div class=”ChapterContextInformation”>
4. Prediction of Life-Threatening Hemorrhage
Keywords
TraumaTransfusionResuscitationDamage controlProtocolHemorrhageWhole bloodPlasmaRBCsPlateletsScoringPredictionIntroduction
Trauma-related mortality is one of the top causes of overall mortality in a number of age groups including both the young and the elderly in industrialized countries [1, 2]. This burden carries not only a toll on society but also a financial toll on the healthcare system as a whole. Of these trauma-related deaths, up to 50% can be attributed to uncontrolled hemorrhage [3]. Massive uncontrolled hemorrhage has been shown to lead to a lethal triad of coagulopathy, hypothermia, and acidosis that can be irreversible [4]. Historically, the early identification of patients with shock and or coagulopathy has been poor. An estimated 10–25% of all multiple injured trauma patients present with shock or acute traumatic coagulopathy upon arrival [5–7]. This shock and coagulopathy is not only related to acute surgical bleeding but also as a result of profound local release of inflammatory mediators due to tissue trauma [8]. In response to the recognition of massive and life-threatening bleeding leading to significant mortality in the trauma population, most patients deemed to be in shock related to surgical or coagulopathic bleeding receive blood transfusions during their early resuscitation phase. Over the last few decades, the transition to a balanced resuscitation of red blood cells (RBCs), fresh frozen plasma (FFP), and platelets emulating whole blood has improved mortality in traumatically injured patients [9–14]. Trauma patients requiring massive transfusion (MT) (>10 RBCs in 24 hours) have also been reported to have a mortality of up to 40–60% and deplete blood banks consuming over 70% of the blood products used for trauma purposes [15]. Meyer et al. showed that despite the American College of Surgeons Quality improvement guidelines recommendation of first cooler of blood product arrival by 15 minutes, odds of mortality increased by 5% with every passing minute waiting for blood products to arrive [16]. It is because of this that at least 85% of major US trauma centers have a massive transfusion protocol (MTP) established for the rapid mobilization of blood products from blood banks and early administration of products in a predefined ratio [17]. Despite everything we know about MTP and the survival benefit of early administration of blood products, there still does not exist a specific set of criteria for early (pre-hospital or ED) activation of the MTP.
Definition of Massive Transfusion
In order to understand the importance of MT with life-threatening hemorrhage (LTH) and its effect on outcomes, it’s necessary to settle on the definition of MT and of an outcome. This unfortunately is not straightforward.
Historically, the definition of MT has been the need of 10 or more units of RBCs over a 24-hour period [18–20]. This definition has been reported to have multiple flaws in appropriately describing the trauma patient with LTH and may either underestimate or overestimate the severity of bleeding and therefore the risk of death from hemorrhage. First, as stated by Cantle and Cotton [18], this definition fails to account for plasma and platelet transfusions that are often co-administered. Second, it may leave out those patients who were so severely injured and clearly in hemorrhagic shock that only received a few units of RBC prior to death [18]. The need for an MTP activation is primarily to direct the blood bank to rapidly deliver blood products to the bedside of a hemorrhaging patient. While this would be essential for a patient needing 10 units of RBC within the first 2 hours, it may not be necessary for 10 units required over a 24-hour period. Recent efforts have been made to improve on the definition by changing the timeframe in which 10 units or more of RBC were transfused to within 6 hours, but this still carries the same flaws as its predecessor and introduces survival bias [18]. In response to these potentially biased definitions of MT, numerous authors have developed their own definitions of MT to improve both the research and clinical definition which may have significant downstream implications with regard to predictive models of need for MT in LTH [18, 21].
The Prospective Observational Multicenter Major Trauma Transfusion (PROMMTT) study , which will be discussed in further detail in a later section, introduced the concept of Resuscitation Intensity (RI) [22]. This concept incorporates all types of fluid used in the initial resuscitation of trauma patients, to include each liter of crystalloid, each 0.5 L of colloid, and each unit of plasma, RBC, or apheresis platelets being defined as a single unit of resuscitation fluid. Rahbar et al. in 2013 showed that there was a threefold increase in mortality when a threshold of 3 units of resuscitation volume was administered over the initial 30 minutes. This translated to a 6-hour mortality of 14.4% vs. 4.5%, respectively, and a 24-hour relative increase in mortality of 76%. Given the RI is based on a fluid requirement per unit time, it accounts for the survival bias seen in the historical definition while also allowing for multiple methods of resuscitation not limited to colloid; however, it may be difficult to compare those patients who receive a predominantly crystalloid resuscitation to those who receive a colloid hemostatic one [18, 22].
