div class=”ChapterContextInformation”>
13. Colloids and Crystalloids
Keywords
Colloids Crystalloids Hetastarch Saline Lactated Ringer’sAbbreviations
- ARDS
Acute respiratory distress syndrome
- ATLS
Advanced Trauma Life Support
- DCR
Damage control resuscitation
- FDA
Federal Drug Administration
- HES
Hydroxyethyl starch
- HSD
6% dextran-70 in 7.5% sodium chloride
- HTS
Hypertonic saline
- kDa
Kilodaltons
- LR
Lactated Ringer’s
- mEq/L
Milli-equivalents per liter
- mmHg
Millimeters of mercury
- mOsm/L
Milli-osmoles per liter
- MW
Molecular weight
- NS
Normal saline
- RBC
Red blood cell
- TCA
Tricarboxylic acid
Introduction
Trauma is one of the leading causes of early mortality in the United States in persons under 50 years of age [1, 2]. Exsanguination is the leading cause of preventable death in this population. Exsanguination accounts for an even larger percentage of traumatic deaths in a battlefield setting than it does in the civilian setting [3, 4]. Trauma surgeons have been investigating methods to stem the degree of hemorrhage and to prevent as many of these deaths as possible. The concept of an abbreviated initial operation to arrest the lethal triad of coagulopathy, metabolic acidosis, and hypothermia was first described in 1983 by Stone and colleagues [5]. Since that time, there has been increasing promulgation of operative strategies that aim to temporize traumatic injuries and not necessarily provide definitive repair. This operative strategy has been termed “damage control surgery” and has been continuously refined based on the care of injured warfighters during the last two decades of conflict. Concurrently with the refinement of damage control surgery, there has been a parallel refinement in the fluid resuscitation known as damage control resuscitation (DCR) [6].
During World War I, battlefield surgeons recognized the importance and utility of whole blood in the treatment of acutely injured persons [7]. This practice continued through World War II and the Korean War, but it was noted that there was difficulty with storing whole blood that leads to significant wastage. Over time the use of whole blood was replaced by component therapy as it facilitated storage and minimized waste. As use of component therapy increased, crystalloid fluid use also increased for restoration of the circulatory volume. Unfortunately, with the large volume of crystalloid therapy there was a concomitant rise in the incidence of acute lung injury through an increase in the amount of extra-vascular lung water [8]. This recognition and efforts to avoid displacing established clots and avoiding coagulopathy lead to a decrease in the volume of crystalloids used, but crystalloids remained a mainstay of trauma resuscitation codified in the Advanced Trauma Life Support (ATLS) guidelines prior to the 10th edition. Blood products were used sparingly due to concerns with acute transfusion reactions and immune system depression.
Over the last several years there has been a resurgence in the utilization of blood products based largely upon the US military experience in Iraq and Afghanistan. Studies emerged that elucidated a survival benefit in patients who received a high plasma to red blood cell (RBC) transfusion ratio [9, 10] in both military combat and civilian trauma populations. This was subsequently studied in the US civilian trauma population in the PROPPR randomized controlled trial which demonstrated improved hemostasis and decreased death from exsanguination when a transfusion ratio of 1:1:1 plasma/platelets/RBCs was given compared to 1:1:2 [11]. This has resulted in recommendations for balanced resuscitation with blood products, including whole blood transfusions, and more judicious use of crystalloid fluids, during the damage control surgery/resuscitation phase of care.

An example of the sequela of intra-abdominal hypertension and bowel edema after a large volume crystalloid and blood product resuscitation following an abdominal gunshot wound. Note the persistent bowel dilation and fibrinous exudate

Chest x-ray with bilateral infiltrates in acute respiratory distress syndrome that developed following a large volume crystalloid resuscitation
Colloids
Common colloid fluids
Name (product name) | Osmolarity (mOsm/L) | Oncotic pressure (mmHg) | Estimated % volume expansion | Electrolyte composition | pH |
---|---|---|---|---|---|
Albumin, 5% | 330 | 20–29 | 80 | Na 100–145 mEq/L Cl 100–145 mEq/L | 6.7–7.3 |
Albumin, 25% | 330 | 100–120 | 200–400 | Na 100–145 mEq/L Cl 100–145 mEq/L | 6.7–7.3 |
6% hydroxyethyl starch (MW 670 kDa) in lactated Ringer’s (Hextend) | 273 | 25–30 | 100 | Na 143 mEq/L Cl 124 mEq/L Lactate 28 mEq/L Ca 5 mEq/L K 3 mEq/L Mg 0.9 mEq/L | 5.9 |
6% hydroxyethyl starch (MW 600 kDa) in normal saline (Hespan) | 308 | 25–30 | 100 | Na 154 mEq/L Cl 154 mEq/L | 5.9 |
6% Dextran-70 in hypertonic saline (7.5%) | 2566 | 75 | 120 | Na 1283 mEq/L Cl 1283 mEq/L | 5.7 |
The macromolecules are designed to be too large to traverse the glycocalyx and generally remain within the vasculature for a period of time. The actual plasma half-life of the colloid is dependent upon its molecular weight, route of metabolism, and degree of organ dysfunction. The concentration of the colloid in plasma increases the oncotic pressure within the vasculature relative to the interstitial and intracellular spaces and forces a net movement of water into the vascular space. The higher the oncotic pressure generated, the greater the amount of volume expansion.
