 12,000 to 17,000 feet in 2 hours, a movement profile that would likely mimic military or tactical operations. In this operation, acetazolamide 500 mg sustained release every day as well as 4 mg Dexamethasone every 12 hours was utilized and no subject on the combination reached symptom threshold for AMS (16). The standard dexamethasone dose for prophylaxis is 4 mg PO every 6 hours, although 4 mg PO twice a day may be effective (17).
Gingko Biloba
The literature on gingko biloba is mixed in its efficacy in preventing AMS with results ranging from 100% effective to no better than placebo (18,19). Because standardization of gingko extracts in nutraceuticals is nonexistent, it makes interpretation of these studies difficult. Expert opinion suggests that if the gingko formulation is good then prophylaxis may be attained. The present recommendation for dosing is 120 mg PO twice a day. The side effect profile of gingko is minimal, leading to its recommended use. There are case reports of gingko effecting platelet function and potentially causing bleeding, but this increased bleeding risk has not been substantiated in actual bleeding studies in patients on gingko.
Medications that have been shown to be ineffective:
▪ Magnesium
▪ Naproxen
▪ Phenytoin
▪ Nifedipine (for AMS) (20)
▪ Antacids
▪ Montelukast
Acute Mountain Sickness
AMS the most common form of altitude illness. The symptoms begin shortly after arrival at high altitude, typically 3 to 24 hours after ascent. Symptoms reach peak severity in 24 to 72 hours and usually subside over the course of 3 to 7 days, but the duration is variable. Up to 40% of people who develop AMS will have symptoms at 7 days and up to 13% after 1 month.
AMS is the initial part of the spectrum of altitude illness and they are both likely extensions of the same underlying pathophysiology. After developing AMS, further ascent without an acclimation period usually exacerbates symptoms and can result in HAPE and high altitude cerebral edema (HACE).
Symptoms:
▪ Headache
▪ Dizziness
▪ Dry cough
▪ Nausea
▪ Emesis
▪ Malaise
▪ Anorexia
The differential diagnosis includes: flu, dehydration, exhaustion, hangover, viral syndrome, arterial gas embolism, and carbon monoxide poisoning.
Those with AMS should not advance their sleeping elevation nor ascend higher until the symptoms abate (typically 24 to 48 hours); however, it is not necessary to descend if symptoms are not severe.
Treatment medications include all those previously described for prophylaxis, but at potentially higher doses.
▪ Acetazolamide 125 to 500 mg PO BID
▪ Dexamethasone 4 to 8 mg followed by 4 mg q6h
▪ Low-flow oxygen
▪ Symptomatic treatment with prochlorperazine (also increase HVR)
▪ APAP or nonsteroidal anti-inflammatory drugs for symptomatic treatment of the headache
▪ Hyperbaric therapy: compression in a portable fabric hyperbaric chamber (such as a Gamow bag) can be effective in rapidly improving AMS symptoms.
HACE
HACE is brain edema caused by altitude exposure due to vasogenic changes in response to low barometric pressure and hypoxia. This vasogenic edema affects mostly the white matter of the brain. Although most individuals who ascend to high altitudes are likely to develop some minimal degree of cerebral edema, most of it is subclinical and with mild or nonexistent symptoms. As the edema increases, it becomes more symptomatic and potentially fatal. HACE can occur as low as 2,430 meters (8,000 feet), but the vast majority of cases occur above 3,600 meters (12,000 feet). Untreated HACE can worsen over 1 to 3 days or may become fulminate and result in death in <12 hours.
Fatal HACE is seen most often in people who have severe AMS symptoms and continue to ascend.
HACE represents the end stage of cerebral AMS. Symptoms include:
▪ Severe headache
▪ Emesis
▪ Altered mental status
▪ Ataxia: this is the pathognomonic sign of HACE; anyone with truncal ataxia at high altitudes has HACE until proven otherwise.
HACE treatment:
▪ Anyone suspected of or suffering from HACE must descend or be evacuated immediately.