The Critical Administration Threshold (CAT) , developed by Savage et al., is one of those models set out to redefine the concept of MT and remove the aforementioned survivor bias creating a more evidenced-based approach [23]. The CAT was defined as a threshold of 3 units of RBCs transfused over a 1-hour period. When compared to the traditional definition of MT as defined previously, CAT was more predictive of mortality (RR 3.6 vs. 1.8 for MT) [23]. Since CAT is a dynamic measure it can be followed throughout a patient’s resuscitation as opposed to one point in time. The number of episodes in which a patient met the CAT threshold within the first 24 hours was also predictive of the odds of death. The most significant improvement of CAT over MT was the ability of CAT to identify those patients who were equally as injured but who did not meet the MT threshold of transfusion in the first 24 hours. Of the 169 patients studied in the original article from 2012, 15 patients were CAT positive but did not meet the MT threshold. The CAT ability to recognize these patients improves on the survivor bias of the old MT definition [3]. This model was prospectively validated by the same authors at a separate institution with similar results. CAT was shown to be a more sensitive tool for identifying those critically injured patients that would benefit from early activation of a MTP when compared to the traditional definition of MT [23]. Although this new approach improves survivor bias, the currently existing models for prediction of the need for MT in LTH all define MT in the historical sense.
Evidence of Improved Outcomes
MTPs have evolved over the last few decades. The purpose of these protocols in general is to minimize any delay of the administration of blood products to a hemorrhaging patient by prescribing a predetermined “batch” of blood units to be empirically delivered from the blood bank to the bedside. An ideal protocol proactively sends batches of blood products to the bedside of a severely bleeding patient, as opposed to a reactive process that requires individual ordering of each blood product, which inevitably results in delays. Any such delay has been associated with an increase in mortality [16].
Efficient MTPs require a coordinated effort between first responders, emergency room, trauma team, and blood bank personnel. Various protocols exist and are site- and regional-specific. Riskin and colleagues in 2009 showed a drastic mortality reduction from 45% to 19% after implementing a MTP [24]. Dente and colleagues also found that there was a drastic reduction in early mortality from 57% to 19% when patients were transfused in a standard <2:1 ratio RBC:FFP than when transfused >3:1 [25]. At many institutions, MTPs are initiated by ED staff or trauma surgeons either prior to patient arrival from information reported by first responders or after primary survey in the trauma room immediately upon arrival. When an MTP is activated, blood banks immediately release uncross-matched RBCs, plasma, and platelets) often in a 1:1:1 unit ratio, typically 5–6 units of RBCs and plasma with 1 unit of apheresis platelets (equal to 5–6 units of whole blood derived platelets). Some centers provide different ratios of products and some even change the ratio delivered in each batch of blood products over time. After the initial MTP pack has been delivered, several blood tests (e.g., CBC, type and cross, comprehensive metabolic panel, standard coagulation assay, Ca2+, lactate, blood gas) are often obtained in order to assess the resuscitation. Some centers use viscoelastic testing (rapid-TEG or ROTEM) to guide the use of specific blood products or hemostatic adjuncts. Several adjuncts to the MTP may also be listed in a protocoled resuscitation effort, including an antifibrinolytic (e.g., tranexamic acid (TXA)), cryoprecipitate, or in rare cases recombinant factors of coagulation including VII and VII, and 4-factor prothrombin complex concentrate (PCC) to aid warfarin reversal. In Europe, recent studies reported on the diversity of the guidelines for damage control resuscitation across a number of institutions [26]. Although some similarities existed among the trauma centers, there are numerous differences in the use of triggers, labs to guide resuscitation, adjuncts, and indications for cessation of MTPs. This only demonstrates the evolving nature of DCR and the complex environment of standardized algorithms in the setting of varied sources of evidence [26].