Because the colloid draws in water from the body tissues, it results in a greater volume expansion than the actual volume of fluid infused. The decreased fluid requirement makes colloids attractive in theory due to mitigation of many of the complications associated with large volume infusions. By requiring less fluid volume per unit of volume expansion and long-term room temperature storage, colloids provide logistical benefits. Additionally, the decreased fluid volume weighs less, which is an important consideration in austere and prehospital environments where there are limitations in how much equipment and supplies can be carried in both military and civilian environments.
Albumin
Albumin is the most abundant protein in plasma and has an average molecular weight of 66 kDa. It is made by the liver and physiologically functions to aid in the transport of small molecules. It provides a pH buffer, maintains oncotic pressure, and aids in the binding and transport of divalent cations. Albumin’s role in maintaining oncotic pressure, and hence circulating blood volume, is the property that makes it useful in fluid resuscitation. Albumin is found in the extracellular fluid as well and can have increased translocation from the vasculature due to increased vascular permeability in the setting of shock. This translocation can contribute to the worsening of soft tissue edema and organ dysfunction.
Since albumin is derived from donated human blood, it does carry a theoretical risk of infectious disease transmission. The blood is screened at the time of donation for prior exposure to several different infectious diseases after which the albumin is purified by either cold fractionation with ethanol or chromatography and is then heat pasteurized. Although the fractionation and pasteurization processes lead to significant reductions in viral particles, there remains a theoretical risk for viral and prion disease transmission [15]. As a resuscitation fluid, albumin is relatively expensive due to the processing required. It is a stable solution that can be stored at room temperature. The purification process also removes the immunogenic elements found in whole blood, although allergic reactions, including anaphylaxis, have been described.
There have been many studies evaluating the use of albumin in humans, but there are a few that are relevant for patients with traumatic hemorrhagic shock and DCR. In 2004, Finfer and colleagues published the results of the SAFE study which evaluated the use of a 4% albumin solution against normal saline for fluid resuscitation of critically ill patients admitted to the ICU, in a multicenter, randomized, and double-blinded study. The trial was adequately powered to evaluate the primary endpoint of 28 day all-cause mortality [16, 17]. There was no difference in the primary or secondary endpoints. However, in a subsequent post-hoc subgroup analysis, the SAFE study investigators found that traumatically brain injured patients who had been in the albumin resuscitation arm had a higher mortality rate at 2 years as compared to those who received normal saline as their resuscitative fluid [18]. The investigators attributed this to increased intracranial pressure during the first post-injury week [19]. A subsequent Cochrane Review in 2013 pooled the data from 24 studies of all types of critically ill patients, yielding 9920 patients, to evaluate the use of albumin for resuscitation. The pooled relative risk was reported to be 1.01 (95% CI 0.93–1.10), thus failing to find a mortality difference when albumin was compared to a crystalloid solution [20]. Given the lack of benefit, as compared to crystalloid solutions, and potential risk of harm in traumatic brain injured patients, albumin is not a common first line product for fluid resuscitation in patients with life-threatening bleeding requiring DCR.
Hydroxyethyl Starch
Hydroxyethyl starches (HES) are highly branched glucose-based polymers that are derived from amylopectin. Hydroxyethyl groups are synthetically added to the hydroxyl groups using ethylene oxide. The addition of multiple hydroxyethyl groups stabilizes the starch to degradation and metabolism. The amylopectins can come from a variety of plant sources and when purified have a wide range of molecular weights due to variations in the polymer length. HES is cleared via renal excretion, and its half-life in plasma is dependent upon renal function and tissue deposition.