▪ Mortality without descent is significant.
▪ Dexamethasone 8mg followed by 4mg q6h by any route available: IV, IM, PO
▪ Oxygen 2 to 4 L/min
▪ Hyperbaric therapy (Gamow bag or equivalent), if descent is not possible.
▪ If reascent must occur, wait at least 72 hours after symptom resolution at a lower elevation.
HAPE
HAPE, untreated, has an extremely high mortality. The pathophysiology is due to a breakdown in the vascular alveolar barrier and “leaky lungs,” markedly elevated pulmonary artery pressures, and regional over-perfusion of the lungs. It is a noncardiogenic pulmonary edema; therefore, vascular fluid overload is not the issue and treatments typically utilized for cardiogenic pulmonary edema (such as nitrates and loop diuretics) will not be efficacious.
HAPE occurs in more than 10% of the people ascending above 3,700 meters; the onset is typically 2 to 4 days after rapid ascent to altitudes >2,438 meters (11,500 feet). Because it is normal for one to desaturate somewhat with sleeping, the onset or sudden worsening of HAPE at night is common.
HAPE Early Symptoms:
▪ Nonproductive cough
▪ Increasing nocturnal cough
▪ Rales can occasionally be noted on exam, but this is NOT pathoneumonic for HAPE
▪ Dyspnea on exertion greater than others at that altitude
▪ Fatigue, often manifested by falling behind others in activities
▪ Weakness with decreased tolerance for physical activity and increased time for recovery after physical exertion
▪ A fit individual who is suddenly always falling behind at altitude should be suspected of developing HAPE.
▪ Resting tachycardia and tachypnea greater than induced by altitude alone
▪ Dyspnea at rest: this symptom indicates HAPE is imminen.
▪ Once symptoms appear, HAPE can progress very rapidly (<12 hours) to coma and death.
▪ Nail beds and lips may be more cyanotic than other climbing members.
HAPE progressive symptoms:
▪ The cough produces frothy and sometimes pink or bloodstained sputum.
▪ Rales become more numerous and widespread.
▪ Wheezing may develop.
▪ Lung sounds progress to being audible (without stethoscope), especially when the individual is supine.
▪ Orthopnea may occur (<20%).
▪ Progressive hypoxemia causes dyspnea and cyanosis.
▪ Marked hypoxia by oximetry
▪ Mental status deteriorates with progressive confusion and sometimes vivid hallucinations (due to hypoxemia).
▪ Obtundation, coma, and death occur without treatment.
▪ Subfebrile/low-grade febrile temperature <38°C (100.5°F)
Dyspnea at rest and cough should be considered to be the onset of HAPE.
DELAY IN TREATMENT OF PROGRESSIVE HAPE USUALLY RESULTS IN DEATH!
HAPE Treatment:
▪ Depends on severity.
▪ Descent is mandatory; descent of even a few hundred meters (300 to 1,000 meters) is helpful and even curative in mild cases and lifesaving in severe cases.
▪ Mortality in severe cases can approach 50% if descent can not be accomplished rapidly.
▪ O2 by mask or cannula.2 to 6 L/min (mild) or 4 to 6 L/min by mask (moderate and severe). DO NOT DELAY DESCENT.
▪ Hyperbaric therapy in a portable fabric hyperbaric chamber may be lifesaving: this should be utilized if descent cannot occur.
▪ Nifedipine 10 mg TID sublingually or 20 mg PO. A second 10 mg sublingual dose can be administered in 15 to 20 minutes, if no improvement in symptoms is apparent (followed by 30 mg TID).
▪ Nifedipine should not be used in lieu of descent; supplemental oxygen or treatment in a hyperbaric bag is appropriately used in conjunction with each of the other therapies.
▪ Dexamethasone my improve HAPE by stabilizing or decreasing the vascular endothelium leakage and as such can be utilized as well. Use HACE treatment doses.