The debate over the most beneficial ratio of blood products during a massive resuscitation of a patient in hemorrhagic shock is ongoing. In the two largest studies to date, the results are mixed. In the prospective observational PROMMTT study, ratios of plasma: RBC and platelet: RBC were compared as both continuous and categorical values. Results indicate that increased ratios as a continuous variable were independently associated with an reduced 6-hour mortality [27]. When both ratios were analyzed as categorical values, a ratio of >1:1 was associated with reduced 6-hour mortality. This mortality benefit was lost, however, after 24 hours [27]. In the Pragmatic, Randomized Optimal Platelet and Plasma Ratios (PROPPR) trial a mortality difference at either 24 hours or 30 days was not reported between patients receiving a 1:1:1 ratio vs. a 1:1:2 ratio of plasma, platelets, and RBCs, respectively [28]. The authors of the trial were able to conclude that the patients who did receive a 1:1:1 ratio had achieved hemostasis and experienced less death from exsanguination in the first 24 hours [28]. Borgman et al. evaluated patients who were predicted to need a MT and found that ratios of FFP:RBC >1:2 during the first 5 hours of admission improved survival, highlighting the importance of utilizing a predictive score to a trauma patient in order to identify who would benefit from an aggressive resuscitation strategy [29]. As noted earlier, ongoing monitoring of the resuscitation is essential and may ideally be guided by viscoelastic monitoring [30]. MTPs are generally stopped by the provider when it is determined that the patient is stabilized and there is no longer life-threatening bleeding; however, it is essential to continue to assess and monitor the patient for metabolic and coagulation derangements or potential new bleeding.