Animal and clinical studies have been difficult to interpret due to the different sizes of starches being studied. Given the significant structural differences between the various HES products, findings with one product may not be applicable to all the others. Two commercially available formulations, Hextend and Hespan, both use an HES mixture with an average molecular weight of 600–670 kDa and are the most commonly studied for the use in trauma resuscitation. There is a newer variant of HES known as tetrastarch that has a mean molecular weight of 130 kDa. Tetrastarch was formulated with the intent of having a shorter half-life than other HES polymers. This product has not been evaluated for use in the trauma resuscitation setting, but it has been associated with negative outcomes in septic ICU patients but may have less risk for anaphylaxis [12, 21, 22].
As with all colloids, HES produces a volume expansion via an increase in vascular oncotic pressure. Hextend and Hespan’s ability to expand the circulatory volume is similar to that of 5% albumin [12]. Both of these solutions contain 6% HES since studies have suggested that HES solutions with concentrations greater than 10% high molecular weight HES have increased rates of death, acute kidney injury, and need for renal replacement therapy [23]. These risks have led to FDA black box warnings on all forms of HES [24, 25]. For many clinicians, HES fluids have long raised concerns about anaphylaxis, coagulopathy, and uncontrolled massive hemorrhage [26], although these concerns have been largely based upon case reports. Olgivie and co-workers attempted to examine the safety, efficacy, and effect on coagulation of Hextend with a non-randomized, non-blinded study in 2008. In this study, they found that the patients who received Hextend had a lower mortality rate and no evidence of coagulopathy or transfusion requirement [26, 27]. The design of the study limits the ability to interpret if there is a survival benefit, but the results do show that Hextend does not affect PT or PTT in the 500–1500 ml volumes studied. Thrombelastography was not used in this study, so we do not know if other aspects of hemostasis were affected by Hextend, which has been associated with decreased factor VIII and von Willebrand factor when used in higher doses [12, 28]. Given the lack of clear clinical benefit and potential risk of harm with HES, it is difficult to recommend its use outside of an austere or logistically challenged environment.
Dextran
Dextrans are biosynthetic, highly branched, polysaccharides. Similar to HES, dextrans can have a wide range of molecular weights, but are purified to specific molecular weights. In the DCR setting, dextran-70 (70 kDa average molecular weight) is the most commonly used and studied. As with albumin and HES, dextrans can provide a significant increase in the intravascular volume relative to the infused volume and are primarily excreted in the urine. Dextrans as a class have been associated with anaphylaxis, coagulopathy, and renal failure by precipitation in the renal tubules. They also impair blood cross-matching by obscuring the RBC surfaces.
There have not been many studies to evaluate the use of dextran in the trauma setting due to concerns about coagulopathy. In 1991, Mattox and co-workers evaluated the use of 6% dextran-70 in 7.5% sodium chloride (HSD) in a multicenter trial. This study compared the use of 250 ml of the dextran solution vs 250 ml of a crystalloid solution prior to standard resuscitation. In this study, they did not note coagulopathy with the doses and volumes used. There was no clear mortality benefit for the study cohort overall, but the subgroup that did require an operation demonstrated a survival benefit. Also importantly, the standard therapy appeared to be associated with a higher incidence of acute respiratory distress syndrome (ARDS), renal failure, and coagulopathy [29]. This lead to a blinded multicenter randomized study of prehospital use of 7.5% hypertonic saline, normal saline, and HSD (ROC study). This study was stopped early after a planned safety review for futility and patient safety. Patients in the HTS and HTS/dextran arms who did not receive blood transfusions in the first 24 hours after injury were found to have a higher mortality rate [30]. It was not clear to the study’s authors why there was this effect, but they postulated that shock was masked by HTS resuscitation resulting in delayed transfusions. Early mortality was higher in the HTS groups, but later mortality was lower leading to no overall difference. Based on these studies, dextran is not recommended for DCR.
Given the lack of a robust benefit with the use of colloids in the setting of resuscitations along with their higher cost, colloid solutions are not recommended as the first line fluid for use in DCR. Currently in the US Military Tactical Combat Casualty Care (TCCC) Guidelines, Hextend remains a part of the treatment recommendations, but only if blood products are not available, and remains preferred over crystalloids due primarily to prehospital logistics [31].