▪ In all cases, move to lower elevation; if resolved, wait at least 72 hours before attempted return to previous elevation.
▪ Salmeterol has been shown to be effective for HAPE treatment; albuterol should also be effective due to the same mechanism (21).
▪ Other drugs that reduce pulmonary artery pressure, such as sildenafil, phentolamine, and hydralazine, have also been shown to be effective in reducing HAPE, although they have not been as well studied as nifedipine.
Prophylaxis
In individuals known to be very susceptible to HAPE who must ascend to an elevation where they have developed HAPE in the past, prophylaxis can be utilized.
Nifedipine has been shown to be effective prophylaxis of HAPE in susceptible individuals (22). Salmeterol or albuterol may also have some prophylactic benefit.
High Altitude Pharyngitis and Bronchitis
This is a common condition occurring typically after 2 to 3 weeks at altitude; it is not a true illness, but rather an inflammatory response to the environment from breathing cold dry air. It may represent the most common altituderelated condition seen at altitude (23). It is not life threatening and requires only symptomatic treatment. Its greatest significance is in being able to differentiate it from the onset of HAPE. Some key discriminators are the timeframe: this develops after a longer time at altitude; HAPE develops 2 to 4 days after the arrival at a new altitude. Most important, the patient will not have dyspnea at rest. If there remains any doubt, treat as if HAPE is the diagnosis. Symptomatic treatment includes lozenges and the wearing of a scarf over the mouth.
Tactical Considerations
Proper acclimatization with graded ascents of intermittent altitude stays prior to combat operations is the ideal method of altitude acclimatization. Three weeks of intermittent altitude exposure (4 hours a day for 5 days a week) can reduce the incidence of altitude illness. This approach can be utilized by having personnel do training missions at altitude prior to an actual prolonged combat mission at altitude. For example, in Afghanistan, newly arrived unacclimatized troops would benefit from a few weeks of air assault training onto the surrounding high peaks, returning to Bagram in between. Acclimatization gained by time spent at a high altitude will last at least 8 days after the return to sea level.
Acetazolamide is effective in reducing AMS symptoms, but it also reduces exercise/work ability (24). This effect has been well shown when acetazolamide is taken at low elevations. It is unclear if this reduction in work ability is overcome by the decrease in AMS and increased acclimatization. Although, in at least one study, the workload was not reduced when acetazolamide was utilized at altitude.
For short duration missions to high elevations with unacclimatized troops, the best pharmacologic prophylaxis is dexamethasone and acetazolamide in combination. If troops are to utilize dexamethasone, a few trial doses at low elevation are warranted to determine those who develop significant psychological side effects from its use.
Cold Environment Injuries
Cold environments have inflicted its own unique constellation of injuries on participants in tactical operations both directly and in combination with separate medical illness or traumatic injuries. Complications of cold environments have had marked impacts on the military expeditions, notably Hannibal during his crossing of the Alps in 218 B.C. the American army garrisoned at Valley Forge in the winter of 1777 to 1778, World Wars I and II, Korea, and the Falkland War (25). One of the more recent examples are the deaths of several army ranger students who died of complications of accidental hypothermia in 1995 due to immersion in water during cold temperatures (26).
