!DOCTYPE html PUBLIC “-//W3C//DTD XHTML 1.1//EN” “http://www.w3.org/TR/xhtml11/DTD/xhtml11.dtd”>
Neuromodulation of Abdominal and Pelvic Pain
INTRODUCTION
Over the last 50 years of pain research, we have been obsessed with peripheral nervous system mechanisms of nociception. From the chemical mediators that stimulate afferent nerve endings, to the glycinergic control of central sensitization in the spinal dorsal horn, our view of nociception has largely ignored the role of supraspinal processing. This preoccupation is surprising, given that the field has matured with Melzack and Wall’s revolutionary idea that pain perception requires cortical processing [31]. We have, in essence, decapitated pain.
Chronic abdominal and pelvic pain consists of a family of visceral pain conditions initiated by organ pathology and chronically maintained by aberrant peripheral and central processes. The characteristics that define visceral pain emphasize the role of the brain in nociceptive processing. Visceral pain consists of diffusely localized pain that is inherently linked with negative emotion and autonomic reactivity. The implications of this coexistence are that the nociceptive processes underlying the chronification of visceral pain must be accompanied by sustained negative emotion, such as anxiety and/or depression, as well as ongoing autonomic arousal, which may parallel the elevated arousal that characterizes chronic stress, both of which recruit extensive cortical processing [37]. Furthermore, the co-occurrence of visceral pain and negative emotion may enhance the formation of pain-related memories. It has been hypothesized that memory formation relies on arousal mechanisms to modulate memory strength [10,30] such that memories encoded during emotion-laden situations will be well remembered for future adaptive purposes. In turn, abdominal and pelvic pain may further dysregulate emotional circuitry, as it disrupts some of the most inherently rewarding physiological processes, including eating, sex, urination, and defecation, which are required for the survival of the organism.
This chapter explores how the brain shapes the modulation of abdominal and pelvic pain across different time scales, through changes in brain function and structure. It is argued that limbic, rather than classic nociceptive circuitry is the primary site of central reorganization in visceral pain.
BASIC ASPECTS
Chronic visceral pain, like any learned experience, is subject to the rules of memory formation and maintenance. As a result, the impact of visceral pain is etched into the synaptic patterns that have relayed nociceptive and emotional information with the repeated exposure to painful visceral stimuli. The activity of these individual synapses is not accessible via neuroimaging due to poor spatial resolution, given that a single voxel represents roughly 100,000 neurons [28]. However, in understanding the rules by which these neurons function and interact, we can extrapolate these principles to large populations of neurons that are accessible via imaging.
The Hebbian axiom, “Neurons that fire together wire together,” is useful in understanding how brain function relates to anatomy. The relay of neural information is physically constrained by the properties of individual neurons (such as variations in axonal and dendritic morphology), their interactions with resident glia, the number of synaptic connections they make with neighboring neurons, and their connections with distant neuronal populations. These anatomical constraints are not fixed; rather, these properties can dynamically adapt to reflect new learning, rendering brain function and anatomy interdependent.
Brain function and structure convey a rich amount of information about the progression from acute to chronic pain. A range of imaging modalities can address unique and complementary clinical questions as to how the brain adapts to pain in the short- and long-term. Short-term changes are reflected in brain functional properties, whereas longer-term changes (as well as predispositions) associated with chronic pain are evident in gray and white matter properties, as well as through cross-sectional and longitudinal assessments of brain function related to clinical pain.
Functional activity associated with acute and chronic pain is measured using functional magnetic resonance imaging (fMRI), which infers neural activation from local variations in oxygenated blood flow [27]. Regional changes in the blood oxygen level dependent (BOLD) signal that correlate with painful stimulation or its perception have yielded consistent patterns of activity in the anterior cingulate cortex (ACC), somatosensory cortices (S1 and S2), insula (INS), thalamus (Th), and prefrontal cortex (PFC), which are collectively referred to as the acute pain “matrix” [46]. Functional activation of these regions has repeatedly correlated with clinically important aspects of pain perception (e.g., pain intensity, unpleasantness, duration, etc), potentially providing an “objective” measure of pain [49]. However, critics have questioned the sensitivity and specificity of this acute pain signature because it is also elicited by non-painful stimulation, as well as by other sensory modalities [21]. Growing evidence also suggests that the neural representation of chronic pain deviates from this acute pain matrix in condition-specific patterns [2]. The literature remains divided as to how abnormal cortical processing of nociceptive information may manifest in the chronic pain state.