Crystalloids
Common crystalloid fluids
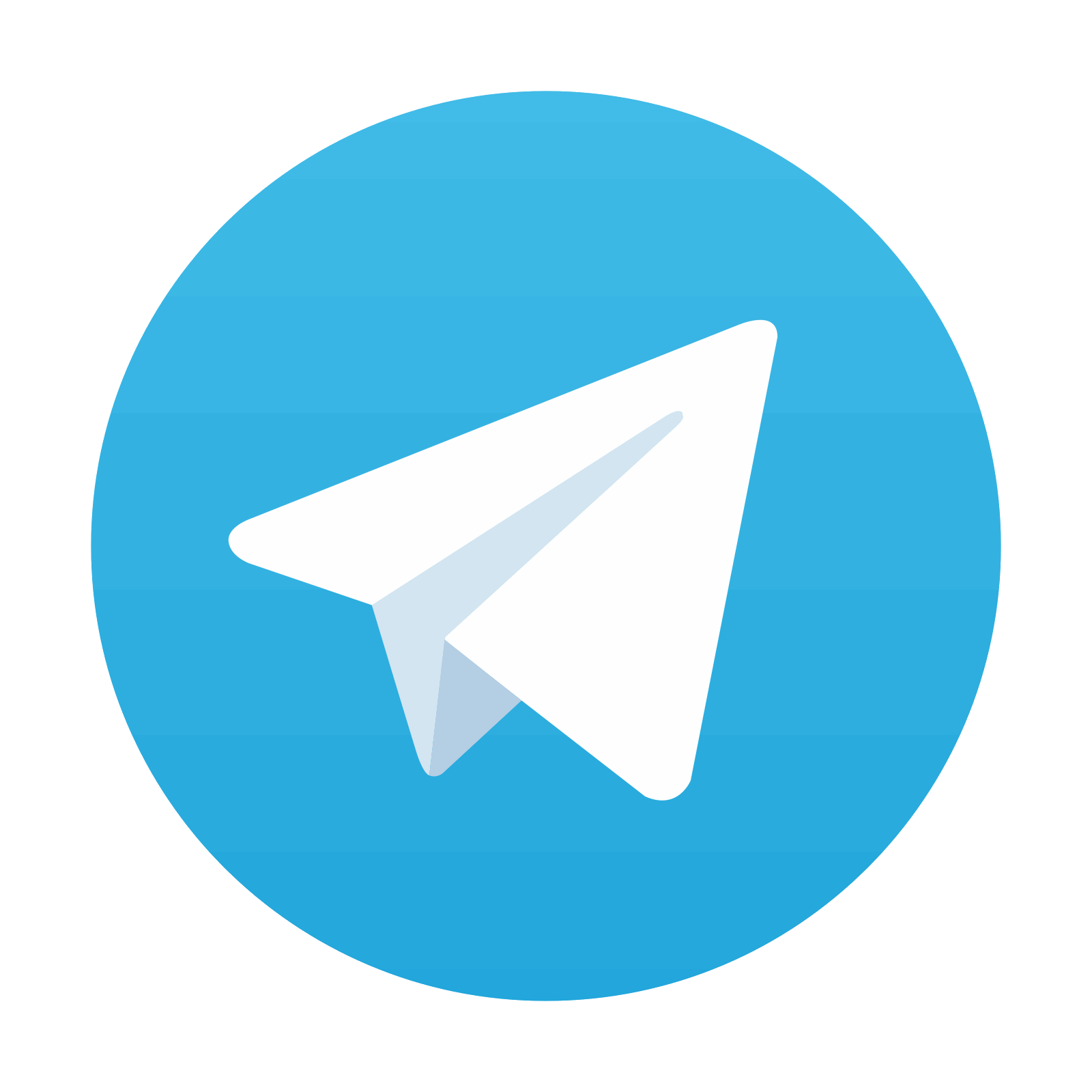
Stay updated, free articles. Join our Telegram channel

Full access? Get Clinical Tree
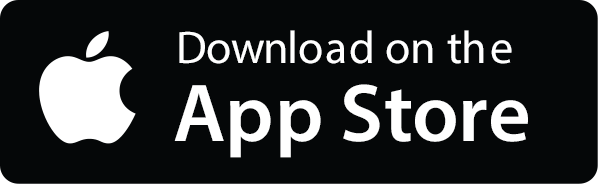
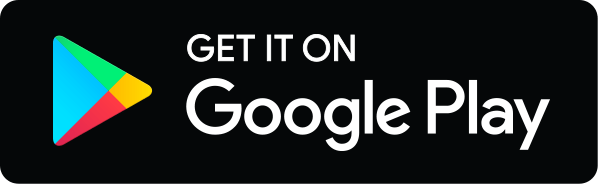