Predictive Models
Civilian database derived MT score/model summarization of characteristics
Score/model | ABC [6] | TASH [4] | PWH [29] | Vandromme [31] | Baker [24] | TBSS [8] |
---|---|---|---|---|---|---|
Design | Retrospective | Retrospective | Retrospective | Retrospective | Retrospective | Retrospective |
Number of patients | 596 | 4527 [4] 1517a [30] | 1.891b | 514 | 654 | 119 113a |
Setting | Civilian, single center | Civilian, multi center | Civilian, single center | Civilian, single center | Civilian, multi center | Civilian, single center |
Variable type | Non-weighted, dichotomous | Weighted | Weighted | Non-weighted, sum predictors | Non-weighted, sum risk factors | Weighted, sum risk factors |
Definition of MT | >10 units first 24 hours | >10 units ED to ICU | >10 units first 24 hours | >10 units first 24 hours | >10 units first 24 hours | |
SBP (mmHg) | ≤90 | <100 <120 | ≤90 | <110 | <90 | <90–≥110 (4 categories) |
Heart rate (bpm) | ≥120 | >120 | ≥120 | >105 | >120 | |
Hemoglobin (g/dl) | <7–<12 (5 categories) | ≤7–>10 (3 categories) | ≤11 | |||
Base excess (mmol/l) | <−10–<−2 (3 categories) | |||||
Base deficit (mmol/l) | >5 | |||||
Lactate (mmol/l) | ≥5 | 0–≥7.5 (4 ranges) | ||||
INR | >1.5 | |||||
FAST | Positive | Positive | Positive | cRegions positive | ||
Age (years) | ≥60 and ≤59 | |||||
Mechanism of injury | Penetrating | High-risk injuryc | ||||
GCS | ≤8 | <9 | ||||
Pelvic/extremity fracture | Positive | Positive (pelvic) | Type A–C | |||
Gender | Male | |||||
AUROC | 0.859 | 0.892 0.887a | 0.889 | 0.91 | 0.985 |
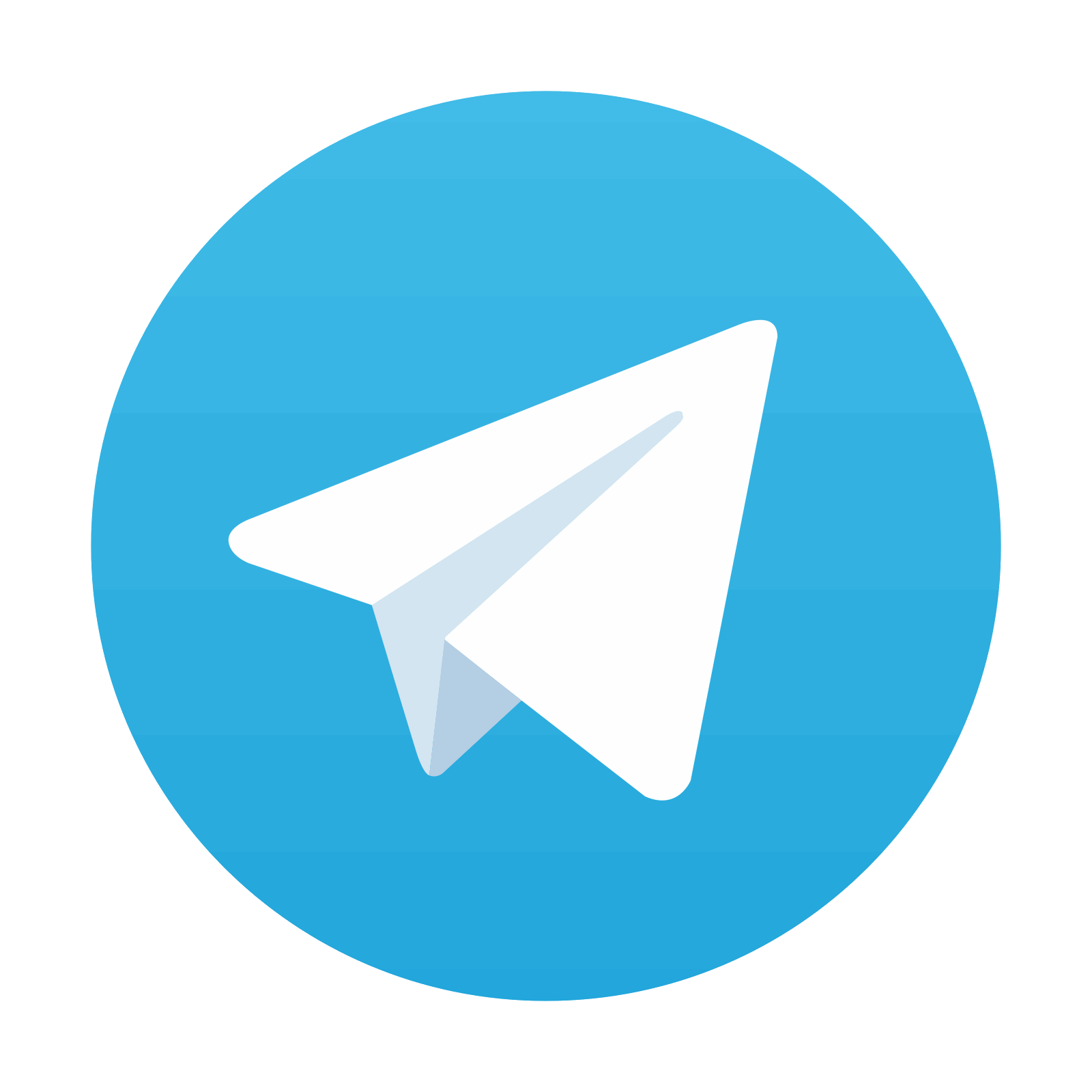
Stay updated, free articles. Join our Telegram channel

Full access? Get Clinical Tree
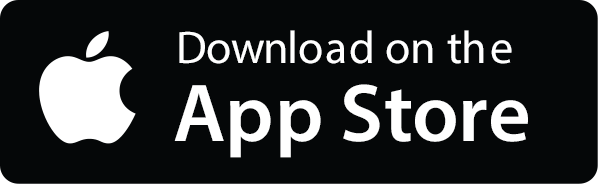
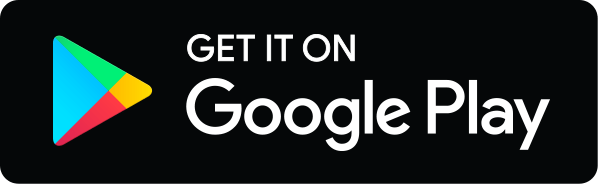