A complementary view of brain functional activity is to view the brain as a conglomerate of efficiently communicating neural networks. When activity in two or more network elements (e.g., voxels, regions) consistently correlate across time they are said to be functionally connected. Network connectivity appears to reflect transient states of mind, including intrinsic and task-related states [19], as well as developmental processes and the presence of chronic disease [1,39]. This suggests that networks can manifest short- and long-term neuroplasticity by (a) adapting to brain state changes in order to optimize the representation of information, as well as (b) evolve over time based on reinforced functional connections, which may in turn promote structural changes [17]. As a result, the rich information conferred by neural networks captures activity and communication patterns that are inaccessible via traditional general linear modeling analysis of fMRI data.
Neuroimaging can differentiate broad anatomical features of the brain, such as gray (neuronal) matter and white (axonal) matter properties. Gray matter is visualized with T1-weighted anatomical images, which differentiate types of brain tissue based on their magnetic properties. Although gray matter density is often assumed to reflect regional concentrations of neurons, it may also reflect changes in vasculature, non-neuronal (glial) growth, neuron morphology (e.g., dendritic arborization and spine growth), neurodegeneration, and less often neurogenesis. The regional distribution of gray matter can capture anatomical changes that take weeks to months to occur. Regional changes in gray matter are associated with the central processes that underlie the maintenance and remission of chronic pain [4,41]. Indeed, brain morphology continues to adapt to the presence of chronic pain over the course of years [5].
White matter microstructure is deduced using diffusion tensor imaging (DTI), which measures directional water flow within the brain. Whereas the water flow within the physical confines of a neuron is uniform in all directions, the water flow along the length of myelinated axons is directionally dependent, or anisotropic. Chronic pain is typically accompanied by subtle changes in white matter integrity, and these axonal properties may change over the course of days to weeks, indicating that it is a longer-term index of brain reorganization. Alternatively, certain white matter properties may also reflect a predisposition for chronic pain development, as suggested by the first longitudinal neuroimaging study of the transition to chronic back pain [29].
DESCRIBING THE SUBJECT
Genital Pain
Vulvar pain syndrome/Vulvodynia, is the most common type of female genital pain, affecting approximately 8% of premenopausal women [36]. In healthy women, vulvar touch and pain perception evoke equivalent patterns of brain activity that are classically observed with acute pain [16]. Similarly, women with provoked vulvodynia exhibit a similar pattern of activity during experimentally-induced vulvar mechanical stimulation. Higher levels of INS and PFC activation were cited as evidence of elevated pain processing in patients, yet equivalent levels of subjective pain in healthy women were not assessed, nor was an innocuous vulvar stimulation control included in the design [35]. This activation pattern was confirmed in a recent study, however, women presenting with different subtypes of vulvodynia did not show elevated regional activity when compared to clinical (fibromyalgia) and healthy controls [20]. Despite this equivocal evidence of altered central nociceptive processing, women with provoked vulvodynia exhibit increased subcortical gray matter density in the basal ganglia and parahippocampal gyrus/hippocampus [38]. These structural changes may reflect longer-term shifts in information processing related to chronic vulvar pain. Indeed, these gray matter properties correlated with multiple clinical indices of vulvar hypersensitivity, as well as with self-reports of pain catastrophizing.
These studies indicate that provoked vulvar pain in women with vulvar pain/vulvodynia recruits the same pattern of nociceptive processing observed during acute pain perception in healthy individuals. On first glance, this finding challenges the hypothesis that abnormal vulvar pain processing plays a role in vulvar pain/vulvodynia. However, multiple methodological and sample biases, noted above, may confound these results. It is also possible that these studies may have failed to elicit clinically-relevant vulvar pain (due to vaginal penetration, for instance) and therefore have limited relevance to the actual mechanisms underlying the condition. The presence of subcortical anatomical abnormalities that correlate with clinical pain parameters, in particular, suggests the presence of either preexisting vulnerabilities that make these women more likely to develop vulvar pain/vulvodynia, or they are indicative of subcortical reorganization related to the maintenance of chronic vulvar pain.
Menstrual Pain
One of the most common forms of female pelvic pain is menstrual pain, or dysmenorrhea, which causes severe pain in 2–29% of premenopausal women [23,48]. During menstruation, pain-related brain activity does not differ between women with and without dysmenorrhea. Specifically, painful thermal lower abdominal and arm stimulation evoked similar activation patterns in the secondary somatosensory cortex (SII), premotor cortex (PMC), INS, ACC, posterior cingulate cortex (PCC), orbitofrontal cortex (OFC), and subcortical regions (e.g., putamen, thalamus (Th), caudate, and brainstem) [48].