Frostbite and Trench Foot
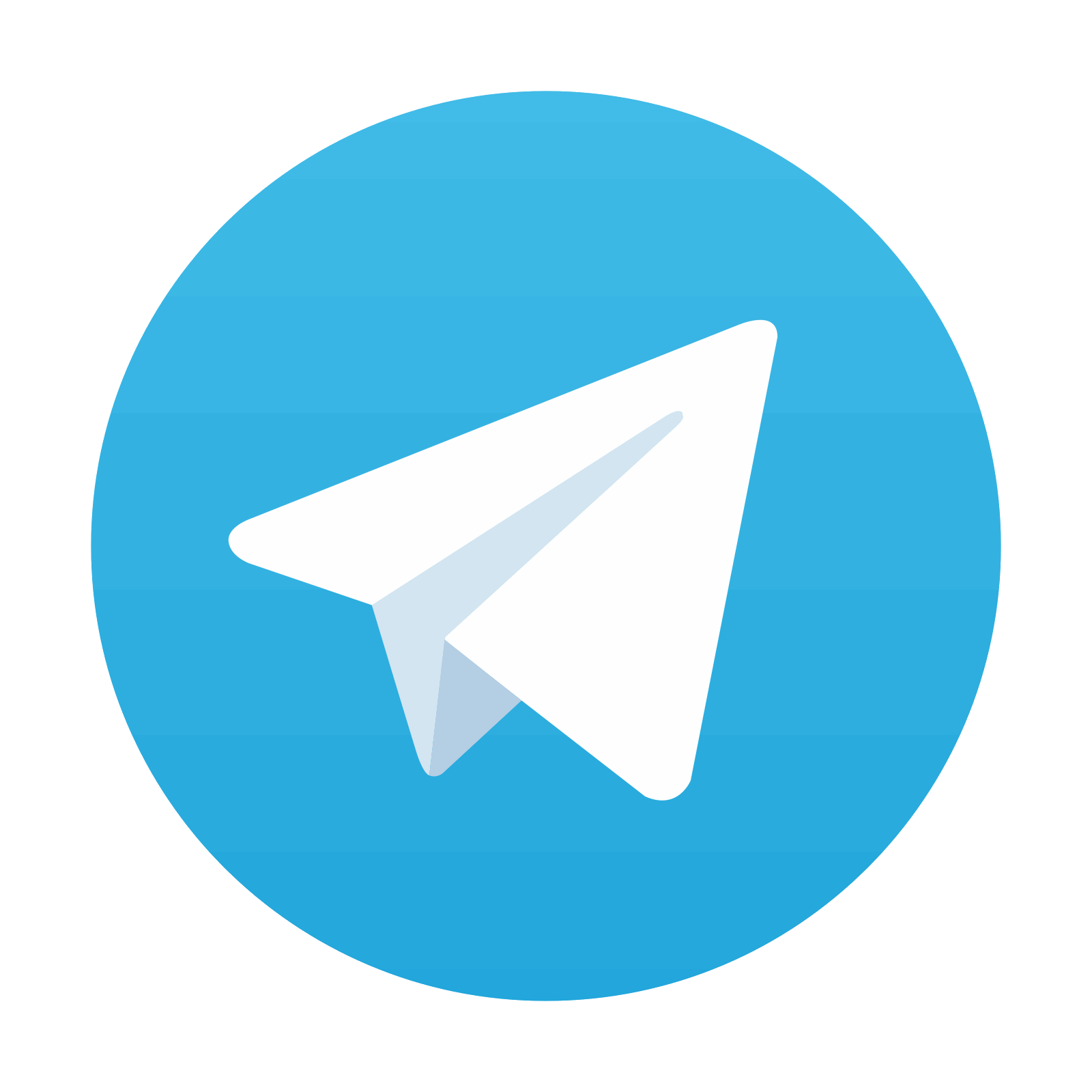
Stay updated, free articles. Join our Telegram channel

Full access? Get Clinical Tree
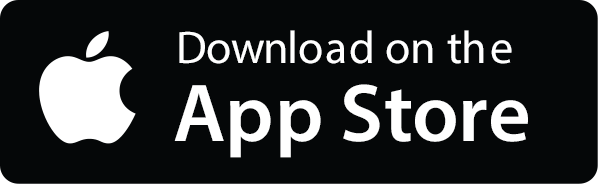
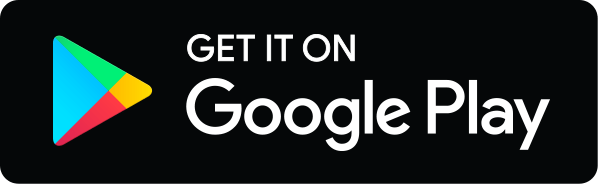