Women with dysmenorrhea exhibit a range of cortical and subcortical anatomical changes that correlate with clinical parameters. A well-designed study of this population discovered nuanced gray matter changes during menstrual and peri-ovulatory phases [47
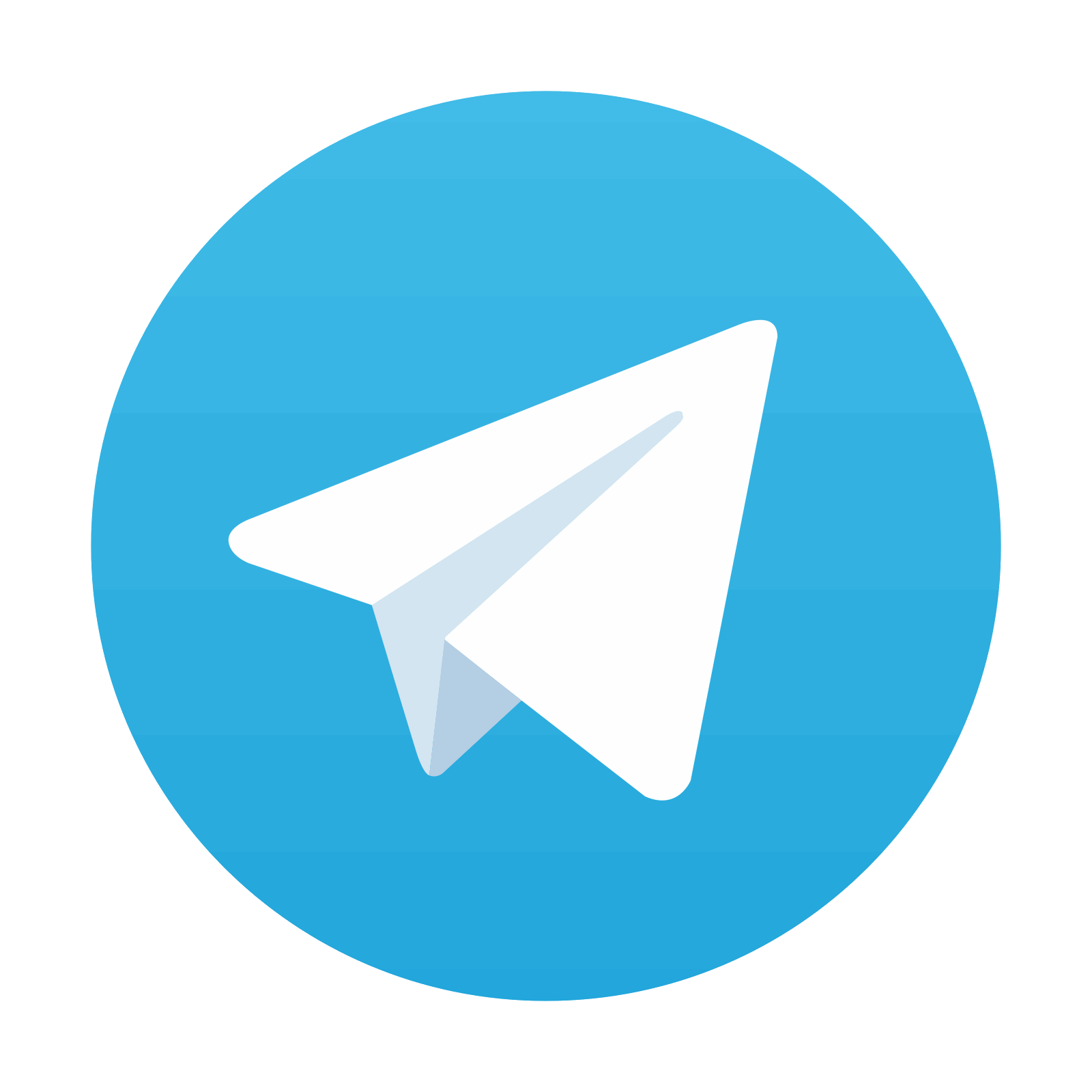
Stay updated, free articles. Join our Telegram channel

Full access? Get Clinical Tree
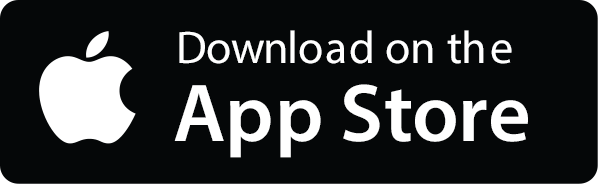
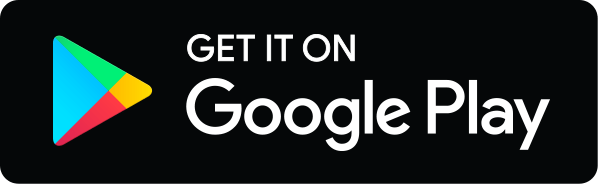